User login
The American Journal of Orthopedics is an Index Medicus publication that is valued by orthopedic surgeons for its peer-reviewed, practice-oriented clinical information. Most articles are written by specialists at leading teaching institutions and help incorporate the latest technology into everyday practice.
Unicondylar Knee Arthroplasty in the U.S. Patient Population: Prevalence and Epidemiology
ABSTRACT
Publications on the prevalence of unicompartmental knee arthroplasty in the United States using a single database may have underestimated the “true” number of cases performed, given that several unicondylar knee arthroplasty (UKA) patients are <65 years and have private insurance. The prevalence of UKA in elderly (≥65 years) and younger (<65 years) populations was evaluated using the 2002 to 2011 5% sample of the Medicare data (Part B) and the 2004 to June 2012 MarketScan Commercial and Medicare Supplemental databases, respectively. The prevalence of UKA was stratified by age, gender, census region, Charlson comorbidity index, Medicare buy-in status, and diagnosis. The annual rate of change in the UKA rate was examined using Poisson regression to evaluate temporal changes considering year as a covariate.
A total of 5235 and 23,310 UKA procedures were identified from the 5% Medicare and MarketScan databases, respectively. The rates of UKA generally increased until 2008, after which there was a decline. In both cohorts, gender and year of operation were found to be significantly associated with UKA rate. Analysis of data obtained over the past few years revealed that males 55 to 64 years, 65 to 69 years, and 70 to 74 years were the only age-gender groups whose UKA rates appeared to be trending upward.
From 2002 to 2011, the rate of UKAs performed in the United States has increased, and a significant proportion of the surgeries were performed in younger (<65 years) patients.
Continue to: Unicondylar knee arthroplasty...
Unicondylar knee arthroplasty (UKA) is an effective surgical treatment for symptomatic degenerative joint disease of a single compartment of the knee, providing improved functional outcomes compared with total knee arthroplasty (TKA).1-3 It has been estimated that the proportion of patients undergoing TKA, who meet the criteria for UKA, varies between 21% and 47%.4,5 However, it has been variably estimated that the usage of UKA ranges from 0% to 50% (mean, 8%) of all primary knee arthroplasties.5-8 It is believed that this discrepancy between the percentage of patients who meet indications for the surgery and those who receive it is associated with various factors, including surgeon training and experiences, diverse indications, economic factors, as well as acknowledgment of the reportedly higher revision rates of UKA than those of TKA in national joint registries.7,9-11
According to their classic article, Kozinn and Scott12 outlined the indications for UKA that, in their experience, led to the most successful outcomes, including age >60 years, weight <82 kg, low physical demand, localized arthritis with no full-thickness chondromalacia elsewhere in the joint, intact anterior cruciate ligament, minimal deformity, and flexion >90°. More recently, indications have been expanded to include younger and more active patients, higher body mass index, and some patterns of patellofemoral chondromalacia, with an increasing number of publications reporting successful clinical outcomes in these cohorts as well.13-17 Taken together, it is clear that the “classic” strict indications for UKA can be safely expanded, which have and will result in an increased number of these procedures being performed above and beyond that which might be predicted based on demographic trends alone.
A growing body of literature has been published on the prevalence and projections of orthopedic procedures in the United States.18-20 Several studies have focused their analysis on 1 of several large administrative databases, including the Nationwide Inpatient Sample, the 5% Medicare Part B database, and the National Hospital Discharge Survey.18,20-23 A concern with limiting an analysis of the prevalence of unicompartmental knee arthroplasty to these particular databases is that it may underestimate the “true” number of cases performed in the United States, given that several UKA patients are <65 years and have private insurance, and therefore, would not be captured statistically by a database that collects data on patients ≥65 years.
The purpose of this study was to quantify the current prevalence and epidemiology of UKA in the U.S. patient population. Our hypothesis was that the number of procedures and the procedural rate of UKA are increasing over time. Furthermore, this increase may be attributed to an increase in select age- or gender-based segments of the population. To test this hypothesis, we analyzed 2 separate large claims databases to capture patients over a spectrum of age and inclusive of both private and public payers, including the 5% Medicare Part B database (2002–2011) for patients ≥65 years and the MarketScan database (2004 to June 2011) for patients <65 years. Understanding the accurate trends in the use of UKA on a national scale is important for legislative bodies, healthcare administrators, and physicians.
MATERIALS AND METHODS
The 2002 to 2011 5% sample of the Medicare data (Part B) and the 2004 to June 2012 MarketScan Commercial and Medicare Supplemental databases were used to evaluate the prevalence of UKA in elderly (≥65 years) and younger (<65 years) populations, respectively. The UKA procedures were identified using the CPT code 27446.
The prevalence of UKA was stratified by age, gender, census region, Charlson Comorbidity Index, Medicare buy-in status, and diagnosis. The buy-in status is a proxy for the socioeconomic status as it reflects the state subsidizing the health insurance premium for the beneficiary. The Charlson Comorbidity Index is a composite score that has been used to assess the comorbidity level of a patient by taking into account the number and the severity of comorbid conditions.24 For the elderly population, the rate of UKA was subsequently evaluated based on the number of beneficiaries for that particular age-gender group and year in both databases. Poisson regression was used to evaluate the annual rate of change in the UKA rate for assessing temporal changes considering year as a covariate. Age and gender, as well as 2-way interaction terms for age, gender, and year, were also considered as covariates.
Continue to: RESULTS...
RESULTS
For the time periods analyzed, a total of 5235 and 23,310 UKA procedures were identified from the 5% Medicare and MarketScan databases, respectively. A peak in the prevalence appeared around 2008 for the elderly population and in 2009 for the younger population (Figure 1). When normalized by the size of the population segment, the rate of UKA was found to be approximately 5 times greater in the elderly population, increasing from 369 in 2002 to 639 in 2008, but plateauing to 561 in 2011. Extrapolating to the 100% Medicare population, these numbers increased to 7380, 12,780, and 11,220, respectively. Temporal changes in the UKA rate were significant, increasing from 24.5 UKAs per 100,000 persons in 2002 to 43.1 UKAs in 2008, followed by a decline to 36.5 in 2011 (P < .0001) (Figure 2). The rates of UKA generally increased from 2002 to 2008 for both males and females in the Medicare cohort; however, the rates of UKA in female patients continuously declined from 2008 onward, whereas the UKA rates in male patients decreased in 2009, followed by an increase in 2010 and 2011 (Figure 2). For the younger population, there was a slight increase in the rate of UKA from 2004 to approximately 2009, after which the rates for both males and females remained relatively steady. When put in the context of the prevalence of TKA, the prevalence of UKA fluctuated during the same time period. In the Medicare population, the prevalence of UKA ranged from 4.3% (2005) to 5.9% (2008) of the TKA prevalence between 2002 and 2011. In the younger MarketScan population, the prevalence of UKA ranged from 6.7% (2005) to 8.9% (2008) between 2004 and June 2012.
The UKA rate differed significantly according to gender (P = .0209), with higher rates for males. Although there were no age-related differences (P = .3723), age–gender interactions were found to be significant (P < .0001). For males, the largest rate of UKA in the most recent year of data was observed in the 70- to 74-year-old group, followed by the 75- to 79- and the 65- to 69-year-old groups (Figure 3). For females, those in the 65- to 69- and the 70- to 74-year-old groups had the highest rate of UKA. In the younger cohort, there were increases in the UKA rates since 2004. These rates appeared to be relatively stable from the 2008 or 2009 period onward, except for females 55–64 years, which demonstrated a steady decline since 2008. Analysis of data obtained over the past few years showed that males 55–64, 65–69, and 70–74 years were the only age–gender groups whose UKA rates appeared to be trending upward.
The vast majority of elderly UKA patients were white (95.5%), and when stratified by census region, the highest proportion of UKA procedures was observed in the South and the Midwest (Figure 4). Furthermore, among patients <65 years, 64.2% had a Charlson score of 0 compared to 40.8% in the elderly group (Figure 5). For the Medicare population, based on their receipt of state subsidies for their insurance premiums, 5.1% of patients were of lower socioeconomic status. Osteoarthritis was diagnosed in 99.4% and 97.3% of the MarketScan and Medicare cohorts, respectively.
In the Medicare cohort, gender (P = .0209) and year of operation (P < .0001) were found to be significantly associated with the rate of UKA, along with age-gender (P < .0001) and gender-year (P = .0202) interaction terms. In the MarketScan cohort, age (P = .0173), gender (P = .0017), and year of operation (P = .0002) were found to be significantly associated with UKA rate. Two-way interactions between age-gender (P = .0018), age–year (P = .0207), and gender-year (P = .0017) were also found to be statistically significant factors.
Continue to: DISCUSSION...
DISCUSSION
The results of our study indicate that between 2002 and 2011, a steadily increasing number of UKA procedures was performed in the United States, and a significant proportion of the surgeries was performed on patients <65 years. Without the MarketScan database data, we would have missed more than 23,000 UKA cases performed during this 10-year time period. This finding validates our research methodology that incorporated data on privately insured younger (<65 years) patients, which is something that has not been done when examining the epidemiology of UKA.
To our knowledge, there are only 2 other publications attempting to quantify the incidence of UKA procedures performed in the United States. Bolognesi and colleagues23 used the Medicare 5% sample to assess trends in the use of knee arthroplasty from 2000 to 2009. The authors reported that a total of 68,603 patients underwent unilateral total knee arthroplasty (n = 65,505) or unicompartmental knee arthroplasty (n = 3098) over this 10-year time period. Given that there is substantial overlap of our time periods, it is not surprising that our Medicare numbers are similar (3098 vs 5235). In their study, the use of TKA increased 1.7-fold, whereas the use of UKA increased 6.2-fold23. In our analysis of the Medicare (2011 vs 2002) and MarketScan (2011 vs 2004) databases, there was a 1.3-fold and a 3.4-fold increase in the number of TKAs performed. Concomitantly, the use of UKA increased 1.5-fold and 2.8-fold, respectively, in these databases over the same time periods. The reason for the slight discrepancy in the numbers may be attributable to the peak occurring in 2008. The other publication on the subject by Riddle and colleagues8 focused on the time period 1998 to 2005 and used implant manufacturer’s sales data cross-referenced to a database of 44 hospitals to derive their national estimates. Using their unique methodology, the authors calculated an incidence of UKA, ranging from 6570 implants in 1998 to 44,990 in 2005. They reported that UKA use during the study period increased by 3 times the rate of TKA in the United States, with an average yearly percentage increase in the number of UKA procedures of 32.5% compared to 9.4% for TKA procedures. It is difficult to account for the discrepancy in the number of UKAs performed reported between our current study and that of Riddle and colleagues;8 however, the fact that the authors used implant manufacturer’s individual sales numbers may indicate that a portion of UKA patients was not captured in either the Medicare 5% or the MarketScan database. Nonetheless, in our analysis, the annual increase in the number of UKA procedures performed during the time periods studied averaged 5.8% in the older population and 25.4% in the younger population compared to the increase in the number of TKA procedures, which averaged 3.6% and 33.9% in the older and younger populations, respectively. In addition, in our study, the percentage of UKAs performed relative to the number of TKAs during the time intervals studied varied from a low of 4.3% to a high of 5.9% in the older population and from a low of 6.7% to a high of 8.9% in the younger population.
During the 10-year period of this study, a general upward trend appeared in the total number of unicompartmental knee arthroplasties performed in both the Medicare and the MarketScan databases. The rate at which the procedure was performed increased in the Medicare population from 24.5 to 36.5 (per 100,000 persons) over a 10-year time period and in the MarketScan cohort from 5.9 to 7.4 (per 100,000 persons) over an 8.5-year time period. This indicates both a larger absolute and a relative rate increase in UKA procedures in the elderly population. Around 2008 and 2009, the data showed a slight dip in the rate of UKA in the Medicare population and a plateau in the rate in the MarketScan database. Although this may be a spurious finding in the data that would be smoothed out with a longer time period investigated, it is interesting that this finding coincided with a national economic downturn. Although it might be expected that macroeconomics may affect the utilization of elective surgery such as total joint replacement, Kurtz and colleagues25 investigated this particular question and found that neither the economic downturns of 2001 or those of 2008 and 2009 had a significant impact on the incidence of total joint replacement surgeries.
Incorporation of the MarketScan database data indicated that a significant proportion of patients undergoing UKA were <65 years and that there was a slight but increasing rate of procedures performed on this age cohort over the past decade. A similar finding has been reported in the Finnish Arthroplasty Registry. Leskinen and colleagues26 reported that the incidence of UKAs among individuals 30 to 59 years increased from 0.2 (per 100,000 persons) to 10 (per 100,000 persons) from 1980 to 2006 and that most of the increase occurred among patients 50 to 59 years. The fact that younger age is no longer observed as a relative contraindication to this procedure is supported by several clinical investigations. Cartier and colleagues27 reported 93% survival at 10 years in patients with a mean age of 65 years, but included patients as young as 28 years, claiming that the results for younger patients were no worse than those for older patients in the series. Pandit and colleagues17 compared the results of 245 young patients (<60 years) to those of 755 older patients (>60 years) and found a survival rate of 97% at 10 years, with no significant difference in mean functional outcomes, failure rate, or survival between the groups at >5 years of follow-up. Given that patients <65 years now account for approximately half of the TKAs performed each year, with the greatest increase in volume among patients between 45 and 54, it is clear that investigations on the epidemiology of UKA must take into account this increasingly relevant younger patient cohort.28
Continue to: Our data indicate...
Our data indicate that only approximately 5% of UKA patients were non-white and another 5% were from lower socioeconomic status. These findings have been observed in multiple other studies looking at the epidemiology of total joint replacement in the United States.29 Bolognesi and colleagues23 reported that although “non-white race” patients made up 12% of the general Medicare sample they were analyzing, these patients accounted for only 5% and 3% of the total knee arthroplasty and unicompartmental knee arthroplasty populations, respectively. Although it is beyond the scope of this paper to delve into the reasons for this discrepancy, it may be related to differences in access to care, healthcare literacy, and trust of patients in the healthcare system.30,31
Our study, like all those based on administrative claims, has several notable inherent limitations. Coding inaccuracies as well as the potential for systematic bias (eg, underreporting) may affect the accuracy of our results. Although the MarketScan Commercial Research Database (Truven Health Analytics) includes nationally representative information for >180 million patients covered with private insurance, it is possible that we have missed some patients who underwent UKA during the time period investigated. However, we feel that the number missed is probably small and does not affect our conclusions in any meaningful manner.
CONCLUSION
This novel analysis of 2 separate administrative claims databases, which more accurately captures all patients undergoing UKA, indicates that there has been a steady increase in the rate of the procedure over the past decade and that a significant proportion of the surgeries were performed in younger (<65 years) patients. Understanding the accurate trends in the use of UKA on a national scale is important for legislative bodies, healthcare administrators, as well as physicians. Furthermore, given the increasing rates of UKA in patients <65 years old, and the increased burden on implants for withstanding increased activities and repetitive loads, it remains imperative to strive to optimize materials, implant designs, and surgical techniques to enhance implant durability.
- Hopper GP, Leach WJ. Participation in sporting activities following knee replacement: total versus unicompartmental. Knee Surg Sports Traumatol Arthrosc. 2008;16(10):973-979. doi: 10.1007/s00167-008-0596-9.
- Lygre SH, Espehaug B, Havelin LI, Furnes O, Vollset SE. Pain and function in patients after primary unicompartmental and total knee arthroplasty. J Bone Joint Surg, (Am). 2010;92(18):2890-2897. doi: 10.2106/JBJS.I.00917.
- Liddle AD, Pandit H, Judge A, Murray DW. Patient-reported outcomes after total and unicompartmental knee arthroplasty: a study of 14,076 matched patients from the National Joint Registry for England and Wales. Bone Joint J. 2015;97-B(6):793-801. doi: 10.1302/0301-620X.97B6.35155.
- Arno S, Maffei D, Walker PS, Schwarzkopf R, Desai P, Steiner GC. Retrospective analysis of total knee arthroplasty cases for visual, histological, and clinical eligibility of unicompartmental knee arthroplasties. J Arthroplast. 2011;26(8):1396-1403. doi: 10.1016/j.arth.2010.12.023.
- Willis-Owen CA, Brust K, Alsop H, Miraldo M, Cobb JP. Unicondylar knee arthroplasty in the UK National Health Service: an analysis of candidacy, outcome and cost efficacy. Knee. 2009;16(6):473-478. doi: 10.1016/j.knee.2009.04.006.
- Murray DW, Liddle AD, Dodd CA, Pandit H. Unicompartmental knee arthroplasty: is the glass half full or half empty? Bone Joint J. 2015;97-B(10 Suppl. A):3-8. doi: 10.1302/0301-620X.97B10.36542.
- Liddle AD, Judge A, Pandit H, Murray DW. Adverse outcomes after total and unicompartmental knee replacement in 101,330 matched patients: a study of data from the National Joint Registry for England and Wales. Lancet. 2014;384(9952):1437-1445. doi: 10.1016/S0140-6736(14)60419-0.
- Riddle DL, Jiranek WA, McGlynn FJ. Yearly incidence of unicompartmental knee arthroplasty in the United States. J Arthroplast. 2008;23(3):408-412. doi: 10.1016/j.arth.2007.04.012.
- Argenson JN, Blanc G, Aubaniac JM, Parratte S. Modern unicompartmental knee arthroplasty with cement: a concise follow-up, at a mean of twenty years, of a previous report. J Bone Joint Surg, (Am). 2013;95(10):905-909. doi: 10.2106/JBJS.L.00963.
- Koskinen E, Eskelinen A, Paavolainen P, Pulkkinen P, Remes V. Comparison of survival and cost-effectiveness between unicondylar arthroplasty and total knee arthroplasty in patients with primary osteoarthritis: a follow-up study of 50,493 knee replacements from the Finnish Arthroplasty Register. Acta Orthop. 2008;79(4):499-507. doi: 10.1080/17453670710015490.
- Knutson K, Lewold S, Robertsson O, Lidgren L. The Swedish knee arthroplasty register. A nation-wide study of 30,003 knees 1976-1992. Acta Orthop Scand. 1994;65(4):375-386. doi: 10.3109/17453679408995475.
- Kozinn SC, Scott R. Unicondylar knee arthroplasty. J Bone Joint Surg, (Am). 1989;71(1):145-150. doi: 10.2106/00004623-198971010-00023.
- Pennington DW. Unicompartmental knee arthroplasty in patients sixty years of age or younger. J Bone Joint Surg, (Am). 2003;85-A(10):1968-1973. doi: 10.2106/00004623-200310000-00016.
- Biswas D, Van Thiel GS, Wetters NG, Pack BJ, Berger RA, Della Valle CJ. Medial unicompartmental knee arthroplasty in patients less than 55 years old: minimum of two years of follow-up. J Arthroplast. 2014;29(1):101-105. doi: 10.1016/j.arth.2013.04.046.
- Murray DW, Pandit H, Weston-Simons JS, et al. Does body mass index affect the outcome of unicompartmental knee replacement? Knee. 2013;20(6):461-465. doi: 10.1016/j.knee.2012.09.017.
- Kang SN, Smith TO, Sprenger De Rover WB, Walton NP. Pre-operative patellofemoral degenerative changes do not affect the outcome after medial Oxford unicompartmental knee replacement: a report from an independent centre. J Bone Joint Surg Br. 2011;93(4):476-478. doi: 10.1302/0301-620X.93B4.25562.
- Pandit H, Jenkins C, Gill HS, et al. Unnecessary contraindications for mobile-bearing unicompartmental knee replacement. J Bone Joint Surg Br. 2011;93(5):622-628. doi: 10.1302/0301-620X.93B5.26214.
- Kurtz S, Mowat F, Ong K, Chan N, Lau E, Halpern M. Prevalence of primary and revision total hip and knee arthroplasty in the United States from 1990 through 2002. J Bone Joint Surg Am. 2005;87(7):1487-1497. doi: 10.2106/JBJS.D.02441.
- Kurtz SM, Ong KL, Schmier J, et al. Future clinical and economic impact of revision total hip and knee arthroplasty. J Bone Joint Surg, (Am). 2007;89(Suppl. 3):144-151. doi: 10.2106/JBJS.G.00587.
- Day JS, Lau E, Ong KL, Williams GR, Ramsey ML, Kurtz SM. Prevalence and projections of total shoulder and elbow arthroplasty in the United States to 2015. J Shoulder Elbow Surg. 2010;19(8):1115-1120. doi: 10.1016/j.jse.2010.02.009.
- Kurtz S, Ong K, Lau E, Mowat F, Halpern M. Projections of primary and revision hip and knee arthroplasty in the United States from 2005 to 2030. J Bone Joint Surg, (Am). 2007;89(4):780-785. doi: 10.2106/JBJS.F.00222.
- Kamath AF, Ong KL, Lau E, et al. Quantifying the burden of revision total joint arthroplasty for periprosthetic infection. J Arthroplast. 2015;30(9):1492-1497. doi: 10.1016/j.arth.2015.03.035.
- Bolognesi MP, Greiner MA, Attarian DE, et al. Unicompartmental knee arthroplasty and total knee arthroplasty among Medicare beneficiaries, 2000 to 2009. J Bone Joint Surg, (Am). 2013;95(22):e174. doi: 10.2106/JBJS.L.00652.
- Charlson ME, Pompei P, Ales KL, MacKenzie CR. A new method of classifying prognostic comorbidity in longitudinal studies: development and validation. J Chronic Dis. 1987;40(5):373-383. doi: 10.1016/0021-9681(87)90171-8.
- Kurtz SM, Ong KL, Lau E, Bozic KJ. Impact of the economic downturn on total joint replacement demand in the United States: updated projections to 2021. J Bone Joint Surg, (Am). 2014;96(8):624-630. doi: 10.2106/JBJS.M.00285.
- Leskinen J, Eskelinen A, Huhtala H, Paavolainen P, Remes V. The incidence of knee arthroplasty for primary osteoarthritis grows rapidly among baby boomers: a population-based study in Finland. Arthritis Rheum. 2012;64(2):423-428. doi: 10.1002/art.33367.
- Cartier P, Sanouiller JL, Grelsamer RP. Unicompartmental knee arthroplasty surgery. 10-year minimum follow-up period. J Arthroplast. 1996;11(7):782-788. doi: 10.1016/S0883-5403(96)80177-X.
- Kurtz SM, Lau E, Ong K, Zhao K, Kelly M, Bozic KJ. Future young patient demand for primary and revision joint replacement: national projections from 2010 to 2030. Clin Orthop Relat Res. 2009;467(10):2606-2612. doi: 10.1007/s11999-009-0834-6.
- Singh JA, Lu X, Rosenthal GE, Ibrahim S, Cram P. Racial disparities in knee and hip total joint arthroplasty: an 18-year analysis of national Medicare data. Ann Rheum Dis. 2014;73(12):2107-2115. doi: 10.1136/annrheumdis-2013-203494.
- Pierce TP, Elmallah RK, Lavernia CJ, et al. Racial disparities in lower extremity arthroplasty outcomes and use. Orthopedics. 2015;38(12): e1139-e1146. doi: 10.3928/01477447-20151123-05.
- Irgit K, Nelson CL. Defining racial and ethnic disparities in THA and TKA. Clin Orthop Relat Res. 2011;469(7):1817-1823. doi: 10.1007/s11999-011-1885-z.
ABSTRACT
Publications on the prevalence of unicompartmental knee arthroplasty in the United States using a single database may have underestimated the “true” number of cases performed, given that several unicondylar knee arthroplasty (UKA) patients are <65 years and have private insurance. The prevalence of UKA in elderly (≥65 years) and younger (<65 years) populations was evaluated using the 2002 to 2011 5% sample of the Medicare data (Part B) and the 2004 to June 2012 MarketScan Commercial and Medicare Supplemental databases, respectively. The prevalence of UKA was stratified by age, gender, census region, Charlson comorbidity index, Medicare buy-in status, and diagnosis. The annual rate of change in the UKA rate was examined using Poisson regression to evaluate temporal changes considering year as a covariate.
A total of 5235 and 23,310 UKA procedures were identified from the 5% Medicare and MarketScan databases, respectively. The rates of UKA generally increased until 2008, after which there was a decline. In both cohorts, gender and year of operation were found to be significantly associated with UKA rate. Analysis of data obtained over the past few years revealed that males 55 to 64 years, 65 to 69 years, and 70 to 74 years were the only age-gender groups whose UKA rates appeared to be trending upward.
From 2002 to 2011, the rate of UKAs performed in the United States has increased, and a significant proportion of the surgeries were performed in younger (<65 years) patients.
Continue to: Unicondylar knee arthroplasty...
Unicondylar knee arthroplasty (UKA) is an effective surgical treatment for symptomatic degenerative joint disease of a single compartment of the knee, providing improved functional outcomes compared with total knee arthroplasty (TKA).1-3 It has been estimated that the proportion of patients undergoing TKA, who meet the criteria for UKA, varies between 21% and 47%.4,5 However, it has been variably estimated that the usage of UKA ranges from 0% to 50% (mean, 8%) of all primary knee arthroplasties.5-8 It is believed that this discrepancy between the percentage of patients who meet indications for the surgery and those who receive it is associated with various factors, including surgeon training and experiences, diverse indications, economic factors, as well as acknowledgment of the reportedly higher revision rates of UKA than those of TKA in national joint registries.7,9-11
According to their classic article, Kozinn and Scott12 outlined the indications for UKA that, in their experience, led to the most successful outcomes, including age >60 years, weight <82 kg, low physical demand, localized arthritis with no full-thickness chondromalacia elsewhere in the joint, intact anterior cruciate ligament, minimal deformity, and flexion >90°. More recently, indications have been expanded to include younger and more active patients, higher body mass index, and some patterns of patellofemoral chondromalacia, with an increasing number of publications reporting successful clinical outcomes in these cohorts as well.13-17 Taken together, it is clear that the “classic” strict indications for UKA can be safely expanded, which have and will result in an increased number of these procedures being performed above and beyond that which might be predicted based on demographic trends alone.
A growing body of literature has been published on the prevalence and projections of orthopedic procedures in the United States.18-20 Several studies have focused their analysis on 1 of several large administrative databases, including the Nationwide Inpatient Sample, the 5% Medicare Part B database, and the National Hospital Discharge Survey.18,20-23 A concern with limiting an analysis of the prevalence of unicompartmental knee arthroplasty to these particular databases is that it may underestimate the “true” number of cases performed in the United States, given that several UKA patients are <65 years and have private insurance, and therefore, would not be captured statistically by a database that collects data on patients ≥65 years.
The purpose of this study was to quantify the current prevalence and epidemiology of UKA in the U.S. patient population. Our hypothesis was that the number of procedures and the procedural rate of UKA are increasing over time. Furthermore, this increase may be attributed to an increase in select age- or gender-based segments of the population. To test this hypothesis, we analyzed 2 separate large claims databases to capture patients over a spectrum of age and inclusive of both private and public payers, including the 5% Medicare Part B database (2002–2011) for patients ≥65 years and the MarketScan database (2004 to June 2011) for patients <65 years. Understanding the accurate trends in the use of UKA on a national scale is important for legislative bodies, healthcare administrators, and physicians.
MATERIALS AND METHODS
The 2002 to 2011 5% sample of the Medicare data (Part B) and the 2004 to June 2012 MarketScan Commercial and Medicare Supplemental databases were used to evaluate the prevalence of UKA in elderly (≥65 years) and younger (<65 years) populations, respectively. The UKA procedures were identified using the CPT code 27446.
The prevalence of UKA was stratified by age, gender, census region, Charlson Comorbidity Index, Medicare buy-in status, and diagnosis. The buy-in status is a proxy for the socioeconomic status as it reflects the state subsidizing the health insurance premium for the beneficiary. The Charlson Comorbidity Index is a composite score that has been used to assess the comorbidity level of a patient by taking into account the number and the severity of comorbid conditions.24 For the elderly population, the rate of UKA was subsequently evaluated based on the number of beneficiaries for that particular age-gender group and year in both databases. Poisson regression was used to evaluate the annual rate of change in the UKA rate for assessing temporal changes considering year as a covariate. Age and gender, as well as 2-way interaction terms for age, gender, and year, were also considered as covariates.
Continue to: RESULTS...
RESULTS
For the time periods analyzed, a total of 5235 and 23,310 UKA procedures were identified from the 5% Medicare and MarketScan databases, respectively. A peak in the prevalence appeared around 2008 for the elderly population and in 2009 for the younger population (Figure 1). When normalized by the size of the population segment, the rate of UKA was found to be approximately 5 times greater in the elderly population, increasing from 369 in 2002 to 639 in 2008, but plateauing to 561 in 2011. Extrapolating to the 100% Medicare population, these numbers increased to 7380, 12,780, and 11,220, respectively. Temporal changes in the UKA rate were significant, increasing from 24.5 UKAs per 100,000 persons in 2002 to 43.1 UKAs in 2008, followed by a decline to 36.5 in 2011 (P < .0001) (Figure 2). The rates of UKA generally increased from 2002 to 2008 for both males and females in the Medicare cohort; however, the rates of UKA in female patients continuously declined from 2008 onward, whereas the UKA rates in male patients decreased in 2009, followed by an increase in 2010 and 2011 (Figure 2). For the younger population, there was a slight increase in the rate of UKA from 2004 to approximately 2009, after which the rates for both males and females remained relatively steady. When put in the context of the prevalence of TKA, the prevalence of UKA fluctuated during the same time period. In the Medicare population, the prevalence of UKA ranged from 4.3% (2005) to 5.9% (2008) of the TKA prevalence between 2002 and 2011. In the younger MarketScan population, the prevalence of UKA ranged from 6.7% (2005) to 8.9% (2008) between 2004 and June 2012.
The UKA rate differed significantly according to gender (P = .0209), with higher rates for males. Although there were no age-related differences (P = .3723), age–gender interactions were found to be significant (P < .0001). For males, the largest rate of UKA in the most recent year of data was observed in the 70- to 74-year-old group, followed by the 75- to 79- and the 65- to 69-year-old groups (Figure 3). For females, those in the 65- to 69- and the 70- to 74-year-old groups had the highest rate of UKA. In the younger cohort, there were increases in the UKA rates since 2004. These rates appeared to be relatively stable from the 2008 or 2009 period onward, except for females 55–64 years, which demonstrated a steady decline since 2008. Analysis of data obtained over the past few years showed that males 55–64, 65–69, and 70–74 years were the only age–gender groups whose UKA rates appeared to be trending upward.
The vast majority of elderly UKA patients were white (95.5%), and when stratified by census region, the highest proportion of UKA procedures was observed in the South and the Midwest (Figure 4). Furthermore, among patients <65 years, 64.2% had a Charlson score of 0 compared to 40.8% in the elderly group (Figure 5). For the Medicare population, based on their receipt of state subsidies for their insurance premiums, 5.1% of patients were of lower socioeconomic status. Osteoarthritis was diagnosed in 99.4% and 97.3% of the MarketScan and Medicare cohorts, respectively.
In the Medicare cohort, gender (P = .0209) and year of operation (P < .0001) were found to be significantly associated with the rate of UKA, along with age-gender (P < .0001) and gender-year (P = .0202) interaction terms. In the MarketScan cohort, age (P = .0173), gender (P = .0017), and year of operation (P = .0002) were found to be significantly associated with UKA rate. Two-way interactions between age-gender (P = .0018), age–year (P = .0207), and gender-year (P = .0017) were also found to be statistically significant factors.
Continue to: DISCUSSION...
DISCUSSION
The results of our study indicate that between 2002 and 2011, a steadily increasing number of UKA procedures was performed in the United States, and a significant proportion of the surgeries was performed on patients <65 years. Without the MarketScan database data, we would have missed more than 23,000 UKA cases performed during this 10-year time period. This finding validates our research methodology that incorporated data on privately insured younger (<65 years) patients, which is something that has not been done when examining the epidemiology of UKA.
To our knowledge, there are only 2 other publications attempting to quantify the incidence of UKA procedures performed in the United States. Bolognesi and colleagues23 used the Medicare 5% sample to assess trends in the use of knee arthroplasty from 2000 to 2009. The authors reported that a total of 68,603 patients underwent unilateral total knee arthroplasty (n = 65,505) or unicompartmental knee arthroplasty (n = 3098) over this 10-year time period. Given that there is substantial overlap of our time periods, it is not surprising that our Medicare numbers are similar (3098 vs 5235). In their study, the use of TKA increased 1.7-fold, whereas the use of UKA increased 6.2-fold23. In our analysis of the Medicare (2011 vs 2002) and MarketScan (2011 vs 2004) databases, there was a 1.3-fold and a 3.4-fold increase in the number of TKAs performed. Concomitantly, the use of UKA increased 1.5-fold and 2.8-fold, respectively, in these databases over the same time periods. The reason for the slight discrepancy in the numbers may be attributable to the peak occurring in 2008. The other publication on the subject by Riddle and colleagues8 focused on the time period 1998 to 2005 and used implant manufacturer’s sales data cross-referenced to a database of 44 hospitals to derive their national estimates. Using their unique methodology, the authors calculated an incidence of UKA, ranging from 6570 implants in 1998 to 44,990 in 2005. They reported that UKA use during the study period increased by 3 times the rate of TKA in the United States, with an average yearly percentage increase in the number of UKA procedures of 32.5% compared to 9.4% for TKA procedures. It is difficult to account for the discrepancy in the number of UKAs performed reported between our current study and that of Riddle and colleagues;8 however, the fact that the authors used implant manufacturer’s individual sales numbers may indicate that a portion of UKA patients was not captured in either the Medicare 5% or the MarketScan database. Nonetheless, in our analysis, the annual increase in the number of UKA procedures performed during the time periods studied averaged 5.8% in the older population and 25.4% in the younger population compared to the increase in the number of TKA procedures, which averaged 3.6% and 33.9% in the older and younger populations, respectively. In addition, in our study, the percentage of UKAs performed relative to the number of TKAs during the time intervals studied varied from a low of 4.3% to a high of 5.9% in the older population and from a low of 6.7% to a high of 8.9% in the younger population.
During the 10-year period of this study, a general upward trend appeared in the total number of unicompartmental knee arthroplasties performed in both the Medicare and the MarketScan databases. The rate at which the procedure was performed increased in the Medicare population from 24.5 to 36.5 (per 100,000 persons) over a 10-year time period and in the MarketScan cohort from 5.9 to 7.4 (per 100,000 persons) over an 8.5-year time period. This indicates both a larger absolute and a relative rate increase in UKA procedures in the elderly population. Around 2008 and 2009, the data showed a slight dip in the rate of UKA in the Medicare population and a plateau in the rate in the MarketScan database. Although this may be a spurious finding in the data that would be smoothed out with a longer time period investigated, it is interesting that this finding coincided with a national economic downturn. Although it might be expected that macroeconomics may affect the utilization of elective surgery such as total joint replacement, Kurtz and colleagues25 investigated this particular question and found that neither the economic downturns of 2001 or those of 2008 and 2009 had a significant impact on the incidence of total joint replacement surgeries.
Incorporation of the MarketScan database data indicated that a significant proportion of patients undergoing UKA were <65 years and that there was a slight but increasing rate of procedures performed on this age cohort over the past decade. A similar finding has been reported in the Finnish Arthroplasty Registry. Leskinen and colleagues26 reported that the incidence of UKAs among individuals 30 to 59 years increased from 0.2 (per 100,000 persons) to 10 (per 100,000 persons) from 1980 to 2006 and that most of the increase occurred among patients 50 to 59 years. The fact that younger age is no longer observed as a relative contraindication to this procedure is supported by several clinical investigations. Cartier and colleagues27 reported 93% survival at 10 years in patients with a mean age of 65 years, but included patients as young as 28 years, claiming that the results for younger patients were no worse than those for older patients in the series. Pandit and colleagues17 compared the results of 245 young patients (<60 years) to those of 755 older patients (>60 years) and found a survival rate of 97% at 10 years, with no significant difference in mean functional outcomes, failure rate, or survival between the groups at >5 years of follow-up. Given that patients <65 years now account for approximately half of the TKAs performed each year, with the greatest increase in volume among patients between 45 and 54, it is clear that investigations on the epidemiology of UKA must take into account this increasingly relevant younger patient cohort.28
Continue to: Our data indicate...
Our data indicate that only approximately 5% of UKA patients were non-white and another 5% were from lower socioeconomic status. These findings have been observed in multiple other studies looking at the epidemiology of total joint replacement in the United States.29 Bolognesi and colleagues23 reported that although “non-white race” patients made up 12% of the general Medicare sample they were analyzing, these patients accounted for only 5% and 3% of the total knee arthroplasty and unicompartmental knee arthroplasty populations, respectively. Although it is beyond the scope of this paper to delve into the reasons for this discrepancy, it may be related to differences in access to care, healthcare literacy, and trust of patients in the healthcare system.30,31
Our study, like all those based on administrative claims, has several notable inherent limitations. Coding inaccuracies as well as the potential for systematic bias (eg, underreporting) may affect the accuracy of our results. Although the MarketScan Commercial Research Database (Truven Health Analytics) includes nationally representative information for >180 million patients covered with private insurance, it is possible that we have missed some patients who underwent UKA during the time period investigated. However, we feel that the number missed is probably small and does not affect our conclusions in any meaningful manner.
CONCLUSION
This novel analysis of 2 separate administrative claims databases, which more accurately captures all patients undergoing UKA, indicates that there has been a steady increase in the rate of the procedure over the past decade and that a significant proportion of the surgeries were performed in younger (<65 years) patients. Understanding the accurate trends in the use of UKA on a national scale is important for legislative bodies, healthcare administrators, as well as physicians. Furthermore, given the increasing rates of UKA in patients <65 years old, and the increased burden on implants for withstanding increased activities and repetitive loads, it remains imperative to strive to optimize materials, implant designs, and surgical techniques to enhance implant durability.
ABSTRACT
Publications on the prevalence of unicompartmental knee arthroplasty in the United States using a single database may have underestimated the “true” number of cases performed, given that several unicondylar knee arthroplasty (UKA) patients are <65 years and have private insurance. The prevalence of UKA in elderly (≥65 years) and younger (<65 years) populations was evaluated using the 2002 to 2011 5% sample of the Medicare data (Part B) and the 2004 to June 2012 MarketScan Commercial and Medicare Supplemental databases, respectively. The prevalence of UKA was stratified by age, gender, census region, Charlson comorbidity index, Medicare buy-in status, and diagnosis. The annual rate of change in the UKA rate was examined using Poisson regression to evaluate temporal changes considering year as a covariate.
A total of 5235 and 23,310 UKA procedures were identified from the 5% Medicare and MarketScan databases, respectively. The rates of UKA generally increased until 2008, after which there was a decline. In both cohorts, gender and year of operation were found to be significantly associated with UKA rate. Analysis of data obtained over the past few years revealed that males 55 to 64 years, 65 to 69 years, and 70 to 74 years were the only age-gender groups whose UKA rates appeared to be trending upward.
From 2002 to 2011, the rate of UKAs performed in the United States has increased, and a significant proportion of the surgeries were performed in younger (<65 years) patients.
Continue to: Unicondylar knee arthroplasty...
Unicondylar knee arthroplasty (UKA) is an effective surgical treatment for symptomatic degenerative joint disease of a single compartment of the knee, providing improved functional outcomes compared with total knee arthroplasty (TKA).1-3 It has been estimated that the proportion of patients undergoing TKA, who meet the criteria for UKA, varies between 21% and 47%.4,5 However, it has been variably estimated that the usage of UKA ranges from 0% to 50% (mean, 8%) of all primary knee arthroplasties.5-8 It is believed that this discrepancy between the percentage of patients who meet indications for the surgery and those who receive it is associated with various factors, including surgeon training and experiences, diverse indications, economic factors, as well as acknowledgment of the reportedly higher revision rates of UKA than those of TKA in national joint registries.7,9-11
According to their classic article, Kozinn and Scott12 outlined the indications for UKA that, in their experience, led to the most successful outcomes, including age >60 years, weight <82 kg, low physical demand, localized arthritis with no full-thickness chondromalacia elsewhere in the joint, intact anterior cruciate ligament, minimal deformity, and flexion >90°. More recently, indications have been expanded to include younger and more active patients, higher body mass index, and some patterns of patellofemoral chondromalacia, with an increasing number of publications reporting successful clinical outcomes in these cohorts as well.13-17 Taken together, it is clear that the “classic” strict indications for UKA can be safely expanded, which have and will result in an increased number of these procedures being performed above and beyond that which might be predicted based on demographic trends alone.
A growing body of literature has been published on the prevalence and projections of orthopedic procedures in the United States.18-20 Several studies have focused their analysis on 1 of several large administrative databases, including the Nationwide Inpatient Sample, the 5% Medicare Part B database, and the National Hospital Discharge Survey.18,20-23 A concern with limiting an analysis of the prevalence of unicompartmental knee arthroplasty to these particular databases is that it may underestimate the “true” number of cases performed in the United States, given that several UKA patients are <65 years and have private insurance, and therefore, would not be captured statistically by a database that collects data on patients ≥65 years.
The purpose of this study was to quantify the current prevalence and epidemiology of UKA in the U.S. patient population. Our hypothesis was that the number of procedures and the procedural rate of UKA are increasing over time. Furthermore, this increase may be attributed to an increase in select age- or gender-based segments of the population. To test this hypothesis, we analyzed 2 separate large claims databases to capture patients over a spectrum of age and inclusive of both private and public payers, including the 5% Medicare Part B database (2002–2011) for patients ≥65 years and the MarketScan database (2004 to June 2011) for patients <65 years. Understanding the accurate trends in the use of UKA on a national scale is important for legislative bodies, healthcare administrators, and physicians.
MATERIALS AND METHODS
The 2002 to 2011 5% sample of the Medicare data (Part B) and the 2004 to June 2012 MarketScan Commercial and Medicare Supplemental databases were used to evaluate the prevalence of UKA in elderly (≥65 years) and younger (<65 years) populations, respectively. The UKA procedures were identified using the CPT code 27446.
The prevalence of UKA was stratified by age, gender, census region, Charlson Comorbidity Index, Medicare buy-in status, and diagnosis. The buy-in status is a proxy for the socioeconomic status as it reflects the state subsidizing the health insurance premium for the beneficiary. The Charlson Comorbidity Index is a composite score that has been used to assess the comorbidity level of a patient by taking into account the number and the severity of comorbid conditions.24 For the elderly population, the rate of UKA was subsequently evaluated based on the number of beneficiaries for that particular age-gender group and year in both databases. Poisson regression was used to evaluate the annual rate of change in the UKA rate for assessing temporal changes considering year as a covariate. Age and gender, as well as 2-way interaction terms for age, gender, and year, were also considered as covariates.
Continue to: RESULTS...
RESULTS
For the time periods analyzed, a total of 5235 and 23,310 UKA procedures were identified from the 5% Medicare and MarketScan databases, respectively. A peak in the prevalence appeared around 2008 for the elderly population and in 2009 for the younger population (Figure 1). When normalized by the size of the population segment, the rate of UKA was found to be approximately 5 times greater in the elderly population, increasing from 369 in 2002 to 639 in 2008, but plateauing to 561 in 2011. Extrapolating to the 100% Medicare population, these numbers increased to 7380, 12,780, and 11,220, respectively. Temporal changes in the UKA rate were significant, increasing from 24.5 UKAs per 100,000 persons in 2002 to 43.1 UKAs in 2008, followed by a decline to 36.5 in 2011 (P < .0001) (Figure 2). The rates of UKA generally increased from 2002 to 2008 for both males and females in the Medicare cohort; however, the rates of UKA in female patients continuously declined from 2008 onward, whereas the UKA rates in male patients decreased in 2009, followed by an increase in 2010 and 2011 (Figure 2). For the younger population, there was a slight increase in the rate of UKA from 2004 to approximately 2009, after which the rates for both males and females remained relatively steady. When put in the context of the prevalence of TKA, the prevalence of UKA fluctuated during the same time period. In the Medicare population, the prevalence of UKA ranged from 4.3% (2005) to 5.9% (2008) of the TKA prevalence between 2002 and 2011. In the younger MarketScan population, the prevalence of UKA ranged from 6.7% (2005) to 8.9% (2008) between 2004 and June 2012.
The UKA rate differed significantly according to gender (P = .0209), with higher rates for males. Although there were no age-related differences (P = .3723), age–gender interactions were found to be significant (P < .0001). For males, the largest rate of UKA in the most recent year of data was observed in the 70- to 74-year-old group, followed by the 75- to 79- and the 65- to 69-year-old groups (Figure 3). For females, those in the 65- to 69- and the 70- to 74-year-old groups had the highest rate of UKA. In the younger cohort, there were increases in the UKA rates since 2004. These rates appeared to be relatively stable from the 2008 or 2009 period onward, except for females 55–64 years, which demonstrated a steady decline since 2008. Analysis of data obtained over the past few years showed that males 55–64, 65–69, and 70–74 years were the only age–gender groups whose UKA rates appeared to be trending upward.
The vast majority of elderly UKA patients were white (95.5%), and when stratified by census region, the highest proportion of UKA procedures was observed in the South and the Midwest (Figure 4). Furthermore, among patients <65 years, 64.2% had a Charlson score of 0 compared to 40.8% in the elderly group (Figure 5). For the Medicare population, based on their receipt of state subsidies for their insurance premiums, 5.1% of patients were of lower socioeconomic status. Osteoarthritis was diagnosed in 99.4% and 97.3% of the MarketScan and Medicare cohorts, respectively.
In the Medicare cohort, gender (P = .0209) and year of operation (P < .0001) were found to be significantly associated with the rate of UKA, along with age-gender (P < .0001) and gender-year (P = .0202) interaction terms. In the MarketScan cohort, age (P = .0173), gender (P = .0017), and year of operation (P = .0002) were found to be significantly associated with UKA rate. Two-way interactions between age-gender (P = .0018), age–year (P = .0207), and gender-year (P = .0017) were also found to be statistically significant factors.
Continue to: DISCUSSION...
DISCUSSION
The results of our study indicate that between 2002 and 2011, a steadily increasing number of UKA procedures was performed in the United States, and a significant proportion of the surgeries was performed on patients <65 years. Without the MarketScan database data, we would have missed more than 23,000 UKA cases performed during this 10-year time period. This finding validates our research methodology that incorporated data on privately insured younger (<65 years) patients, which is something that has not been done when examining the epidemiology of UKA.
To our knowledge, there are only 2 other publications attempting to quantify the incidence of UKA procedures performed in the United States. Bolognesi and colleagues23 used the Medicare 5% sample to assess trends in the use of knee arthroplasty from 2000 to 2009. The authors reported that a total of 68,603 patients underwent unilateral total knee arthroplasty (n = 65,505) or unicompartmental knee arthroplasty (n = 3098) over this 10-year time period. Given that there is substantial overlap of our time periods, it is not surprising that our Medicare numbers are similar (3098 vs 5235). In their study, the use of TKA increased 1.7-fold, whereas the use of UKA increased 6.2-fold23. In our analysis of the Medicare (2011 vs 2002) and MarketScan (2011 vs 2004) databases, there was a 1.3-fold and a 3.4-fold increase in the number of TKAs performed. Concomitantly, the use of UKA increased 1.5-fold and 2.8-fold, respectively, in these databases over the same time periods. The reason for the slight discrepancy in the numbers may be attributable to the peak occurring in 2008. The other publication on the subject by Riddle and colleagues8 focused on the time period 1998 to 2005 and used implant manufacturer’s sales data cross-referenced to a database of 44 hospitals to derive their national estimates. Using their unique methodology, the authors calculated an incidence of UKA, ranging from 6570 implants in 1998 to 44,990 in 2005. They reported that UKA use during the study period increased by 3 times the rate of TKA in the United States, with an average yearly percentage increase in the number of UKA procedures of 32.5% compared to 9.4% for TKA procedures. It is difficult to account for the discrepancy in the number of UKAs performed reported between our current study and that of Riddle and colleagues;8 however, the fact that the authors used implant manufacturer’s individual sales numbers may indicate that a portion of UKA patients was not captured in either the Medicare 5% or the MarketScan database. Nonetheless, in our analysis, the annual increase in the number of UKA procedures performed during the time periods studied averaged 5.8% in the older population and 25.4% in the younger population compared to the increase in the number of TKA procedures, which averaged 3.6% and 33.9% in the older and younger populations, respectively. In addition, in our study, the percentage of UKAs performed relative to the number of TKAs during the time intervals studied varied from a low of 4.3% to a high of 5.9% in the older population and from a low of 6.7% to a high of 8.9% in the younger population.
During the 10-year period of this study, a general upward trend appeared in the total number of unicompartmental knee arthroplasties performed in both the Medicare and the MarketScan databases. The rate at which the procedure was performed increased in the Medicare population from 24.5 to 36.5 (per 100,000 persons) over a 10-year time period and in the MarketScan cohort from 5.9 to 7.4 (per 100,000 persons) over an 8.5-year time period. This indicates both a larger absolute and a relative rate increase in UKA procedures in the elderly population. Around 2008 and 2009, the data showed a slight dip in the rate of UKA in the Medicare population and a plateau in the rate in the MarketScan database. Although this may be a spurious finding in the data that would be smoothed out with a longer time period investigated, it is interesting that this finding coincided with a national economic downturn. Although it might be expected that macroeconomics may affect the utilization of elective surgery such as total joint replacement, Kurtz and colleagues25 investigated this particular question and found that neither the economic downturns of 2001 or those of 2008 and 2009 had a significant impact on the incidence of total joint replacement surgeries.
Incorporation of the MarketScan database data indicated that a significant proportion of patients undergoing UKA were <65 years and that there was a slight but increasing rate of procedures performed on this age cohort over the past decade. A similar finding has been reported in the Finnish Arthroplasty Registry. Leskinen and colleagues26 reported that the incidence of UKAs among individuals 30 to 59 years increased from 0.2 (per 100,000 persons) to 10 (per 100,000 persons) from 1980 to 2006 and that most of the increase occurred among patients 50 to 59 years. The fact that younger age is no longer observed as a relative contraindication to this procedure is supported by several clinical investigations. Cartier and colleagues27 reported 93% survival at 10 years in patients with a mean age of 65 years, but included patients as young as 28 years, claiming that the results for younger patients were no worse than those for older patients in the series. Pandit and colleagues17 compared the results of 245 young patients (<60 years) to those of 755 older patients (>60 years) and found a survival rate of 97% at 10 years, with no significant difference in mean functional outcomes, failure rate, or survival between the groups at >5 years of follow-up. Given that patients <65 years now account for approximately half of the TKAs performed each year, with the greatest increase in volume among patients between 45 and 54, it is clear that investigations on the epidemiology of UKA must take into account this increasingly relevant younger patient cohort.28
Continue to: Our data indicate...
Our data indicate that only approximately 5% of UKA patients were non-white and another 5% were from lower socioeconomic status. These findings have been observed in multiple other studies looking at the epidemiology of total joint replacement in the United States.29 Bolognesi and colleagues23 reported that although “non-white race” patients made up 12% of the general Medicare sample they were analyzing, these patients accounted for only 5% and 3% of the total knee arthroplasty and unicompartmental knee arthroplasty populations, respectively. Although it is beyond the scope of this paper to delve into the reasons for this discrepancy, it may be related to differences in access to care, healthcare literacy, and trust of patients in the healthcare system.30,31
Our study, like all those based on administrative claims, has several notable inherent limitations. Coding inaccuracies as well as the potential for systematic bias (eg, underreporting) may affect the accuracy of our results. Although the MarketScan Commercial Research Database (Truven Health Analytics) includes nationally representative information for >180 million patients covered with private insurance, it is possible that we have missed some patients who underwent UKA during the time period investigated. However, we feel that the number missed is probably small and does not affect our conclusions in any meaningful manner.
CONCLUSION
This novel analysis of 2 separate administrative claims databases, which more accurately captures all patients undergoing UKA, indicates that there has been a steady increase in the rate of the procedure over the past decade and that a significant proportion of the surgeries were performed in younger (<65 years) patients. Understanding the accurate trends in the use of UKA on a national scale is important for legislative bodies, healthcare administrators, as well as physicians. Furthermore, given the increasing rates of UKA in patients <65 years old, and the increased burden on implants for withstanding increased activities and repetitive loads, it remains imperative to strive to optimize materials, implant designs, and surgical techniques to enhance implant durability.
- Hopper GP, Leach WJ. Participation in sporting activities following knee replacement: total versus unicompartmental. Knee Surg Sports Traumatol Arthrosc. 2008;16(10):973-979. doi: 10.1007/s00167-008-0596-9.
- Lygre SH, Espehaug B, Havelin LI, Furnes O, Vollset SE. Pain and function in patients after primary unicompartmental and total knee arthroplasty. J Bone Joint Surg, (Am). 2010;92(18):2890-2897. doi: 10.2106/JBJS.I.00917.
- Liddle AD, Pandit H, Judge A, Murray DW. Patient-reported outcomes after total and unicompartmental knee arthroplasty: a study of 14,076 matched patients from the National Joint Registry for England and Wales. Bone Joint J. 2015;97-B(6):793-801. doi: 10.1302/0301-620X.97B6.35155.
- Arno S, Maffei D, Walker PS, Schwarzkopf R, Desai P, Steiner GC. Retrospective analysis of total knee arthroplasty cases for visual, histological, and clinical eligibility of unicompartmental knee arthroplasties. J Arthroplast. 2011;26(8):1396-1403. doi: 10.1016/j.arth.2010.12.023.
- Willis-Owen CA, Brust K, Alsop H, Miraldo M, Cobb JP. Unicondylar knee arthroplasty in the UK National Health Service: an analysis of candidacy, outcome and cost efficacy. Knee. 2009;16(6):473-478. doi: 10.1016/j.knee.2009.04.006.
- Murray DW, Liddle AD, Dodd CA, Pandit H. Unicompartmental knee arthroplasty: is the glass half full or half empty? Bone Joint J. 2015;97-B(10 Suppl. A):3-8. doi: 10.1302/0301-620X.97B10.36542.
- Liddle AD, Judge A, Pandit H, Murray DW. Adverse outcomes after total and unicompartmental knee replacement in 101,330 matched patients: a study of data from the National Joint Registry for England and Wales. Lancet. 2014;384(9952):1437-1445. doi: 10.1016/S0140-6736(14)60419-0.
- Riddle DL, Jiranek WA, McGlynn FJ. Yearly incidence of unicompartmental knee arthroplasty in the United States. J Arthroplast. 2008;23(3):408-412. doi: 10.1016/j.arth.2007.04.012.
- Argenson JN, Blanc G, Aubaniac JM, Parratte S. Modern unicompartmental knee arthroplasty with cement: a concise follow-up, at a mean of twenty years, of a previous report. J Bone Joint Surg, (Am). 2013;95(10):905-909. doi: 10.2106/JBJS.L.00963.
- Koskinen E, Eskelinen A, Paavolainen P, Pulkkinen P, Remes V. Comparison of survival and cost-effectiveness between unicondylar arthroplasty and total knee arthroplasty in patients with primary osteoarthritis: a follow-up study of 50,493 knee replacements from the Finnish Arthroplasty Register. Acta Orthop. 2008;79(4):499-507. doi: 10.1080/17453670710015490.
- Knutson K, Lewold S, Robertsson O, Lidgren L. The Swedish knee arthroplasty register. A nation-wide study of 30,003 knees 1976-1992. Acta Orthop Scand. 1994;65(4):375-386. doi: 10.3109/17453679408995475.
- Kozinn SC, Scott R. Unicondylar knee arthroplasty. J Bone Joint Surg, (Am). 1989;71(1):145-150. doi: 10.2106/00004623-198971010-00023.
- Pennington DW. Unicompartmental knee arthroplasty in patients sixty years of age or younger. J Bone Joint Surg, (Am). 2003;85-A(10):1968-1973. doi: 10.2106/00004623-200310000-00016.
- Biswas D, Van Thiel GS, Wetters NG, Pack BJ, Berger RA, Della Valle CJ. Medial unicompartmental knee arthroplasty in patients less than 55 years old: minimum of two years of follow-up. J Arthroplast. 2014;29(1):101-105. doi: 10.1016/j.arth.2013.04.046.
- Murray DW, Pandit H, Weston-Simons JS, et al. Does body mass index affect the outcome of unicompartmental knee replacement? Knee. 2013;20(6):461-465. doi: 10.1016/j.knee.2012.09.017.
- Kang SN, Smith TO, Sprenger De Rover WB, Walton NP. Pre-operative patellofemoral degenerative changes do not affect the outcome after medial Oxford unicompartmental knee replacement: a report from an independent centre. J Bone Joint Surg Br. 2011;93(4):476-478. doi: 10.1302/0301-620X.93B4.25562.
- Pandit H, Jenkins C, Gill HS, et al. Unnecessary contraindications for mobile-bearing unicompartmental knee replacement. J Bone Joint Surg Br. 2011;93(5):622-628. doi: 10.1302/0301-620X.93B5.26214.
- Kurtz S, Mowat F, Ong K, Chan N, Lau E, Halpern M. Prevalence of primary and revision total hip and knee arthroplasty in the United States from 1990 through 2002. J Bone Joint Surg Am. 2005;87(7):1487-1497. doi: 10.2106/JBJS.D.02441.
- Kurtz SM, Ong KL, Schmier J, et al. Future clinical and economic impact of revision total hip and knee arthroplasty. J Bone Joint Surg, (Am). 2007;89(Suppl. 3):144-151. doi: 10.2106/JBJS.G.00587.
- Day JS, Lau E, Ong KL, Williams GR, Ramsey ML, Kurtz SM. Prevalence and projections of total shoulder and elbow arthroplasty in the United States to 2015. J Shoulder Elbow Surg. 2010;19(8):1115-1120. doi: 10.1016/j.jse.2010.02.009.
- Kurtz S, Ong K, Lau E, Mowat F, Halpern M. Projections of primary and revision hip and knee arthroplasty in the United States from 2005 to 2030. J Bone Joint Surg, (Am). 2007;89(4):780-785. doi: 10.2106/JBJS.F.00222.
- Kamath AF, Ong KL, Lau E, et al. Quantifying the burden of revision total joint arthroplasty for periprosthetic infection. J Arthroplast. 2015;30(9):1492-1497. doi: 10.1016/j.arth.2015.03.035.
- Bolognesi MP, Greiner MA, Attarian DE, et al. Unicompartmental knee arthroplasty and total knee arthroplasty among Medicare beneficiaries, 2000 to 2009. J Bone Joint Surg, (Am). 2013;95(22):e174. doi: 10.2106/JBJS.L.00652.
- Charlson ME, Pompei P, Ales KL, MacKenzie CR. A new method of classifying prognostic comorbidity in longitudinal studies: development and validation. J Chronic Dis. 1987;40(5):373-383. doi: 10.1016/0021-9681(87)90171-8.
- Kurtz SM, Ong KL, Lau E, Bozic KJ. Impact of the economic downturn on total joint replacement demand in the United States: updated projections to 2021. J Bone Joint Surg, (Am). 2014;96(8):624-630. doi: 10.2106/JBJS.M.00285.
- Leskinen J, Eskelinen A, Huhtala H, Paavolainen P, Remes V. The incidence of knee arthroplasty for primary osteoarthritis grows rapidly among baby boomers: a population-based study in Finland. Arthritis Rheum. 2012;64(2):423-428. doi: 10.1002/art.33367.
- Cartier P, Sanouiller JL, Grelsamer RP. Unicompartmental knee arthroplasty surgery. 10-year minimum follow-up period. J Arthroplast. 1996;11(7):782-788. doi: 10.1016/S0883-5403(96)80177-X.
- Kurtz SM, Lau E, Ong K, Zhao K, Kelly M, Bozic KJ. Future young patient demand for primary and revision joint replacement: national projections from 2010 to 2030. Clin Orthop Relat Res. 2009;467(10):2606-2612. doi: 10.1007/s11999-009-0834-6.
- Singh JA, Lu X, Rosenthal GE, Ibrahim S, Cram P. Racial disparities in knee and hip total joint arthroplasty: an 18-year analysis of national Medicare data. Ann Rheum Dis. 2014;73(12):2107-2115. doi: 10.1136/annrheumdis-2013-203494.
- Pierce TP, Elmallah RK, Lavernia CJ, et al. Racial disparities in lower extremity arthroplasty outcomes and use. Orthopedics. 2015;38(12): e1139-e1146. doi: 10.3928/01477447-20151123-05.
- Irgit K, Nelson CL. Defining racial and ethnic disparities in THA and TKA. Clin Orthop Relat Res. 2011;469(7):1817-1823. doi: 10.1007/s11999-011-1885-z.
- Hopper GP, Leach WJ. Participation in sporting activities following knee replacement: total versus unicompartmental. Knee Surg Sports Traumatol Arthrosc. 2008;16(10):973-979. doi: 10.1007/s00167-008-0596-9.
- Lygre SH, Espehaug B, Havelin LI, Furnes O, Vollset SE. Pain and function in patients after primary unicompartmental and total knee arthroplasty. J Bone Joint Surg, (Am). 2010;92(18):2890-2897. doi: 10.2106/JBJS.I.00917.
- Liddle AD, Pandit H, Judge A, Murray DW. Patient-reported outcomes after total and unicompartmental knee arthroplasty: a study of 14,076 matched patients from the National Joint Registry for England and Wales. Bone Joint J. 2015;97-B(6):793-801. doi: 10.1302/0301-620X.97B6.35155.
- Arno S, Maffei D, Walker PS, Schwarzkopf R, Desai P, Steiner GC. Retrospective analysis of total knee arthroplasty cases for visual, histological, and clinical eligibility of unicompartmental knee arthroplasties. J Arthroplast. 2011;26(8):1396-1403. doi: 10.1016/j.arth.2010.12.023.
- Willis-Owen CA, Brust K, Alsop H, Miraldo M, Cobb JP. Unicondylar knee arthroplasty in the UK National Health Service: an analysis of candidacy, outcome and cost efficacy. Knee. 2009;16(6):473-478. doi: 10.1016/j.knee.2009.04.006.
- Murray DW, Liddle AD, Dodd CA, Pandit H. Unicompartmental knee arthroplasty: is the glass half full or half empty? Bone Joint J. 2015;97-B(10 Suppl. A):3-8. doi: 10.1302/0301-620X.97B10.36542.
- Liddle AD, Judge A, Pandit H, Murray DW. Adverse outcomes after total and unicompartmental knee replacement in 101,330 matched patients: a study of data from the National Joint Registry for England and Wales. Lancet. 2014;384(9952):1437-1445. doi: 10.1016/S0140-6736(14)60419-0.
- Riddle DL, Jiranek WA, McGlynn FJ. Yearly incidence of unicompartmental knee arthroplasty in the United States. J Arthroplast. 2008;23(3):408-412. doi: 10.1016/j.arth.2007.04.012.
- Argenson JN, Blanc G, Aubaniac JM, Parratte S. Modern unicompartmental knee arthroplasty with cement: a concise follow-up, at a mean of twenty years, of a previous report. J Bone Joint Surg, (Am). 2013;95(10):905-909. doi: 10.2106/JBJS.L.00963.
- Koskinen E, Eskelinen A, Paavolainen P, Pulkkinen P, Remes V. Comparison of survival and cost-effectiveness between unicondylar arthroplasty and total knee arthroplasty in patients with primary osteoarthritis: a follow-up study of 50,493 knee replacements from the Finnish Arthroplasty Register. Acta Orthop. 2008;79(4):499-507. doi: 10.1080/17453670710015490.
- Knutson K, Lewold S, Robertsson O, Lidgren L. The Swedish knee arthroplasty register. A nation-wide study of 30,003 knees 1976-1992. Acta Orthop Scand. 1994;65(4):375-386. doi: 10.3109/17453679408995475.
- Kozinn SC, Scott R. Unicondylar knee arthroplasty. J Bone Joint Surg, (Am). 1989;71(1):145-150. doi: 10.2106/00004623-198971010-00023.
- Pennington DW. Unicompartmental knee arthroplasty in patients sixty years of age or younger. J Bone Joint Surg, (Am). 2003;85-A(10):1968-1973. doi: 10.2106/00004623-200310000-00016.
- Biswas D, Van Thiel GS, Wetters NG, Pack BJ, Berger RA, Della Valle CJ. Medial unicompartmental knee arthroplasty in patients less than 55 years old: minimum of two years of follow-up. J Arthroplast. 2014;29(1):101-105. doi: 10.1016/j.arth.2013.04.046.
- Murray DW, Pandit H, Weston-Simons JS, et al. Does body mass index affect the outcome of unicompartmental knee replacement? Knee. 2013;20(6):461-465. doi: 10.1016/j.knee.2012.09.017.
- Kang SN, Smith TO, Sprenger De Rover WB, Walton NP. Pre-operative patellofemoral degenerative changes do not affect the outcome after medial Oxford unicompartmental knee replacement: a report from an independent centre. J Bone Joint Surg Br. 2011;93(4):476-478. doi: 10.1302/0301-620X.93B4.25562.
- Pandit H, Jenkins C, Gill HS, et al. Unnecessary contraindications for mobile-bearing unicompartmental knee replacement. J Bone Joint Surg Br. 2011;93(5):622-628. doi: 10.1302/0301-620X.93B5.26214.
- Kurtz S, Mowat F, Ong K, Chan N, Lau E, Halpern M. Prevalence of primary and revision total hip and knee arthroplasty in the United States from 1990 through 2002. J Bone Joint Surg Am. 2005;87(7):1487-1497. doi: 10.2106/JBJS.D.02441.
- Kurtz SM, Ong KL, Schmier J, et al. Future clinical and economic impact of revision total hip and knee arthroplasty. J Bone Joint Surg, (Am). 2007;89(Suppl. 3):144-151. doi: 10.2106/JBJS.G.00587.
- Day JS, Lau E, Ong KL, Williams GR, Ramsey ML, Kurtz SM. Prevalence and projections of total shoulder and elbow arthroplasty in the United States to 2015. J Shoulder Elbow Surg. 2010;19(8):1115-1120. doi: 10.1016/j.jse.2010.02.009.
- Kurtz S, Ong K, Lau E, Mowat F, Halpern M. Projections of primary and revision hip and knee arthroplasty in the United States from 2005 to 2030. J Bone Joint Surg, (Am). 2007;89(4):780-785. doi: 10.2106/JBJS.F.00222.
- Kamath AF, Ong KL, Lau E, et al. Quantifying the burden of revision total joint arthroplasty for periprosthetic infection. J Arthroplast. 2015;30(9):1492-1497. doi: 10.1016/j.arth.2015.03.035.
- Bolognesi MP, Greiner MA, Attarian DE, et al. Unicompartmental knee arthroplasty and total knee arthroplasty among Medicare beneficiaries, 2000 to 2009. J Bone Joint Surg, (Am). 2013;95(22):e174. doi: 10.2106/JBJS.L.00652.
- Charlson ME, Pompei P, Ales KL, MacKenzie CR. A new method of classifying prognostic comorbidity in longitudinal studies: development and validation. J Chronic Dis. 1987;40(5):373-383. doi: 10.1016/0021-9681(87)90171-8.
- Kurtz SM, Ong KL, Lau E, Bozic KJ. Impact of the economic downturn on total joint replacement demand in the United States: updated projections to 2021. J Bone Joint Surg, (Am). 2014;96(8):624-630. doi: 10.2106/JBJS.M.00285.
- Leskinen J, Eskelinen A, Huhtala H, Paavolainen P, Remes V. The incidence of knee arthroplasty for primary osteoarthritis grows rapidly among baby boomers: a population-based study in Finland. Arthritis Rheum. 2012;64(2):423-428. doi: 10.1002/art.33367.
- Cartier P, Sanouiller JL, Grelsamer RP. Unicompartmental knee arthroplasty surgery. 10-year minimum follow-up period. J Arthroplast. 1996;11(7):782-788. doi: 10.1016/S0883-5403(96)80177-X.
- Kurtz SM, Lau E, Ong K, Zhao K, Kelly M, Bozic KJ. Future young patient demand for primary and revision joint replacement: national projections from 2010 to 2030. Clin Orthop Relat Res. 2009;467(10):2606-2612. doi: 10.1007/s11999-009-0834-6.
- Singh JA, Lu X, Rosenthal GE, Ibrahim S, Cram P. Racial disparities in knee and hip total joint arthroplasty: an 18-year analysis of national Medicare data. Ann Rheum Dis. 2014;73(12):2107-2115. doi: 10.1136/annrheumdis-2013-203494.
- Pierce TP, Elmallah RK, Lavernia CJ, et al. Racial disparities in lower extremity arthroplasty outcomes and use. Orthopedics. 2015;38(12): e1139-e1146. doi: 10.3928/01477447-20151123-05.
- Irgit K, Nelson CL. Defining racial and ethnic disparities in THA and TKA. Clin Orthop Relat Res. 2011;469(7):1817-1823. doi: 10.1007/s11999-011-1885-z.
TAKE-HOME POINTS
- Prior publications on prevalence of unicondylar knee arthroplasty (UKA) in the United States using a single database may have underestimated the “true” number of cases performed.
- For the time periods analyzed, a total of 5,235 and 23,310 UKA procedures were identified from the 5% Medicare and MarketScan databases, respectively.
- Rates of UKA generally increased until 2008, after which there was a decline through 2012.
- Gender and year of operation were found to be significantly associated with UKA rate.
- Males ages 55-64, 65-69, and 70-74 were the only age-gender groups whose UKA rates appear to be trending upward.
Fragility Fractures: Diagnosis and Treatment
ABSTRACT
Fragility fractures are estimated to affect 3 million people annually in the United States. As they are associated with a significant mortality rate, the prevention of these fractures should be a priority for orthopedists. At-risk patients include the elderly and those with thyroid disease, diabetes, hypertension, and heart disease. Osteoporosis is diagnosed by the presence of a fragility fracture or by dual-energy x-ray absorptiometry (DXA) in the absence of a fragility fracture. In 2011, the United States Preventive Services Task Force (USPSTF) recommended that all women ≥65 years should be screened for osteoporosis by DXA. Women <65 years with a 10-year fracture risk =/> than that of a 65-year-old white woman should also be screened for osteoporosis. Lifestyle changes, such as calcium and vitamin D supplementation, exercise, and smoking cessation, are non-pharmacologic treatment options. The National Osteoporosis Foundation recommends treating osteoporosis with pharmacotherapy in patients with a high risk for fracture (T score <–2.5) or history of fragility fracture. Understanding risk factors and eliminating medications known to cause decreased BMD are vital to prevention and will be necessary to limit these fractures and their associated expenses in the future.
Continue to: Fragility fractures are caused by...
Fragility fractures are caused by falls from standing height or repetitive physiological loads.1 With the growing aging population in the United States, it is estimated that 3 million people will be affected by fragility fractures yearly.2 In the setting of osseous insufficiency, fractures that are typically associated with high-energy trauma are encountered in patients who simply trip over a parking lot curb or fall off their bike. After surgery, the severe disruption of patients’ lives continues with a prolonged rehabilitation period.
Fragility fractures are not only traumatizing for patients; they are also associated with significantly increased mortality. A study by Gosch and colleagues found that 70.6% of patients died during the normal follow-up period, and 29.4% of patients died within the first year of suffering a fracture.3 Also, the mean life expectancy post-fragility fracture was only 527 days.3 Diagnosis and treatment of osteoporosis is imperative to prevent fragility fractures before they occur.
RISK FACTORS AND CAUSES
The incidence of fragility fractures increases in patients with comorbidities such as thyroid disease, diabetes, hypertension, and heart disease.4 Hyperthyroidism and treated hypothyroidism cause an imbalance between osteoblast and osteoclast activity, resulting in osteoporosis.5 A thyroid-stimulating hormone level < 0.1 increases the risk of vertebral and non-vertebral fractures by a factor of 4.5 and 3.2 mIU/L respectively.4 Patients with diabetes also have an increased risk of fragility fractures, which is due to impaired healing capabilities, especially that of bone healing. Approximately 2 million people are affected by type 1 diabetes in the United States, and 20% of those patients will develop osteoporosis.6
Hypertension and osteoporosis are 2 diseases that occur often in the elderly. Common etiological factors believed to cause both hypertension and osteoporosis are low calcium intake, high consumption of salt, and vitamin D and vitamin K deficiency. Also, hypertension treated with loop diuretics has been found to cause negative effects on bone and increase the risk of osteoporosis.7 The only antihypertensive medications that preserve bone mineral density (BMD) and reduce fracture risk are thiazide diuretics.7 Lastly, an association between coronary artery disease and osteoporosis has been hypothesized. The link is not completely understood, but it is believed that oxidative stress and inflammation are the culprits in both diseases.8 In contrast to previous hypotheses, Sosa and colleagues found an independent association between beta blockers and fragility fractures.9 The idea that beta blockers and fragility fractures are linked is still controversial and needs more study. Unlike beta blockers, statins provide a protective effect on bone. They increase BMD and reduce fracture risk by inhibiting osteoclastogenesis.10
In addition to loop diuretics and beta blockers, inhaled glucocorticoids, oral glucocorticoids, proton pump inhibitors (PPIs), H2 receptor antagonists, and anticonvulsants decrease bone density and increase the incidence of fragility fractures.11 Chronic glucocorticoid therapy is the most common cause of secondary osteoporosis. Osteoblasts and osteocytes undergo apoptosis in the presence of glucocorticoids.12 Patients on glucocorticoid therapy have an increased risk of fracture, even with higher BMD values.13 Bone changes that occur while a patient is taking glucocorticoids may not be detected during BMD testing. Therefore, a high level of suspicion of osteoporosis in patients on long-term glucocorticoids is imperative.
Proton pump inhibitors are among the most prescribed medications in the world; they reduce bone resorption, increasing the risk of fracture.14 Proton pump inhibitors and H2 receptor antagonists are hypothesized to cause malabsorption of calcium and indirectly cause osteoporosis. The risk of osteoporosis increases with the length of PPI treatment.15 However, exposure lasting <7 years does not increase the risk of fracture.16 It is recommended that patients on long-term PPIs be referred for BMD testing.
An association between anticonvulsants and osteoporosis has been found in observational studies. The mechanism of this association is not yet fully understood, but it is believed that exacerbation of vitamin D deficiency leads to increased bone metabolism.17 Gastrointestinal (GI) calcium absorption also decreases with anticonvulsant use. Prolonged antiepileptic therapy and high-dose therapy rapidly decrease BMD. Primidone, carbamazepine, phenobarbital, and phenytoin are the drugs most often associated with decreased BMD. Osteoporosis and fragility fracture in these patients can be prevented with calcium, vitamin D, and the bisphosphonate risedronate. These medications have been shown to improve BMD by 69%.18
Continue to: DIAGNOSIS...
DIAGNOSIS
Osteoporosis is diagnosed by the presence of a fragility fracture or by dual-energy x-ray absorptiometry (DXA) in the absence of a fragility fracture.19 Measurements of the femoral neck by DXA are used to diagnose osteoporosis, although DXA can also be used to measure the bone density of the spine and peripheral skeleton.20
The World Health Organization developed a set of T score criteria to diagnose osteoporosis in postmenopausal women (Table 1). A T score >-1 is normal, <-1 but >-2.5 signifies osteopenia, <-2.5 is osteoporosis, and <-2.5 with fragility fracture is severe osteoporosis.19 The Z score, not the T score, should be used to assess osteoporosis in premenopausal women, men <50 years, and children (Table 2). The Z score is calculated by comparing the patient’s BMD with the mean BMD of their peers of a similar age, race, and gender.19 Z scores <-2.0 indicate low BMD for chronological age. A Z score > -2.0 is considered within the expected range for age.20 Bone mineral density testing is the rate- limiting step to starting osteoporosis treatment.21 Without testing, treatment of osteoporosis is very unlikely.
Table 1. T Score Criteria
T score | Diagnosis |
> -1.0 | Normal |
-1.0 to -2.5 | Osteopenia |
< -2.5 | Osteoporosis |
< -2.5 with fragility fracture | Severe osteoporosis |
Table 2. Z Score Criteria
Z score | Diagnosis |
> -2.0 | Normal BMD for age |
< -2.0 | Low BMD for age |
The World Health Organization also developed a tool to predict fracture risk. The Fracture Risk Assessment Tool uses fracture history in addition to other risk factors to predict a patient’s 10-year risk of major fracture.22 Risk factors used to assess fracture risk include age, sex, weight, height, previous fracture, parental hip fracture history, current smoker, glucocorticoid use, rheumatoid arthritis, secondary osteoporosis, excessive alcohol use, and femoral neck BMD.
In 2011, the United States Preventive Services Task Force (USPSTF) recommended that all women ≥65 years should be screened for osteoporosis by DXA. Women <65 years with a 10-year fracture risk =/> than that of a 65-year-old white woman should also be screened for osteoporosis. These recommendations are different for men. It was concluded that the evidence was insufficient to support osteoporosis screening in men.23 As of April 2017, Centers for Medicare and Medicaid Services current reimbursement rates for DXA scans are, on average, $123.10 in the hospital setting and $41.63 in the office setting. The axial DXA CPT code is 77080.
Continue to: TREATMENT...
TREATMENT
NONPHARMACOLOGIC
Patients with mild osteoporosis may be treated first non-pharmacologically. Lifestyle changes such as calcium and vitamin D supplementation, exercise, and smoking cessation are non-pharmacologic treatment options. Calcium carbonate and calcium citrate are common supplements. Calcium carbonate is 40% elemental calcium, whereas calcium citrate supplements are only 21% elemental calcium. Calcium supplements are best absorbed when taken with food.24 The recommended daily total calcium intake is 1200 mg.25 Only 500 to 600 milligrams of calcium can be absorbed by the GI tract at a time. Therefore, calcium supplements should be taken at least 4 to 5 hours apart.24Patients should also be counseled that calcium supplements may cause GI side effects such as bloating and constipation. To reduce side effects, patients can slowly increase the dose of calcium to a therapeutic level.
Vitamin D supplementation works best in conjunction with calcium supplementation. Vitamin D functions to regulate calcium absorption in the intestine and stimulate bone resorption and maintain the serum calcium concentration. The National Osteoporosis Foundation recommends 800 to 1000 international units of vitamin D daily.24 Lifestyle changes may be sufficient to stop the progression of osteoporosis in its early stages. Once osteoporosis becomes severe enough, pharmacotherapy is needed to stop further bone destruction and improve BMD.
PHARMACOLOGIC
After an initial fragility fracture, the risk of additional ones increases significantly, making treatment of osteoporosis essential. The National Osteoporosis Foundation recommends treating osteoporosis with pharmacotherapy in patients with a high risk of fracture (T score <-2.5) or history of fragility fracture.26 Bisphosphonates inhibit bone resorption and are considered the first-line therapy for postmenopausal women with osteoporosis. A common side effect of oral bisphosphonates is GI toxicity. Patients are advised to avoid lying down for at least 30 minutes after medication administration to avoid esophageal irritation. Oral bisphosphonates should also be taken in the morning on an empty stomach with at least 8 ounces of water. Recurrent bisphosphonate use should be avoided in patients with chronic kidney disease. Oral alendronate and risedronate are typically discontinued after 5 years of use.27 Long-term bisphosphonate use may cause an increased risk of fragility fracture due to oversuppression of bone turnover. To avoid this risk, bisphosphonate “drug holidays” are an option. Bisphosphonates accumulate over time, creating reservoirs. Even after therapy is stopped, patients continue to have therapeutic effects for 2 to 5 years.28
Bisphosphonates are available in both oral and intravenous forms. Alendronate is available in doses of 10 mg and 70 mg for daily and weekly administration, respectively. Both are available in tablet form, but the 70 mg weekly dose is also available in a dissolvable formulation. Alendronate is available in a reduced dose for osteoporosis prevention. Alendronate dosing for osteoporosis prevention is 5 mg daily or 35 mg weekly. Risedronate is dosed as 5 mg daily, 35 mg weekly, or 150 mg monthly. Intravenous bisphosphonates are indicated when oral bisphosphonates are not tolerated, only after vitamin D has been assessed and is within the normal range. Zoledronic acid is administered as a 15-minute infusion once a year.
Teriparatide (Forteo; PTH-1-34) is available for glucocorticoid-induced osteoporosis, postmenopausal women, and men with severe osteoporosis. It is indicated for patients in whom bisphosphonate treatment has failed or those who do not tolerate bisphosphonates. Teriparatide is a synthetic parathyroid hormone (PTH) that acts as an anabolic agent, stimulating bone formation, maturation, and remodeling.29 In addition to its application as a bone-building hormone, teriparatide has gained popularity for various off-label uses. These include accelerated osteosynthesis, stress fracture healing, and in the nonoperative treatment of osteoarthritis.29 Parathyroid hormone has been shown to stimulate the maturation, proliferation, and maintenance of osteoblast progenitor cells. More recently, PTH has been shown to regulate chondrocyte signaling, as well as differentiation and maturation. Further study on the chondroregenerative potential of PTH has demonstrated its efficacy as a novel disease-modifying agent in the treatment of osteoarthritis.29 Teriparatide is administered as a daily subcutaneous injection. The United States dosing is 600 mcg/2.4 mL. Adverse effects such as orthostatic hypotension and osteosarcoma may occur. BMD testing should be performed 1 to 2 years after initiation of teriparatide and every 2 years thereafter.26
Abaloparatide (Tymlos), a human parathyroid hormone, is another treatment option for postmenopausal women at risk of osteoporotic fracture. In a study comparing the efficacy of abaloparatide and teriparatide, treatment with abaloparatide was found to induce higher BMD levels in a time frame of 12 months. The BMD differences could be attributed to many factors, such as an enhanced net anabolic effect or a reduced osteoblast expression. Furthermore, the risk of developing new vertebral and nonvertebral fractures decreased in the abaloparatide group compared with the placebo group over a period of 18 months.30
Continue to: The recommended daily dose for abaloparatide...
The recommended daily dose for abaloparatide is 80 mcg via subcutaneous injection with calcium and vitamin D supplements.31 Adverse reactions were consistent between abaloparatide and teriparatide, and included hypercalcemia, hypercalciuria, and orthostatic hypotension.30 The use of parathyroid analogs for >2 years is not recommended due to the risk of osteosarcoma.
Denosumab (Prolia) is a monoclonal antibody that stops osteoclastogenesis by blocking the binding of RANKL to RANK.31 It is indicated for patients intolerant to bisphosphonates or with impaired kidney function. Prolia is administered subcutaneously in 60 mg doses every 6 months in men and postmenopausal women with osteoporosis. Prolia is contraindicated in patients with hypersensitivity to any component of the medication, pregnancy, and hypocalcemia.
Selective estrogen receptor modulators (SERMs), such as raloxifene and tamoxifen, can treat osteoporosis effectively in postmenopausal women. Raloxifene is considered the SERM of choice due to the availability of more robust safety and efficacy data. Raloxifene increases BMD while decreasing bone resorption and bone turnover.32 It is also used to reduce breast cancer risk; however, it increases the risk of thromboembolic events and hot flashes. Tamoxifen is not typically used to treat osteoporosis, but women treated for breast cancer with tamoxifen receive some bone protection.
Lastly, calcitonin and strontium ranelate are also options to treat osteoporosis. However, both calcitonin and strontium ranelate have weak effects on BMD. Calcitonin only transiently inhibits osteoclast activity.33 Therefore, medications like bisphosphonates, teriparatide, denosumab, and SERMs are preferred.
A summary of medications used to treat osteoporosis can be found in Table 3.
Table 3. Overview of Common Medications Used in the Treatment and Prevention of Osteoporosis
Medication | Indication | Dosing |
Calcium supplementation | Mild osteoporosis | 1200 mg oral/d |
Vitamin D supplementation | Mild osteoporosis | 800 to 1000 IU oral/d |
Alendronate | Postmenopausal osteoporosis
Osteoporosis prevention | 10 mg oral/d 70 mg oral/wk
5 mg/d 35 mg/wk |
Risedronate | Postmenopausal osteoporosis | 5 mg oral/d 35 mg oral/wk 150 mg oral/mo |
Teriparatide (Forteo) | Glucocorticoid-inducted osteoporosis, postmenopausal osteoporosis, men with severe osteoporosis | 600 mcg/2.4 mL subcutaneous/d |
Abaloparatide (Tymlos) | Postmenopausal osteoporosis | 80 mcg subcutaneous/d |
Denosumab (Prolia) | Patients intolerant to bisphosphonates; patients with impaired kidney function. | 60 mg subcutaneous every 6 mo |
Raloxifene | Postmenopausal osteoporosis | 60 mg oral/d |
Tamoxifen | Postmenopausal osteoporosis | 20 mg oral/d |
Calcitonin | Postmenopausal osteoporosis | 100 units intramuscular or subcutaneous/d 200 units (1 spray) intranasal/d |
Strontium ranelate | Postmenopausal osteoporosis Severe osteoporosis in men | 2 g/d dissolved in water, prior to bedtime Not recommended in CrCl <30 mL/min |
Abbreviation: CrCl, creatinine clearance.
CONCLUSION
With a growing aging population, the prevalence of osteoporosis is expected to increase. By 2025, experts estimate that there will be 2 million fractures yearly, costing the United States upwards of $25 billion.34,35 This estimate does not include the cost of lost productivity or disability, which will likely cost billions more.34,35 Understanding risk factors and eliminating medications known to cause decreased BMD are vital. Obtaining a BMD measurement is the rate-limiting step for treatment initiation. Without an appropriate diagnosis, treatment is unlikely. As providers, it us our responsibility to maintain a high level of suspicion of osteoporosis in the elderly and promptly diagnose and treat them.
- Dietz SO, Hofmann A, Rommens PM. Haemorrhage in fragility fractures of the pelvis. Eur J Trauma Emerg Surg. 2015;41:363-367. doi: 10.1007/s00068-014-0452-1
- Burge R, Dawson-Hughes B, Solomon DH, Wong JB, King A, Tosteson A. Incidence and economic burden of osteoporosis-related fractures in the United States, 2005-2025. J Bone Miner Res. 2007;22(3):465-475. doi: 10.1359/jbmr.061113.
- Gosch M, Hoffmann-Weltin Y, Roth T, Blauth M, Nicholas JA, Kammerlander C. Orthogeriatric co-management improves the outcome of long-term care residents with fragility fractures. Arch Orthop Trauma Surg. 2016; 136(10):1403-1409. doi: 10.1007/s00402-016-2543-4.
- Maccagnano G, Notarnicola A, Pesce V, Mudoni S, Tafuri S, Moretti B. The prevalence of fragility fractures in a population of a region of southern Italy affected by thyroid disorders. BioMed Res Int. 2016. doi: 10.1155/2016/6017165.
- Mosekilde L, Eriksen EF, Charles P. Effects of thyroid hormones on bone and mineral metabolism. Endocrinol Metab Clin North Am. 1990;19(1):35-63. doi: 10.1016/S0889-8529(18)30338-4.
- Liporace FA, Breitbart EA, Yoon RS, Doyle E, Paglia DM, Lin S. The effect of locally delivered recombinant human bone morphogenic protein-2 with hydroxyapatite/tri-calcium phosphate on the biomechanical properties of bone in diabetes-related osteoporosis. J Orthop Traumatol.2015;16(2):151-159. doi: 10.1007/s10195-014-0327-6.
- Ilic K, Obradovic N, Vujasinovic-Stupar N. The relationship among hypertension, antihypertensive medications, and osteoporosis: a narrative review. Calcif. Tissue Int. 2013;92(3):217-227. doi: 10.1007/s00223-012-9671-9.
- Yesil Y, Ulger, Z, Halil M, et al. Coexistence of osteoporosis (OP) and coronary artery disease (CAD) in the elderly: it is not just a by chance event. Arch Gerontol Geriatr. 2012;54(3):473-476. doi: 10.1016/j.archger.2011.06.007.
- Sosa M, Saavedra P, de Tejada MJG, et al, GIUMO Cooperative Group. Beta-blocker use is associated with fragility fractures in postmenopausal women with coronary heart disease. Aging Clin Exp Res.2011;23(3):112-117. doi: 10.3275/7041.
- An T, Hao J, Li R, Yang M, Cheng G, Zou M. Efficacy of statins for osteoporosis: a systematic review and met-analysis. Osteoporos Int. 2017;28(1):47-57. doi: 10.1007/s00198-016-3844-8.
- Munson JC, Bynum JP, Bell J, et al. Patterns of prescription drug use before and after fragility fracture. JAMA Intern Med. 2016;176(10):1531-1538. doi: 10.1001/jamainternmed.2016.4814.
- Saag KG, Agnesdei D, Hans D, et al. Trabecular bone score in patients with chronic glucocorticoid therapy-induced osteoporosis treated with alendronate or teriparatide. Arthritis Rheumatol. 2016;68(9):2122-2128. doi: 10.1002/art.39726.
- Chuang MH, Chuang TL, Koo M, Wang YF. Trabecular bone score reflects trabecular microarchitecture deterioration and fragility fracture in female adult patients receiving glucocorticoid therapy: A pre-post controlled study. BioMed Res Int. 2017. doi: 10.1155/2017/4210217.
- Andersen BN, Johansen PB, Abrahamsen B. Proton pump inhibitors and osteoporosis. Curr Opin Rheumatol. 2016;28(4):420-425. doi: 10.1097/BOR.0000000000000291.
- Jacob L, Hadji P, Kostev K. The use of proton pump inhibitors is positively associated with osteoporosis in postmenopausal women in Germany. Climacteric. 2016; 19(5):478-481. doi: 10.1080/13697137.2016.1200549.
- Targownik LE, Lix LM, Metge CJ, Prior HJ, Leung S, Leslie WD. Use of proton pump inhibitors and risk of osteoporosis-related fracture. Can Med Assoc J. 2008;179:319-326. doi: 10.1503/cmaj.071330.
- Lee RH, Lyles KH, Colon-Emeric C. A review of the effect of anticonvulsant medications on bone mineral density and fracture risk. Am J Geriatr Pharmacother. 2010;8(1):34-46. doi: 10.1016/j.amjopharm.2010.02.003.
- Arora E, Singh H, Gupta YK. Impact of antiepileptic drugs on bone health: Need for monitoring, treatment, and prevention. J Family Med Prim Care. 2016;5(2):248-253. doi: 10.4103/2249-4863.192338.
- Maghraoui AE, Roux C. DXA scanning in clinical practice. Q J Med. 2008;101(8):605-617. doi: 10.1093/qjmed/hcn022.
- Watts NB, Lewiecki EM, Miller PD, Baim S. National osteoporosis foundation 2008 clinician’s guide to prevention and treatment of osteoporosis and the world health organization fracture risk assessment tool (FRAX): What they mean to the bone densiometrist and bone technologist. J Clin Densitom. 2008;11(4):473-477. doi: 10.1016/j.jocd.2008.04.003.
- MacLean C, Newberry S, Maglione M, et al. Systematic review: comparative effectiveness of treatments to prevent fractures in men and women with low bone density or osteoporosis. Ann Intern Med. 2007;148(3):197-213. doi: 10.7326/0003-4819-148-3-200802050-00198.
- Beaton DE, Vidmar M, Pitzul KB, et al. Addition of a fracture risk assessment to a coordinator’s role improved treatment rates within 6 months of screening in a fragility fracture screening program. J Am Geriatr Soc. 2017; 28(3):863-869. doi: 10.1007/s00198-016-3794-1.
- U.S. Preventative Services Task Force. Screening for osteoporosis. Ann Intern Med. 2011;154(5):356-364. doi: 10.7326/0003-4819-154-5-201103010-00307.
- Sunyecz JA. The use of calcium and vitamin D in the management of osteoporosis. Ther Clin Risk Manag. 2008;4(4):827-836.
- Eastell, R. (1998). Treatment of postmenopausal osteoporosis. N Engl J Med. 1998;338:736-746. doi: 10.1056/NEJM199803123381107.
- Cosman F, de Beur SJ, LeBoff MS, et al, National Osteoporosis Foundation. Clinician’s guide to prevention and treatment of osteoporosis. Osteoporos Int. 2014;25(10):2359-2381. doi: 10.1007/s00198-014-2794-2.
- Black DM, Schartz AV, Ensrud KE, et al, doi:10.1001/jama.296.24.2927.
- Schmidt GA, Horner KE, McDanel DL, Ross MB, Moores KG. Risks and benefits of long-term bisphosphonate therapy. Am J Health Syst Pharm. 2010;67(12):994-1001. doi: 10.2146/ajhp090506.
- Kraenzlin, ME, Meier C. Parathyroid hormone analogues in the treatment of osteoporosis. Nat Rev Endocrinol. 2011;7(11):647-656. doi: 10.1038/nrendo.2011.108.
- Miller P, Hattersley G, Riis B, et al. Effect of abaloparatide vs placebo on new vertebral fractures in postmenopausal women with osteoporosis. JAMA. 2016;316(7):722-733. doi: 10.1001/jama.2016.11136.
- TYMLOSTM [prescribing information]. Waltham, MA: Radius Health, Inc; 2017.
- Tetsunaga T, Tetsunaga T, Nishida K, et al. Denosumab and alendronate treatment in patients with back pain due to fresh osteoporotic vertebral fractures. J Orthop Sci. 2017;22(2):230-236. doi: 10.1016/j.jos.2016.11.017.
- Recker, RR, Mitlak BH, Ni X, Krege JH. Long-term raloxifene for postmenopausal osteoporosis. Curr Med Res Opin. 2011;27(9):1755-1761. doi: 10.1185/03007995.2011.606312.
- Yildirim K, Gureser G, Karatay S, et al. Comparison of the effects of alendronate, risedronate and calcitonin treatment in postmenopausal osteoporosis. J Back Musculoskelet Rehabil.2005;18(3/4):85-89. doi: 10.3233/BMR-2005-183-405.
- Christensen L, Iqbal S, Macarios D, Badamgarav E, Harley C. Cost of fractures commonly associated with osteoporosis in a managed-care population. J Med Econ. 2010;13(2):302-313. doi: 10.3111/13696998.2010.488969.
ABSTRACT
Fragility fractures are estimated to affect 3 million people annually in the United States. As they are associated with a significant mortality rate, the prevention of these fractures should be a priority for orthopedists. At-risk patients include the elderly and those with thyroid disease, diabetes, hypertension, and heart disease. Osteoporosis is diagnosed by the presence of a fragility fracture or by dual-energy x-ray absorptiometry (DXA) in the absence of a fragility fracture. In 2011, the United States Preventive Services Task Force (USPSTF) recommended that all women ≥65 years should be screened for osteoporosis by DXA. Women <65 years with a 10-year fracture risk =/> than that of a 65-year-old white woman should also be screened for osteoporosis. Lifestyle changes, such as calcium and vitamin D supplementation, exercise, and smoking cessation, are non-pharmacologic treatment options. The National Osteoporosis Foundation recommends treating osteoporosis with pharmacotherapy in patients with a high risk for fracture (T score <–2.5) or history of fragility fracture. Understanding risk factors and eliminating medications known to cause decreased BMD are vital to prevention and will be necessary to limit these fractures and their associated expenses in the future.
Continue to: Fragility fractures are caused by...
Fragility fractures are caused by falls from standing height or repetitive physiological loads.1 With the growing aging population in the United States, it is estimated that 3 million people will be affected by fragility fractures yearly.2 In the setting of osseous insufficiency, fractures that are typically associated with high-energy trauma are encountered in patients who simply trip over a parking lot curb or fall off their bike. After surgery, the severe disruption of patients’ lives continues with a prolonged rehabilitation period.
Fragility fractures are not only traumatizing for patients; they are also associated with significantly increased mortality. A study by Gosch and colleagues found that 70.6% of patients died during the normal follow-up period, and 29.4% of patients died within the first year of suffering a fracture.3 Also, the mean life expectancy post-fragility fracture was only 527 days.3 Diagnosis and treatment of osteoporosis is imperative to prevent fragility fractures before they occur.
RISK FACTORS AND CAUSES
The incidence of fragility fractures increases in patients with comorbidities such as thyroid disease, diabetes, hypertension, and heart disease.4 Hyperthyroidism and treated hypothyroidism cause an imbalance between osteoblast and osteoclast activity, resulting in osteoporosis.5 A thyroid-stimulating hormone level < 0.1 increases the risk of vertebral and non-vertebral fractures by a factor of 4.5 and 3.2 mIU/L respectively.4 Patients with diabetes also have an increased risk of fragility fractures, which is due to impaired healing capabilities, especially that of bone healing. Approximately 2 million people are affected by type 1 diabetes in the United States, and 20% of those patients will develop osteoporosis.6
Hypertension and osteoporosis are 2 diseases that occur often in the elderly. Common etiological factors believed to cause both hypertension and osteoporosis are low calcium intake, high consumption of salt, and vitamin D and vitamin K deficiency. Also, hypertension treated with loop diuretics has been found to cause negative effects on bone and increase the risk of osteoporosis.7 The only antihypertensive medications that preserve bone mineral density (BMD) and reduce fracture risk are thiazide diuretics.7 Lastly, an association between coronary artery disease and osteoporosis has been hypothesized. The link is not completely understood, but it is believed that oxidative stress and inflammation are the culprits in both diseases.8 In contrast to previous hypotheses, Sosa and colleagues found an independent association between beta blockers and fragility fractures.9 The idea that beta blockers and fragility fractures are linked is still controversial and needs more study. Unlike beta blockers, statins provide a protective effect on bone. They increase BMD and reduce fracture risk by inhibiting osteoclastogenesis.10
In addition to loop diuretics and beta blockers, inhaled glucocorticoids, oral glucocorticoids, proton pump inhibitors (PPIs), H2 receptor antagonists, and anticonvulsants decrease bone density and increase the incidence of fragility fractures.11 Chronic glucocorticoid therapy is the most common cause of secondary osteoporosis. Osteoblasts and osteocytes undergo apoptosis in the presence of glucocorticoids.12 Patients on glucocorticoid therapy have an increased risk of fracture, even with higher BMD values.13 Bone changes that occur while a patient is taking glucocorticoids may not be detected during BMD testing. Therefore, a high level of suspicion of osteoporosis in patients on long-term glucocorticoids is imperative.
Proton pump inhibitors are among the most prescribed medications in the world; they reduce bone resorption, increasing the risk of fracture.14 Proton pump inhibitors and H2 receptor antagonists are hypothesized to cause malabsorption of calcium and indirectly cause osteoporosis. The risk of osteoporosis increases with the length of PPI treatment.15 However, exposure lasting <7 years does not increase the risk of fracture.16 It is recommended that patients on long-term PPIs be referred for BMD testing.
An association between anticonvulsants and osteoporosis has been found in observational studies. The mechanism of this association is not yet fully understood, but it is believed that exacerbation of vitamin D deficiency leads to increased bone metabolism.17 Gastrointestinal (GI) calcium absorption also decreases with anticonvulsant use. Prolonged antiepileptic therapy and high-dose therapy rapidly decrease BMD. Primidone, carbamazepine, phenobarbital, and phenytoin are the drugs most often associated with decreased BMD. Osteoporosis and fragility fracture in these patients can be prevented with calcium, vitamin D, and the bisphosphonate risedronate. These medications have been shown to improve BMD by 69%.18
Continue to: DIAGNOSIS...
DIAGNOSIS
Osteoporosis is diagnosed by the presence of a fragility fracture or by dual-energy x-ray absorptiometry (DXA) in the absence of a fragility fracture.19 Measurements of the femoral neck by DXA are used to diagnose osteoporosis, although DXA can also be used to measure the bone density of the spine and peripheral skeleton.20
The World Health Organization developed a set of T score criteria to diagnose osteoporosis in postmenopausal women (Table 1). A T score >-1 is normal, <-1 but >-2.5 signifies osteopenia, <-2.5 is osteoporosis, and <-2.5 with fragility fracture is severe osteoporosis.19 The Z score, not the T score, should be used to assess osteoporosis in premenopausal women, men <50 years, and children (Table 2). The Z score is calculated by comparing the patient’s BMD with the mean BMD of their peers of a similar age, race, and gender.19 Z scores <-2.0 indicate low BMD for chronological age. A Z score > -2.0 is considered within the expected range for age.20 Bone mineral density testing is the rate- limiting step to starting osteoporosis treatment.21 Without testing, treatment of osteoporosis is very unlikely.
Table 1. T Score Criteria
T score | Diagnosis |
> -1.0 | Normal |
-1.0 to -2.5 | Osteopenia |
< -2.5 | Osteoporosis |
< -2.5 with fragility fracture | Severe osteoporosis |
Table 2. Z Score Criteria
Z score | Diagnosis |
> -2.0 | Normal BMD for age |
< -2.0 | Low BMD for age |
The World Health Organization also developed a tool to predict fracture risk. The Fracture Risk Assessment Tool uses fracture history in addition to other risk factors to predict a patient’s 10-year risk of major fracture.22 Risk factors used to assess fracture risk include age, sex, weight, height, previous fracture, parental hip fracture history, current smoker, glucocorticoid use, rheumatoid arthritis, secondary osteoporosis, excessive alcohol use, and femoral neck BMD.
In 2011, the United States Preventive Services Task Force (USPSTF) recommended that all women ≥65 years should be screened for osteoporosis by DXA. Women <65 years with a 10-year fracture risk =/> than that of a 65-year-old white woman should also be screened for osteoporosis. These recommendations are different for men. It was concluded that the evidence was insufficient to support osteoporosis screening in men.23 As of April 2017, Centers for Medicare and Medicaid Services current reimbursement rates for DXA scans are, on average, $123.10 in the hospital setting and $41.63 in the office setting. The axial DXA CPT code is 77080.
Continue to: TREATMENT...
TREATMENT
NONPHARMACOLOGIC
Patients with mild osteoporosis may be treated first non-pharmacologically. Lifestyle changes such as calcium and vitamin D supplementation, exercise, and smoking cessation are non-pharmacologic treatment options. Calcium carbonate and calcium citrate are common supplements. Calcium carbonate is 40% elemental calcium, whereas calcium citrate supplements are only 21% elemental calcium. Calcium supplements are best absorbed when taken with food.24 The recommended daily total calcium intake is 1200 mg.25 Only 500 to 600 milligrams of calcium can be absorbed by the GI tract at a time. Therefore, calcium supplements should be taken at least 4 to 5 hours apart.24Patients should also be counseled that calcium supplements may cause GI side effects such as bloating and constipation. To reduce side effects, patients can slowly increase the dose of calcium to a therapeutic level.
Vitamin D supplementation works best in conjunction with calcium supplementation. Vitamin D functions to regulate calcium absorption in the intestine and stimulate bone resorption and maintain the serum calcium concentration. The National Osteoporosis Foundation recommends 800 to 1000 international units of vitamin D daily.24 Lifestyle changes may be sufficient to stop the progression of osteoporosis in its early stages. Once osteoporosis becomes severe enough, pharmacotherapy is needed to stop further bone destruction and improve BMD.
PHARMACOLOGIC
After an initial fragility fracture, the risk of additional ones increases significantly, making treatment of osteoporosis essential. The National Osteoporosis Foundation recommends treating osteoporosis with pharmacotherapy in patients with a high risk of fracture (T score <-2.5) or history of fragility fracture.26 Bisphosphonates inhibit bone resorption and are considered the first-line therapy for postmenopausal women with osteoporosis. A common side effect of oral bisphosphonates is GI toxicity. Patients are advised to avoid lying down for at least 30 minutes after medication administration to avoid esophageal irritation. Oral bisphosphonates should also be taken in the morning on an empty stomach with at least 8 ounces of water. Recurrent bisphosphonate use should be avoided in patients with chronic kidney disease. Oral alendronate and risedronate are typically discontinued after 5 years of use.27 Long-term bisphosphonate use may cause an increased risk of fragility fracture due to oversuppression of bone turnover. To avoid this risk, bisphosphonate “drug holidays” are an option. Bisphosphonates accumulate over time, creating reservoirs. Even after therapy is stopped, patients continue to have therapeutic effects for 2 to 5 years.28
Bisphosphonates are available in both oral and intravenous forms. Alendronate is available in doses of 10 mg and 70 mg for daily and weekly administration, respectively. Both are available in tablet form, but the 70 mg weekly dose is also available in a dissolvable formulation. Alendronate is available in a reduced dose for osteoporosis prevention. Alendronate dosing for osteoporosis prevention is 5 mg daily or 35 mg weekly. Risedronate is dosed as 5 mg daily, 35 mg weekly, or 150 mg monthly. Intravenous bisphosphonates are indicated when oral bisphosphonates are not tolerated, only after vitamin D has been assessed and is within the normal range. Zoledronic acid is administered as a 15-minute infusion once a year.
Teriparatide (Forteo; PTH-1-34) is available for glucocorticoid-induced osteoporosis, postmenopausal women, and men with severe osteoporosis. It is indicated for patients in whom bisphosphonate treatment has failed or those who do not tolerate bisphosphonates. Teriparatide is a synthetic parathyroid hormone (PTH) that acts as an anabolic agent, stimulating bone formation, maturation, and remodeling.29 In addition to its application as a bone-building hormone, teriparatide has gained popularity for various off-label uses. These include accelerated osteosynthesis, stress fracture healing, and in the nonoperative treatment of osteoarthritis.29 Parathyroid hormone has been shown to stimulate the maturation, proliferation, and maintenance of osteoblast progenitor cells. More recently, PTH has been shown to regulate chondrocyte signaling, as well as differentiation and maturation. Further study on the chondroregenerative potential of PTH has demonstrated its efficacy as a novel disease-modifying agent in the treatment of osteoarthritis.29 Teriparatide is administered as a daily subcutaneous injection. The United States dosing is 600 mcg/2.4 mL. Adverse effects such as orthostatic hypotension and osteosarcoma may occur. BMD testing should be performed 1 to 2 years after initiation of teriparatide and every 2 years thereafter.26
Abaloparatide (Tymlos), a human parathyroid hormone, is another treatment option for postmenopausal women at risk of osteoporotic fracture. In a study comparing the efficacy of abaloparatide and teriparatide, treatment with abaloparatide was found to induce higher BMD levels in a time frame of 12 months. The BMD differences could be attributed to many factors, such as an enhanced net anabolic effect or a reduced osteoblast expression. Furthermore, the risk of developing new vertebral and nonvertebral fractures decreased in the abaloparatide group compared with the placebo group over a period of 18 months.30
Continue to: The recommended daily dose for abaloparatide...
The recommended daily dose for abaloparatide is 80 mcg via subcutaneous injection with calcium and vitamin D supplements.31 Adverse reactions were consistent between abaloparatide and teriparatide, and included hypercalcemia, hypercalciuria, and orthostatic hypotension.30 The use of parathyroid analogs for >2 years is not recommended due to the risk of osteosarcoma.
Denosumab (Prolia) is a monoclonal antibody that stops osteoclastogenesis by blocking the binding of RANKL to RANK.31 It is indicated for patients intolerant to bisphosphonates or with impaired kidney function. Prolia is administered subcutaneously in 60 mg doses every 6 months in men and postmenopausal women with osteoporosis. Prolia is contraindicated in patients with hypersensitivity to any component of the medication, pregnancy, and hypocalcemia.
Selective estrogen receptor modulators (SERMs), such as raloxifene and tamoxifen, can treat osteoporosis effectively in postmenopausal women. Raloxifene is considered the SERM of choice due to the availability of more robust safety and efficacy data. Raloxifene increases BMD while decreasing bone resorption and bone turnover.32 It is also used to reduce breast cancer risk; however, it increases the risk of thromboembolic events and hot flashes. Tamoxifen is not typically used to treat osteoporosis, but women treated for breast cancer with tamoxifen receive some bone protection.
Lastly, calcitonin and strontium ranelate are also options to treat osteoporosis. However, both calcitonin and strontium ranelate have weak effects on BMD. Calcitonin only transiently inhibits osteoclast activity.33 Therefore, medications like bisphosphonates, teriparatide, denosumab, and SERMs are preferred.
A summary of medications used to treat osteoporosis can be found in Table 3.
Table 3. Overview of Common Medications Used in the Treatment and Prevention of Osteoporosis
Medication | Indication | Dosing |
Calcium supplementation | Mild osteoporosis | 1200 mg oral/d |
Vitamin D supplementation | Mild osteoporosis | 800 to 1000 IU oral/d |
Alendronate | Postmenopausal osteoporosis
Osteoporosis prevention | 10 mg oral/d 70 mg oral/wk
5 mg/d 35 mg/wk |
Risedronate | Postmenopausal osteoporosis | 5 mg oral/d 35 mg oral/wk 150 mg oral/mo |
Teriparatide (Forteo) | Glucocorticoid-inducted osteoporosis, postmenopausal osteoporosis, men with severe osteoporosis | 600 mcg/2.4 mL subcutaneous/d |
Abaloparatide (Tymlos) | Postmenopausal osteoporosis | 80 mcg subcutaneous/d |
Denosumab (Prolia) | Patients intolerant to bisphosphonates; patients with impaired kidney function. | 60 mg subcutaneous every 6 mo |
Raloxifene | Postmenopausal osteoporosis | 60 mg oral/d |
Tamoxifen | Postmenopausal osteoporosis | 20 mg oral/d |
Calcitonin | Postmenopausal osteoporosis | 100 units intramuscular or subcutaneous/d 200 units (1 spray) intranasal/d |
Strontium ranelate | Postmenopausal osteoporosis Severe osteoporosis in men | 2 g/d dissolved in water, prior to bedtime Not recommended in CrCl <30 mL/min |
Abbreviation: CrCl, creatinine clearance.
CONCLUSION
With a growing aging population, the prevalence of osteoporosis is expected to increase. By 2025, experts estimate that there will be 2 million fractures yearly, costing the United States upwards of $25 billion.34,35 This estimate does not include the cost of lost productivity or disability, which will likely cost billions more.34,35 Understanding risk factors and eliminating medications known to cause decreased BMD are vital. Obtaining a BMD measurement is the rate-limiting step for treatment initiation. Without an appropriate diagnosis, treatment is unlikely. As providers, it us our responsibility to maintain a high level of suspicion of osteoporosis in the elderly and promptly diagnose and treat them.
ABSTRACT
Fragility fractures are estimated to affect 3 million people annually in the United States. As they are associated with a significant mortality rate, the prevention of these fractures should be a priority for orthopedists. At-risk patients include the elderly and those with thyroid disease, diabetes, hypertension, and heart disease. Osteoporosis is diagnosed by the presence of a fragility fracture or by dual-energy x-ray absorptiometry (DXA) in the absence of a fragility fracture. In 2011, the United States Preventive Services Task Force (USPSTF) recommended that all women ≥65 years should be screened for osteoporosis by DXA. Women <65 years with a 10-year fracture risk =/> than that of a 65-year-old white woman should also be screened for osteoporosis. Lifestyle changes, such as calcium and vitamin D supplementation, exercise, and smoking cessation, are non-pharmacologic treatment options. The National Osteoporosis Foundation recommends treating osteoporosis with pharmacotherapy in patients with a high risk for fracture (T score <–2.5) or history of fragility fracture. Understanding risk factors and eliminating medications known to cause decreased BMD are vital to prevention and will be necessary to limit these fractures and their associated expenses in the future.
Continue to: Fragility fractures are caused by...
Fragility fractures are caused by falls from standing height or repetitive physiological loads.1 With the growing aging population in the United States, it is estimated that 3 million people will be affected by fragility fractures yearly.2 In the setting of osseous insufficiency, fractures that are typically associated with high-energy trauma are encountered in patients who simply trip over a parking lot curb or fall off their bike. After surgery, the severe disruption of patients’ lives continues with a prolonged rehabilitation period.
Fragility fractures are not only traumatizing for patients; they are also associated with significantly increased mortality. A study by Gosch and colleagues found that 70.6% of patients died during the normal follow-up period, and 29.4% of patients died within the first year of suffering a fracture.3 Also, the mean life expectancy post-fragility fracture was only 527 days.3 Diagnosis and treatment of osteoporosis is imperative to prevent fragility fractures before they occur.
RISK FACTORS AND CAUSES
The incidence of fragility fractures increases in patients with comorbidities such as thyroid disease, diabetes, hypertension, and heart disease.4 Hyperthyroidism and treated hypothyroidism cause an imbalance between osteoblast and osteoclast activity, resulting in osteoporosis.5 A thyroid-stimulating hormone level < 0.1 increases the risk of vertebral and non-vertebral fractures by a factor of 4.5 and 3.2 mIU/L respectively.4 Patients with diabetes also have an increased risk of fragility fractures, which is due to impaired healing capabilities, especially that of bone healing. Approximately 2 million people are affected by type 1 diabetes in the United States, and 20% of those patients will develop osteoporosis.6
Hypertension and osteoporosis are 2 diseases that occur often in the elderly. Common etiological factors believed to cause both hypertension and osteoporosis are low calcium intake, high consumption of salt, and vitamin D and vitamin K deficiency. Also, hypertension treated with loop diuretics has been found to cause negative effects on bone and increase the risk of osteoporosis.7 The only antihypertensive medications that preserve bone mineral density (BMD) and reduce fracture risk are thiazide diuretics.7 Lastly, an association between coronary artery disease and osteoporosis has been hypothesized. The link is not completely understood, but it is believed that oxidative stress and inflammation are the culprits in both diseases.8 In contrast to previous hypotheses, Sosa and colleagues found an independent association between beta blockers and fragility fractures.9 The idea that beta blockers and fragility fractures are linked is still controversial and needs more study. Unlike beta blockers, statins provide a protective effect on bone. They increase BMD and reduce fracture risk by inhibiting osteoclastogenesis.10
In addition to loop diuretics and beta blockers, inhaled glucocorticoids, oral glucocorticoids, proton pump inhibitors (PPIs), H2 receptor antagonists, and anticonvulsants decrease bone density and increase the incidence of fragility fractures.11 Chronic glucocorticoid therapy is the most common cause of secondary osteoporosis. Osteoblasts and osteocytes undergo apoptosis in the presence of glucocorticoids.12 Patients on glucocorticoid therapy have an increased risk of fracture, even with higher BMD values.13 Bone changes that occur while a patient is taking glucocorticoids may not be detected during BMD testing. Therefore, a high level of suspicion of osteoporosis in patients on long-term glucocorticoids is imperative.
Proton pump inhibitors are among the most prescribed medications in the world; they reduce bone resorption, increasing the risk of fracture.14 Proton pump inhibitors and H2 receptor antagonists are hypothesized to cause malabsorption of calcium and indirectly cause osteoporosis. The risk of osteoporosis increases with the length of PPI treatment.15 However, exposure lasting <7 years does not increase the risk of fracture.16 It is recommended that patients on long-term PPIs be referred for BMD testing.
An association between anticonvulsants and osteoporosis has been found in observational studies. The mechanism of this association is not yet fully understood, but it is believed that exacerbation of vitamin D deficiency leads to increased bone metabolism.17 Gastrointestinal (GI) calcium absorption also decreases with anticonvulsant use. Prolonged antiepileptic therapy and high-dose therapy rapidly decrease BMD. Primidone, carbamazepine, phenobarbital, and phenytoin are the drugs most often associated with decreased BMD. Osteoporosis and fragility fracture in these patients can be prevented with calcium, vitamin D, and the bisphosphonate risedronate. These medications have been shown to improve BMD by 69%.18
Continue to: DIAGNOSIS...
DIAGNOSIS
Osteoporosis is diagnosed by the presence of a fragility fracture or by dual-energy x-ray absorptiometry (DXA) in the absence of a fragility fracture.19 Measurements of the femoral neck by DXA are used to diagnose osteoporosis, although DXA can also be used to measure the bone density of the spine and peripheral skeleton.20
The World Health Organization developed a set of T score criteria to diagnose osteoporosis in postmenopausal women (Table 1). A T score >-1 is normal, <-1 but >-2.5 signifies osteopenia, <-2.5 is osteoporosis, and <-2.5 with fragility fracture is severe osteoporosis.19 The Z score, not the T score, should be used to assess osteoporosis in premenopausal women, men <50 years, and children (Table 2). The Z score is calculated by comparing the patient’s BMD with the mean BMD of their peers of a similar age, race, and gender.19 Z scores <-2.0 indicate low BMD for chronological age. A Z score > -2.0 is considered within the expected range for age.20 Bone mineral density testing is the rate- limiting step to starting osteoporosis treatment.21 Without testing, treatment of osteoporosis is very unlikely.
Table 1. T Score Criteria
T score | Diagnosis |
> -1.0 | Normal |
-1.0 to -2.5 | Osteopenia |
< -2.5 | Osteoporosis |
< -2.5 with fragility fracture | Severe osteoporosis |
Table 2. Z Score Criteria
Z score | Diagnosis |
> -2.0 | Normal BMD for age |
< -2.0 | Low BMD for age |
The World Health Organization also developed a tool to predict fracture risk. The Fracture Risk Assessment Tool uses fracture history in addition to other risk factors to predict a patient’s 10-year risk of major fracture.22 Risk factors used to assess fracture risk include age, sex, weight, height, previous fracture, parental hip fracture history, current smoker, glucocorticoid use, rheumatoid arthritis, secondary osteoporosis, excessive alcohol use, and femoral neck BMD.
In 2011, the United States Preventive Services Task Force (USPSTF) recommended that all women ≥65 years should be screened for osteoporosis by DXA. Women <65 years with a 10-year fracture risk =/> than that of a 65-year-old white woman should also be screened for osteoporosis. These recommendations are different for men. It was concluded that the evidence was insufficient to support osteoporosis screening in men.23 As of April 2017, Centers for Medicare and Medicaid Services current reimbursement rates for DXA scans are, on average, $123.10 in the hospital setting and $41.63 in the office setting. The axial DXA CPT code is 77080.
Continue to: TREATMENT...
TREATMENT
NONPHARMACOLOGIC
Patients with mild osteoporosis may be treated first non-pharmacologically. Lifestyle changes such as calcium and vitamin D supplementation, exercise, and smoking cessation are non-pharmacologic treatment options. Calcium carbonate and calcium citrate are common supplements. Calcium carbonate is 40% elemental calcium, whereas calcium citrate supplements are only 21% elemental calcium. Calcium supplements are best absorbed when taken with food.24 The recommended daily total calcium intake is 1200 mg.25 Only 500 to 600 milligrams of calcium can be absorbed by the GI tract at a time. Therefore, calcium supplements should be taken at least 4 to 5 hours apart.24Patients should also be counseled that calcium supplements may cause GI side effects such as bloating and constipation. To reduce side effects, patients can slowly increase the dose of calcium to a therapeutic level.
Vitamin D supplementation works best in conjunction with calcium supplementation. Vitamin D functions to regulate calcium absorption in the intestine and stimulate bone resorption and maintain the serum calcium concentration. The National Osteoporosis Foundation recommends 800 to 1000 international units of vitamin D daily.24 Lifestyle changes may be sufficient to stop the progression of osteoporosis in its early stages. Once osteoporosis becomes severe enough, pharmacotherapy is needed to stop further bone destruction and improve BMD.
PHARMACOLOGIC
After an initial fragility fracture, the risk of additional ones increases significantly, making treatment of osteoporosis essential. The National Osteoporosis Foundation recommends treating osteoporosis with pharmacotherapy in patients with a high risk of fracture (T score <-2.5) or history of fragility fracture.26 Bisphosphonates inhibit bone resorption and are considered the first-line therapy for postmenopausal women with osteoporosis. A common side effect of oral bisphosphonates is GI toxicity. Patients are advised to avoid lying down for at least 30 minutes after medication administration to avoid esophageal irritation. Oral bisphosphonates should also be taken in the morning on an empty stomach with at least 8 ounces of water. Recurrent bisphosphonate use should be avoided in patients with chronic kidney disease. Oral alendronate and risedronate are typically discontinued after 5 years of use.27 Long-term bisphosphonate use may cause an increased risk of fragility fracture due to oversuppression of bone turnover. To avoid this risk, bisphosphonate “drug holidays” are an option. Bisphosphonates accumulate over time, creating reservoirs. Even after therapy is stopped, patients continue to have therapeutic effects for 2 to 5 years.28
Bisphosphonates are available in both oral and intravenous forms. Alendronate is available in doses of 10 mg and 70 mg for daily and weekly administration, respectively. Both are available in tablet form, but the 70 mg weekly dose is also available in a dissolvable formulation. Alendronate is available in a reduced dose for osteoporosis prevention. Alendronate dosing for osteoporosis prevention is 5 mg daily or 35 mg weekly. Risedronate is dosed as 5 mg daily, 35 mg weekly, or 150 mg monthly. Intravenous bisphosphonates are indicated when oral bisphosphonates are not tolerated, only after vitamin D has been assessed and is within the normal range. Zoledronic acid is administered as a 15-minute infusion once a year.
Teriparatide (Forteo; PTH-1-34) is available for glucocorticoid-induced osteoporosis, postmenopausal women, and men with severe osteoporosis. It is indicated for patients in whom bisphosphonate treatment has failed or those who do not tolerate bisphosphonates. Teriparatide is a synthetic parathyroid hormone (PTH) that acts as an anabolic agent, stimulating bone formation, maturation, and remodeling.29 In addition to its application as a bone-building hormone, teriparatide has gained popularity for various off-label uses. These include accelerated osteosynthesis, stress fracture healing, and in the nonoperative treatment of osteoarthritis.29 Parathyroid hormone has been shown to stimulate the maturation, proliferation, and maintenance of osteoblast progenitor cells. More recently, PTH has been shown to regulate chondrocyte signaling, as well as differentiation and maturation. Further study on the chondroregenerative potential of PTH has demonstrated its efficacy as a novel disease-modifying agent in the treatment of osteoarthritis.29 Teriparatide is administered as a daily subcutaneous injection. The United States dosing is 600 mcg/2.4 mL. Adverse effects such as orthostatic hypotension and osteosarcoma may occur. BMD testing should be performed 1 to 2 years after initiation of teriparatide and every 2 years thereafter.26
Abaloparatide (Tymlos), a human parathyroid hormone, is another treatment option for postmenopausal women at risk of osteoporotic fracture. In a study comparing the efficacy of abaloparatide and teriparatide, treatment with abaloparatide was found to induce higher BMD levels in a time frame of 12 months. The BMD differences could be attributed to many factors, such as an enhanced net anabolic effect or a reduced osteoblast expression. Furthermore, the risk of developing new vertebral and nonvertebral fractures decreased in the abaloparatide group compared with the placebo group over a period of 18 months.30
Continue to: The recommended daily dose for abaloparatide...
The recommended daily dose for abaloparatide is 80 mcg via subcutaneous injection with calcium and vitamin D supplements.31 Adverse reactions were consistent between abaloparatide and teriparatide, and included hypercalcemia, hypercalciuria, and orthostatic hypotension.30 The use of parathyroid analogs for >2 years is not recommended due to the risk of osteosarcoma.
Denosumab (Prolia) is a monoclonal antibody that stops osteoclastogenesis by blocking the binding of RANKL to RANK.31 It is indicated for patients intolerant to bisphosphonates or with impaired kidney function. Prolia is administered subcutaneously in 60 mg doses every 6 months in men and postmenopausal women with osteoporosis. Prolia is contraindicated in patients with hypersensitivity to any component of the medication, pregnancy, and hypocalcemia.
Selective estrogen receptor modulators (SERMs), such as raloxifene and tamoxifen, can treat osteoporosis effectively in postmenopausal women. Raloxifene is considered the SERM of choice due to the availability of more robust safety and efficacy data. Raloxifene increases BMD while decreasing bone resorption and bone turnover.32 It is also used to reduce breast cancer risk; however, it increases the risk of thromboembolic events and hot flashes. Tamoxifen is not typically used to treat osteoporosis, but women treated for breast cancer with tamoxifen receive some bone protection.
Lastly, calcitonin and strontium ranelate are also options to treat osteoporosis. However, both calcitonin and strontium ranelate have weak effects on BMD. Calcitonin only transiently inhibits osteoclast activity.33 Therefore, medications like bisphosphonates, teriparatide, denosumab, and SERMs are preferred.
A summary of medications used to treat osteoporosis can be found in Table 3.
Table 3. Overview of Common Medications Used in the Treatment and Prevention of Osteoporosis
Medication | Indication | Dosing |
Calcium supplementation | Mild osteoporosis | 1200 mg oral/d |
Vitamin D supplementation | Mild osteoporosis | 800 to 1000 IU oral/d |
Alendronate | Postmenopausal osteoporosis
Osteoporosis prevention | 10 mg oral/d 70 mg oral/wk
5 mg/d 35 mg/wk |
Risedronate | Postmenopausal osteoporosis | 5 mg oral/d 35 mg oral/wk 150 mg oral/mo |
Teriparatide (Forteo) | Glucocorticoid-inducted osteoporosis, postmenopausal osteoporosis, men with severe osteoporosis | 600 mcg/2.4 mL subcutaneous/d |
Abaloparatide (Tymlos) | Postmenopausal osteoporosis | 80 mcg subcutaneous/d |
Denosumab (Prolia) | Patients intolerant to bisphosphonates; patients with impaired kidney function. | 60 mg subcutaneous every 6 mo |
Raloxifene | Postmenopausal osteoporosis | 60 mg oral/d |
Tamoxifen | Postmenopausal osteoporosis | 20 mg oral/d |
Calcitonin | Postmenopausal osteoporosis | 100 units intramuscular or subcutaneous/d 200 units (1 spray) intranasal/d |
Strontium ranelate | Postmenopausal osteoporosis Severe osteoporosis in men | 2 g/d dissolved in water, prior to bedtime Not recommended in CrCl <30 mL/min |
Abbreviation: CrCl, creatinine clearance.
CONCLUSION
With a growing aging population, the prevalence of osteoporosis is expected to increase. By 2025, experts estimate that there will be 2 million fractures yearly, costing the United States upwards of $25 billion.34,35 This estimate does not include the cost of lost productivity or disability, which will likely cost billions more.34,35 Understanding risk factors and eliminating medications known to cause decreased BMD are vital. Obtaining a BMD measurement is the rate-limiting step for treatment initiation. Without an appropriate diagnosis, treatment is unlikely. As providers, it us our responsibility to maintain a high level of suspicion of osteoporosis in the elderly and promptly diagnose and treat them.
- Dietz SO, Hofmann A, Rommens PM. Haemorrhage in fragility fractures of the pelvis. Eur J Trauma Emerg Surg. 2015;41:363-367. doi: 10.1007/s00068-014-0452-1
- Burge R, Dawson-Hughes B, Solomon DH, Wong JB, King A, Tosteson A. Incidence and economic burden of osteoporosis-related fractures in the United States, 2005-2025. J Bone Miner Res. 2007;22(3):465-475. doi: 10.1359/jbmr.061113.
- Gosch M, Hoffmann-Weltin Y, Roth T, Blauth M, Nicholas JA, Kammerlander C. Orthogeriatric co-management improves the outcome of long-term care residents with fragility fractures. Arch Orthop Trauma Surg. 2016; 136(10):1403-1409. doi: 10.1007/s00402-016-2543-4.
- Maccagnano G, Notarnicola A, Pesce V, Mudoni S, Tafuri S, Moretti B. The prevalence of fragility fractures in a population of a region of southern Italy affected by thyroid disorders. BioMed Res Int. 2016. doi: 10.1155/2016/6017165.
- Mosekilde L, Eriksen EF, Charles P. Effects of thyroid hormones on bone and mineral metabolism. Endocrinol Metab Clin North Am. 1990;19(1):35-63. doi: 10.1016/S0889-8529(18)30338-4.
- Liporace FA, Breitbart EA, Yoon RS, Doyle E, Paglia DM, Lin S. The effect of locally delivered recombinant human bone morphogenic protein-2 with hydroxyapatite/tri-calcium phosphate on the biomechanical properties of bone in diabetes-related osteoporosis. J Orthop Traumatol.2015;16(2):151-159. doi: 10.1007/s10195-014-0327-6.
- Ilic K, Obradovic N, Vujasinovic-Stupar N. The relationship among hypertension, antihypertensive medications, and osteoporosis: a narrative review. Calcif. Tissue Int. 2013;92(3):217-227. doi: 10.1007/s00223-012-9671-9.
- Yesil Y, Ulger, Z, Halil M, et al. Coexistence of osteoporosis (OP) and coronary artery disease (CAD) in the elderly: it is not just a by chance event. Arch Gerontol Geriatr. 2012;54(3):473-476. doi: 10.1016/j.archger.2011.06.007.
- Sosa M, Saavedra P, de Tejada MJG, et al, GIUMO Cooperative Group. Beta-blocker use is associated with fragility fractures in postmenopausal women with coronary heart disease. Aging Clin Exp Res.2011;23(3):112-117. doi: 10.3275/7041.
- An T, Hao J, Li R, Yang M, Cheng G, Zou M. Efficacy of statins for osteoporosis: a systematic review and met-analysis. Osteoporos Int. 2017;28(1):47-57. doi: 10.1007/s00198-016-3844-8.
- Munson JC, Bynum JP, Bell J, et al. Patterns of prescription drug use before and after fragility fracture. JAMA Intern Med. 2016;176(10):1531-1538. doi: 10.1001/jamainternmed.2016.4814.
- Saag KG, Agnesdei D, Hans D, et al. Trabecular bone score in patients with chronic glucocorticoid therapy-induced osteoporosis treated with alendronate or teriparatide. Arthritis Rheumatol. 2016;68(9):2122-2128. doi: 10.1002/art.39726.
- Chuang MH, Chuang TL, Koo M, Wang YF. Trabecular bone score reflects trabecular microarchitecture deterioration and fragility fracture in female adult patients receiving glucocorticoid therapy: A pre-post controlled study. BioMed Res Int. 2017. doi: 10.1155/2017/4210217.
- Andersen BN, Johansen PB, Abrahamsen B. Proton pump inhibitors and osteoporosis. Curr Opin Rheumatol. 2016;28(4):420-425. doi: 10.1097/BOR.0000000000000291.
- Jacob L, Hadji P, Kostev K. The use of proton pump inhibitors is positively associated with osteoporosis in postmenopausal women in Germany. Climacteric. 2016; 19(5):478-481. doi: 10.1080/13697137.2016.1200549.
- Targownik LE, Lix LM, Metge CJ, Prior HJ, Leung S, Leslie WD. Use of proton pump inhibitors and risk of osteoporosis-related fracture. Can Med Assoc J. 2008;179:319-326. doi: 10.1503/cmaj.071330.
- Lee RH, Lyles KH, Colon-Emeric C. A review of the effect of anticonvulsant medications on bone mineral density and fracture risk. Am J Geriatr Pharmacother. 2010;8(1):34-46. doi: 10.1016/j.amjopharm.2010.02.003.
- Arora E, Singh H, Gupta YK. Impact of antiepileptic drugs on bone health: Need for monitoring, treatment, and prevention. J Family Med Prim Care. 2016;5(2):248-253. doi: 10.4103/2249-4863.192338.
- Maghraoui AE, Roux C. DXA scanning in clinical practice. Q J Med. 2008;101(8):605-617. doi: 10.1093/qjmed/hcn022.
- Watts NB, Lewiecki EM, Miller PD, Baim S. National osteoporosis foundation 2008 clinician’s guide to prevention and treatment of osteoporosis and the world health organization fracture risk assessment tool (FRAX): What they mean to the bone densiometrist and bone technologist. J Clin Densitom. 2008;11(4):473-477. doi: 10.1016/j.jocd.2008.04.003.
- MacLean C, Newberry S, Maglione M, et al. Systematic review: comparative effectiveness of treatments to prevent fractures in men and women with low bone density or osteoporosis. Ann Intern Med. 2007;148(3):197-213. doi: 10.7326/0003-4819-148-3-200802050-00198.
- Beaton DE, Vidmar M, Pitzul KB, et al. Addition of a fracture risk assessment to a coordinator’s role improved treatment rates within 6 months of screening in a fragility fracture screening program. J Am Geriatr Soc. 2017; 28(3):863-869. doi: 10.1007/s00198-016-3794-1.
- U.S. Preventative Services Task Force. Screening for osteoporosis. Ann Intern Med. 2011;154(5):356-364. doi: 10.7326/0003-4819-154-5-201103010-00307.
- Sunyecz JA. The use of calcium and vitamin D in the management of osteoporosis. Ther Clin Risk Manag. 2008;4(4):827-836.
- Eastell, R. (1998). Treatment of postmenopausal osteoporosis. N Engl J Med. 1998;338:736-746. doi: 10.1056/NEJM199803123381107.
- Cosman F, de Beur SJ, LeBoff MS, et al, National Osteoporosis Foundation. Clinician’s guide to prevention and treatment of osteoporosis. Osteoporos Int. 2014;25(10):2359-2381. doi: 10.1007/s00198-014-2794-2.
- Black DM, Schartz AV, Ensrud KE, et al, doi:10.1001/jama.296.24.2927.
- Schmidt GA, Horner KE, McDanel DL, Ross MB, Moores KG. Risks and benefits of long-term bisphosphonate therapy. Am J Health Syst Pharm. 2010;67(12):994-1001. doi: 10.2146/ajhp090506.
- Kraenzlin, ME, Meier C. Parathyroid hormone analogues in the treatment of osteoporosis. Nat Rev Endocrinol. 2011;7(11):647-656. doi: 10.1038/nrendo.2011.108.
- Miller P, Hattersley G, Riis B, et al. Effect of abaloparatide vs placebo on new vertebral fractures in postmenopausal women with osteoporosis. JAMA. 2016;316(7):722-733. doi: 10.1001/jama.2016.11136.
- TYMLOSTM [prescribing information]. Waltham, MA: Radius Health, Inc; 2017.
- Tetsunaga T, Tetsunaga T, Nishida K, et al. Denosumab and alendronate treatment in patients with back pain due to fresh osteoporotic vertebral fractures. J Orthop Sci. 2017;22(2):230-236. doi: 10.1016/j.jos.2016.11.017.
- Recker, RR, Mitlak BH, Ni X, Krege JH. Long-term raloxifene for postmenopausal osteoporosis. Curr Med Res Opin. 2011;27(9):1755-1761. doi: 10.1185/03007995.2011.606312.
- Yildirim K, Gureser G, Karatay S, et al. Comparison of the effects of alendronate, risedronate and calcitonin treatment in postmenopausal osteoporosis. J Back Musculoskelet Rehabil.2005;18(3/4):85-89. doi: 10.3233/BMR-2005-183-405.
- Christensen L, Iqbal S, Macarios D, Badamgarav E, Harley C. Cost of fractures commonly associated with osteoporosis in a managed-care population. J Med Econ. 2010;13(2):302-313. doi: 10.3111/13696998.2010.488969.
- Dietz SO, Hofmann A, Rommens PM. Haemorrhage in fragility fractures of the pelvis. Eur J Trauma Emerg Surg. 2015;41:363-367. doi: 10.1007/s00068-014-0452-1
- Burge R, Dawson-Hughes B, Solomon DH, Wong JB, King A, Tosteson A. Incidence and economic burden of osteoporosis-related fractures in the United States, 2005-2025. J Bone Miner Res. 2007;22(3):465-475. doi: 10.1359/jbmr.061113.
- Gosch M, Hoffmann-Weltin Y, Roth T, Blauth M, Nicholas JA, Kammerlander C. Orthogeriatric co-management improves the outcome of long-term care residents with fragility fractures. Arch Orthop Trauma Surg. 2016; 136(10):1403-1409. doi: 10.1007/s00402-016-2543-4.
- Maccagnano G, Notarnicola A, Pesce V, Mudoni S, Tafuri S, Moretti B. The prevalence of fragility fractures in a population of a region of southern Italy affected by thyroid disorders. BioMed Res Int. 2016. doi: 10.1155/2016/6017165.
- Mosekilde L, Eriksen EF, Charles P. Effects of thyroid hormones on bone and mineral metabolism. Endocrinol Metab Clin North Am. 1990;19(1):35-63. doi: 10.1016/S0889-8529(18)30338-4.
- Liporace FA, Breitbart EA, Yoon RS, Doyle E, Paglia DM, Lin S. The effect of locally delivered recombinant human bone morphogenic protein-2 with hydroxyapatite/tri-calcium phosphate on the biomechanical properties of bone in diabetes-related osteoporosis. J Orthop Traumatol.2015;16(2):151-159. doi: 10.1007/s10195-014-0327-6.
- Ilic K, Obradovic N, Vujasinovic-Stupar N. The relationship among hypertension, antihypertensive medications, and osteoporosis: a narrative review. Calcif. Tissue Int. 2013;92(3):217-227. doi: 10.1007/s00223-012-9671-9.
- Yesil Y, Ulger, Z, Halil M, et al. Coexistence of osteoporosis (OP) and coronary artery disease (CAD) in the elderly: it is not just a by chance event. Arch Gerontol Geriatr. 2012;54(3):473-476. doi: 10.1016/j.archger.2011.06.007.
- Sosa M, Saavedra P, de Tejada MJG, et al, GIUMO Cooperative Group. Beta-blocker use is associated with fragility fractures in postmenopausal women with coronary heart disease. Aging Clin Exp Res.2011;23(3):112-117. doi: 10.3275/7041.
- An T, Hao J, Li R, Yang M, Cheng G, Zou M. Efficacy of statins for osteoporosis: a systematic review and met-analysis. Osteoporos Int. 2017;28(1):47-57. doi: 10.1007/s00198-016-3844-8.
- Munson JC, Bynum JP, Bell J, et al. Patterns of prescription drug use before and after fragility fracture. JAMA Intern Med. 2016;176(10):1531-1538. doi: 10.1001/jamainternmed.2016.4814.
- Saag KG, Agnesdei D, Hans D, et al. Trabecular bone score in patients with chronic glucocorticoid therapy-induced osteoporosis treated with alendronate or teriparatide. Arthritis Rheumatol. 2016;68(9):2122-2128. doi: 10.1002/art.39726.
- Chuang MH, Chuang TL, Koo M, Wang YF. Trabecular bone score reflects trabecular microarchitecture deterioration and fragility fracture in female adult patients receiving glucocorticoid therapy: A pre-post controlled study. BioMed Res Int. 2017. doi: 10.1155/2017/4210217.
- Andersen BN, Johansen PB, Abrahamsen B. Proton pump inhibitors and osteoporosis. Curr Opin Rheumatol. 2016;28(4):420-425. doi: 10.1097/BOR.0000000000000291.
- Jacob L, Hadji P, Kostev K. The use of proton pump inhibitors is positively associated with osteoporosis in postmenopausal women in Germany. Climacteric. 2016; 19(5):478-481. doi: 10.1080/13697137.2016.1200549.
- Targownik LE, Lix LM, Metge CJ, Prior HJ, Leung S, Leslie WD. Use of proton pump inhibitors and risk of osteoporosis-related fracture. Can Med Assoc J. 2008;179:319-326. doi: 10.1503/cmaj.071330.
- Lee RH, Lyles KH, Colon-Emeric C. A review of the effect of anticonvulsant medications on bone mineral density and fracture risk. Am J Geriatr Pharmacother. 2010;8(1):34-46. doi: 10.1016/j.amjopharm.2010.02.003.
- Arora E, Singh H, Gupta YK. Impact of antiepileptic drugs on bone health: Need for monitoring, treatment, and prevention. J Family Med Prim Care. 2016;5(2):248-253. doi: 10.4103/2249-4863.192338.
- Maghraoui AE, Roux C. DXA scanning in clinical practice. Q J Med. 2008;101(8):605-617. doi: 10.1093/qjmed/hcn022.
- Watts NB, Lewiecki EM, Miller PD, Baim S. National osteoporosis foundation 2008 clinician’s guide to prevention and treatment of osteoporosis and the world health organization fracture risk assessment tool (FRAX): What they mean to the bone densiometrist and bone technologist. J Clin Densitom. 2008;11(4):473-477. doi: 10.1016/j.jocd.2008.04.003.
- MacLean C, Newberry S, Maglione M, et al. Systematic review: comparative effectiveness of treatments to prevent fractures in men and women with low bone density or osteoporosis. Ann Intern Med. 2007;148(3):197-213. doi: 10.7326/0003-4819-148-3-200802050-00198.
- Beaton DE, Vidmar M, Pitzul KB, et al. Addition of a fracture risk assessment to a coordinator’s role improved treatment rates within 6 months of screening in a fragility fracture screening program. J Am Geriatr Soc. 2017; 28(3):863-869. doi: 10.1007/s00198-016-3794-1.
- U.S. Preventative Services Task Force. Screening for osteoporosis. Ann Intern Med. 2011;154(5):356-364. doi: 10.7326/0003-4819-154-5-201103010-00307.
- Sunyecz JA. The use of calcium and vitamin D in the management of osteoporosis. Ther Clin Risk Manag. 2008;4(4):827-836.
- Eastell, R. (1998). Treatment of postmenopausal osteoporosis. N Engl J Med. 1998;338:736-746. doi: 10.1056/NEJM199803123381107.
- Cosman F, de Beur SJ, LeBoff MS, et al, National Osteoporosis Foundation. Clinician’s guide to prevention and treatment of osteoporosis. Osteoporos Int. 2014;25(10):2359-2381. doi: 10.1007/s00198-014-2794-2.
- Black DM, Schartz AV, Ensrud KE, et al, doi:10.1001/jama.296.24.2927.
- Schmidt GA, Horner KE, McDanel DL, Ross MB, Moores KG. Risks and benefits of long-term bisphosphonate therapy. Am J Health Syst Pharm. 2010;67(12):994-1001. doi: 10.2146/ajhp090506.
- Kraenzlin, ME, Meier C. Parathyroid hormone analogues in the treatment of osteoporosis. Nat Rev Endocrinol. 2011;7(11):647-656. doi: 10.1038/nrendo.2011.108.
- Miller P, Hattersley G, Riis B, et al. Effect of abaloparatide vs placebo on new vertebral fractures in postmenopausal women with osteoporosis. JAMA. 2016;316(7):722-733. doi: 10.1001/jama.2016.11136.
- TYMLOSTM [prescribing information]. Waltham, MA: Radius Health, Inc; 2017.
- Tetsunaga T, Tetsunaga T, Nishida K, et al. Denosumab and alendronate treatment in patients with back pain due to fresh osteoporotic vertebral fractures. J Orthop Sci. 2017;22(2):230-236. doi: 10.1016/j.jos.2016.11.017.
- Recker, RR, Mitlak BH, Ni X, Krege JH. Long-term raloxifene for postmenopausal osteoporosis. Curr Med Res Opin. 2011;27(9):1755-1761. doi: 10.1185/03007995.2011.606312.
- Yildirim K, Gureser G, Karatay S, et al. Comparison of the effects of alendronate, risedronate and calcitonin treatment in postmenopausal osteoporosis. J Back Musculoskelet Rehabil.2005;18(3/4):85-89. doi: 10.3233/BMR-2005-183-405.
- Christensen L, Iqbal S, Macarios D, Badamgarav E, Harley C. Cost of fractures commonly associated with osteoporosis in a managed-care population. J Med Econ. 2010;13(2):302-313. doi: 10.3111/13696998.2010.488969.
TAKE-HOME POINTS
- 3 million people sustain fragility fractures annually, and nearly 30% die within a year of the fracture.
- The incidence of fragility fractures increases in patients with comorbidities such as thyroid disease, diabetes, hypertension, and heart disease.
- The World Health Organization has developed a set of T-core criteria to diagnose osteoporosis in postmenopausal women: a score >–1 is normal; <–1 but >–2.5 signifies osteopenia; <–2.5 denotes osteoporosis; and <–2.5 with fragility fracture indicates severe osteoporosis.
- The Z score, not the T score, should be used to assess osteoporosis in premenopausal women, men <50 years, and children. The Z score is calculated by comparing the patient’s BMD with the mean BMD of their peers of a similar age, race, and gender. Z scores <–2.0 indicate low BMD for chronological age. A Z score > –2.0 is considered within the expected range for age.
- After an initial fragility fracture, the risk for additional ones increases significantly, making treatment of osteoporosis essential. The National Osteoporosis Foundation recommends treating osteoporosis with pharmacotherapy in patients with a high risk for fracture (T score <–2.5) or history of fragility fracture.26
The Characteristics of Surgeons Performing Total Shoulder Arthroplasty: Volume Consistency, Training, and Specialization
ABSTRACT
Total shoulder arthroplasty (TSA) has proved a cost-effective, reproducible procedure for multiple shoulder pathologies. As utilization of TSA continues to grow, it is important to investigate procedure diversity, training, and other characteristics of surgeons performing TSA. To identify surgeons performing TSA in the Medicare population, the Medicare Provider Utilization and Payment Databases from 2012 through 2014 were used. This dataset includes any provider who bills Medicare >10 times with a single billing code. A web-based search was performed for each physician performing >10 TSA in all years of the study to identify their surgical training characteristics. Between 2012 and 2014, 1374 surgeons (39 females [2.8%]) performed >10 TSA in Medicare patients in at least 1 year (71,973 TSA). Only 44.3% (609/1374) of surgeons met this threshold for all 3 years (55,538 TSA). Of these 609 surgeons, 191 (31.3%) were shoulder and elbow fellowship trained (21,444 TSA). Shoulder and elbow fellowship-trained surgeons were at earlier points in their careers and practiced in large referral-based centers with other surgeons performing TSA. In addition to TSA, surgeons performed other non-arthroplasty shoulder procedures (80.2% of surgeons), total knee arthroplasty (46.3%), repairs of traumatic injuries (29.8%), total hip arthroplasty (27.8%), non-arthroplasty knee surgeries (27.2%), elbow procedures (19.6%), and hand surgery (15.4%) during the study period. With less than one-third of TSA performed by shoulder and elbow fellowship-trained surgeons with consistent moderate-volume practices, the impact of consistent high-volume practices and targeted fellowship training on quality must be determined.
Continue to: With the adoption of reverse shoulder arthroplasty...
With the adoption of reverse shoulder arthroplasty, utilization of total shoulder arthroplasty (TSA) has increased substantially over the last decade.1–3 Such increases are likely secondary to an aging population, increased comfort with the procedure, and the adoption of broadened indications for reverse shoulder arthroplasty, especially in the setting of proximal humerus fractures in the elderly.4–7 Between 2012 and 2014 alone, the number of surgeons performing >10 TSA in Medicare patients annually increased by 28.6% (824 to 1060 surgeons) providing a 26.6% (20,824-26,365 procedures) increase in national volume in the Medicare population.2 With this boom in utilization, scrutiny of this now routine procedure and those performing it is necessary.
Prior reviews have demonstrated a strong link between surgeon and hospital TSA volume and outcomes of the procedure.8–10 Somerson and colleagues11 investigated fellowship training among surgeons performing TSA in 2012 and found that only 28% had completed a shoulder and elbow fellowship. In addition to prior analyses2, 12, Somerson and colleagues confirmed a persistent geographic variation in utilization of TSA.11 In conjunction with the evolution of shoulder arthroplasty, dedicated shoulder and elbow fellowship training has expanded. With a shift toward specialization in care, nearly 90% of orthopedic surgery residents plan to pursue shoulder and elbow fellowships, comprising 4.6% of (42/897) of available positions.13
What remains unknown is the specialization of surgeons performing TSA, the regularity of their arthroplasty volume, and trends in TSA specialization over time. Therefore, this study aims to (a) identify surgeons performing shoulder arthroplasty and cohort changes over time, (b) determine the case profile of surgeons consistently performing shoulder arthroplasty, and (c) establish the characteristics of shoulder arthroplasty surgeons with a specific focus on fellowship training.
METHODS
Prior to collecting surgeon-specific data, we identified surgeons performing TSA through the Centers for Medicare and Medicaid Services’ public release of “Medicare Provider Utilization and Payment Data: Physician and Other Supplier.”14 Datasets from 2012, 2013, and 2014 were used to identify all surgeons performing >10 TSAs (Current Procedural Terminology [CPT] Code 23472) during at least 1 of those years. This dataset provides the name, identification number, address, and all billing (by volume) for each unique CPT code submitted ≥10 times in a calendar year.
Once the cohort of surgeons had been generated, the number of surgeons consistently performing TSA year-over-year was determined. This allowed for an analysis of the consistency with which surgeons are performing moderate- to high-volume TSA. To form a case profile of surgeons performing TSA and observe how this shifted over time, a count and a description of each CPT code submitted by each surgeon was identified. To maintain patient privacy, only those claims made >10 times are reported for a provider (both physicians and physician-extenders are included in this dataset). First, all CPT codes were reviewed and tagged as surgical or non-surgical events. Then, every procedural CPT code identified was reviewed and categorized based upon anatomic location and procedure (eg, total knee arthroplasty [TKA]). It is important to note that all claims in this dataset are limited to those patients participating in Medicare’s fee-for-service program.
Specialization was defined as the number of categorized procedures as a percentage of all procedures performed on Medicare patients. The trends for national, regional, and individual specialization of TSA, arthroplasty (major joint), and shoulder procedures were determined.
Continue to: To investigate the characteristics of surgeons...
To investigate the characteristics of surgeons consistently performing TSA, all surgeons performing a minimum of 11 TSA in Medicare fee-for-service beneficiaries in all years between 2012 and 2014 were identified. Such surgeons were defined as consistent TSA surgeons. Investigation of this cohort included a web-based search of their self-reported post-graduate fellowship training and year of graduation from medical school. Using these data, the percentage of surgeons performing TSA who underwent formal shoulder and elbow training was determined. In addition, the impact of fellowship training on shoulder specialization and practice location was determined. Surgeons who had completed multiple fellowships were categorized under all of them. As such, there may be some duplication of surgeons in the comparisons. In addition, other potential characteristics of shoulder and elbow fellowship-trained surgeons were investigated: number of regional shoulder surgeons, urban area, total number of Medicare beneficiaries, average reimbursement for TSA, ethnicity of Medicare beneficiaries, and percentage of Medicare patients eligible for Medicaid. Geographic regions were defined by the Dartmouth Atlas and assigned by hospital referral region.15 These defined regions were used to assess the beneficiaries (number and characteristics) that individual surgeons were likely serving. The United States Census Bureau characterization of zip code-based regions as urban areas (population >50,000), urban clusters (2500 to 50,000), and rural region (<2500) was used to categorize practice location.16
Descriptive statistics were used initially to report these findings. To analyze predictors of utilization and specialization, comparative statistics were undertaken. For comparison of binomial variables between groups, a χ2analysis was utilized. For continuous variables, data normality was assessed. A skewness and kurtosis <2 and 12, respectively, was considered to represent parametric data. For parametric data, the mean was reported; conversely, the median is reported for non-parametric data. To assess continuous variables between groups, a t test or a Wilcoxon rank-sum test was used for parametric and non-parametric distributions, respectively.
RESULTS
Between 2012 and 2014, 1374 surgeons (39 females [2.8%]) performed >10 TSA in Medicare patients in at least 1 year, for a combined total of 71,973 TSAs (Table 1). In 2012, only 834 surgeons (13 females [1.6%]) performed a minimum of 10 TSA in Medicare patients (21,137 arthroplasties; 25.3 per surgeon). This increased to 1078 surgeons (33 females [3.1%]; P = .04) performing 26,865 TSA (24.92 per surgeon) in 2014. Utilization of non-physician assistants in TSA also increased significantly over this period, with 307 assisting in 6885 TSAs (22.4 per provider) in 2012 and 465 assisting 10,433 TSA (22.4 per provider) in 2014. When all procedures were considered, including those performed at outpatient visits, 1319 physicians (95.9% of cohort) were active in 2012—providing either surgical procedures or outpatient consults to the Medicare population. Yet, only 63.2% performed >10 TSA in Medicare patients. The number of active surgeons performing TSA increased to 79.6% (1078/1353) in 2014 (P < .001).
Table 1. Trends in the Number of Providers Performing TSA between 2012 and 2014*
2012 | 2013 | 2014 | Total | |
Providers (no.) | 1141 | 1373 | 1543 | 1994 |
Physicians | 834 | 984 | 1,078 | 1,374 |
Non-physicians | 307 | 389 | 465 | 620 |
TSA (no.) | 28,022 | 32,641 | 37,298 | 97,961 |
Physicians | 21,137 | 23,971 | 26,865 | 71,973 |
Non-physicians | 6,885 | 8,670 | 10,433 | 25,988 |
TSA per provider | 24.5 | 23.8 | 24.2 | 49.2 |
Physicians | 25.3 | 24.3 | 24.9 | 52.4 |
Non-physicians | 22.4 | 22.3 | 22.4 | 41.2 |
Procedures (no.) | 210,845 | 224,123 | 227,305 | 662,273 |
Physicians | 152,862 | 160,114 | 160,851 | 473,827 |
Non-physicians | 57,983 | 64,009 | 66,454 | 188,446 |
Procedure per provider | 114.4 | 116.8 | 116.5 | 332.13 |
Physicians | 115.9 | 118.9 | 118.9 | 344.9 |
Non-physicians | 110.7 | 111.9 | 111.1 | 303.9 |
Active providers (no.) | 1843 | 1919 | 1951 | 1994 |
Physicians | 1319 | 1347 | 1353 | 1374 |
Non-physicians | 524 | 572 | 598 | 620 |
* Included are the number of arthroplasties and total procedures over time among this cohort. The number of active providers, determined by billing Medicare for office or surgical procedures within that year, is reported.
Abbreviation: TSA, total shoulder arthroplasty.
In addition to TSA, this cohort of surgeons submitted 240 unique CPT codes with case volumes >10 annually over the 3-year study period. Of these, 80.2% (1102/1374) of surgeons performed non-arthroplasty shoulder procedures on Medicare patients, for a combined total of 202,335 procedures over the 3-year study period (Table 2). A significant proportion of these procedures were arthroscopic debridement (60,014 procedures performed by 908 surgeons) and arthroscopic rotator cuff repair (47,089 procedures performed by 809 surgeons). Just under half (49.1%; 674/1374) of surgeons performing TSA also performed TKA during this period (77,873 arthroplasties). Fewer surgeons (27.8%; 382/1374) performed total hip arthroplasty during this period (27,322 arthroplasties). Other procedure types that this group of surgeons routinely performed on Medicare patients were repairs of traumatic injuries (29.8%), non-arthroplasty knee surgeries (27.2%), elbow procedures (19.6%), and hand surgery (15.4%). By case load, non-arthroplasty shoulder procedures consisted of 43% of Medicare volume over the study period (Figure 1). Between 2012 and 2014, the average proportion of Medicare cases that were shoulder arthroplasties increased from 13.8% (21,137/152,862) to 16.7% (26,865/160,851; P = .001). Shoulder arthroplasty constituted 100% of the Medicare surgical case volume for 67 (4.9%; 67/1374) of the surgeons.
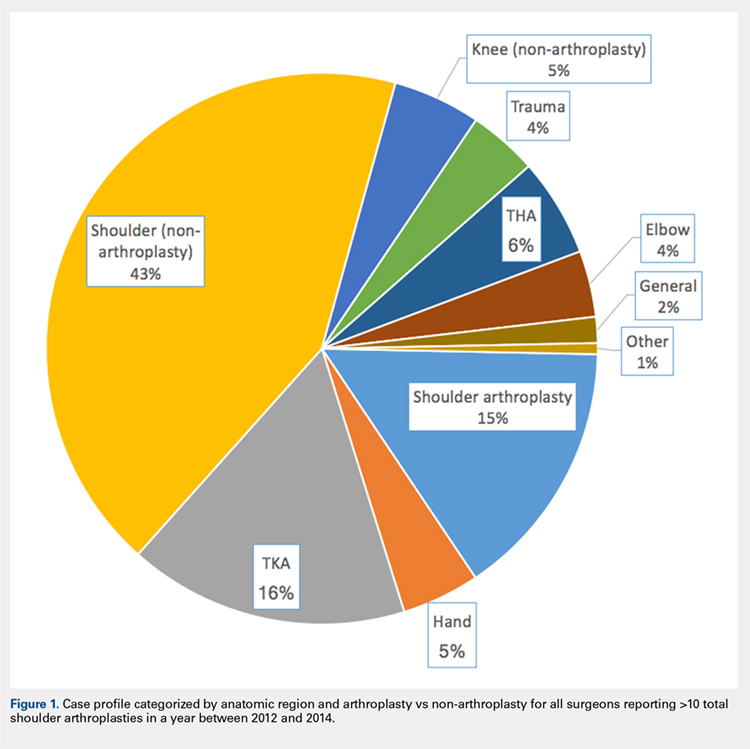
Table 2. Case Volumes over Time with All Procedures Categorized by Anatomic Region and Arthroplasty vs Non-arthroplasty*
2012 | 2013 | 2014 | Total | |
Shoulder arthroplasty | 21,351 (n=837) | 24,128 (n=984) | 26,902 (n=1,078) | 72,381 (n=1,374) |
23472: total shoulder arthroplasty | 21,137 (n=834) | 23,971 (n=984) | 26,865 (n=1,078) | 71,973 (n=1,374) |
23470: Hemiarthroplasty | 214 (n=14) | 84 (n=6) | 37 (n=2) | 335 (n=15) |
Shoulder (non-arthroplasty) | 65,947 (n=887) | 68,746 (n=942) | 67,642 (n=932) | 202,335 (n=1,102) |
29826: arthroscopic acromioplasty | 19,152 (n=724) | 20,367 (n=760) | 20,495 (n=754) | 60,014 (n=908) |
29827: arthroscopic rotator cuff repair | 14,700 (n=613) | 15,963 (n=664) | 16,426 (n=658) | 47,089 (n=809) |
23412: open rotator cuff repair | 1957 (n=88) | 2046 (n=90) | 2112 (n=2,112) | 6115 (n=143) |
23430: Open biceps tenodesis | 4063 (n=178) | 3998 (n=167) | 4601 (n=185) | 12,662 (n=288) |
29823: arthroscopic major debridement | 7428 (n=301) | 7745 (n=309) | 5202 (n=210) | 20,375 (n=417) |
Total knee arthroplasty | 25,640 (n=565) | 26,558 (n=587) | 25,675 (n=580) | 77,873 (n=637) |
Total hip arthroplasty | 8729 (n=316) | 9226 (n=318) | 9367 (n=330) | 27,322 (n=382) |
Trauma | 6454 (n=260) | 6396 (n=254) | 6364 (n=261) | 19,214 (n=410) |
27245: surgical treatment of broken thigh bone (intertrochanteric) | 2602 (n=162) | 2654 (n=164) | 2537 (n=160) | 7793 (n=274) |
27236: surgical treatment of broken thigh bone (hemiarthroplasty) | 1961 (n=123) | 1703 (n=111) | 1702 (n=112) | 5366 (n=205) |
Hand | 6343 (n=139) | 7321 (n=154) | 8006 (n=172) | 21,670 (n=211) |
Elbow | 6113 (n=198) | 6139 (n=204) | 6131 (n=198) | 18,383 (n=270) |
Knee (non-arthroplasty) | 8514 (n=275) | 8140 (n=275) | 7689 (n=230) | 24,343 (n=374) |
Outpatient visits | 879,740 (n=1,282) | 907,124 (n=1,320) | 921,291 (n=1,327) | 2,708,155 (n=1,342) |
New patient | 195,898 (n=1,276) | 192,937 (n=1,305) | 191,427 (n=1,315) | 571,203 (n=1,332) |
Existing patient | 740,307 (n=1,279) | 714,187 (n=1,316) | 729,864 (n=1,324) | 2,29,976 (n=1,338) |
* Procedures of interest with high case volumes are reported individually.
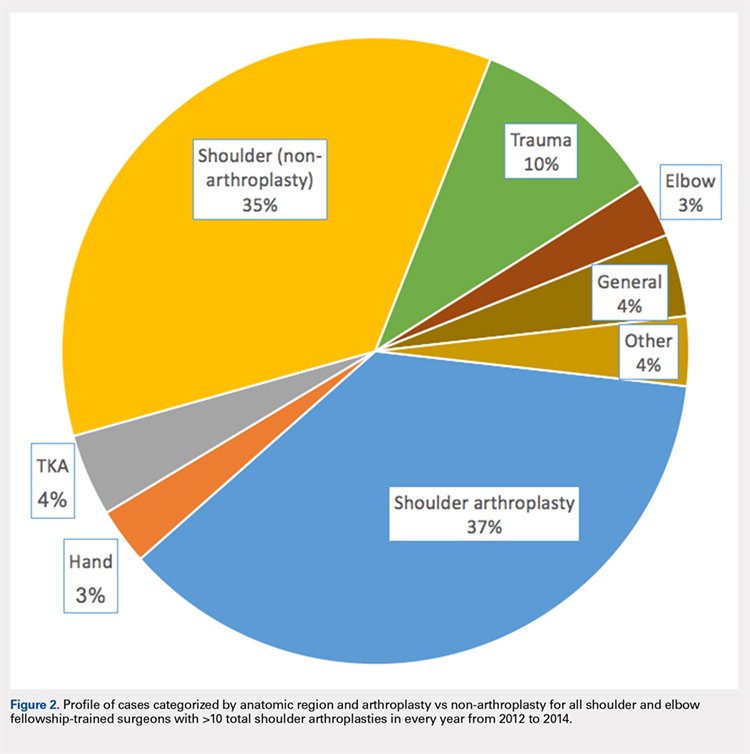
Only 44.3% (609/1374) of surgeons performed TSA in a minimum of 11 Medicare patients in all 3 years of the study period (consistent providers of TSA), providing a total of 55,538 TSA (77.2%; 55,538/71,973). When fellowship training was evaluated, 191 (31.4%; 191/609) of these surgeons were shoulder and elbow fellowship trained (21,444 TSA; 38.6%; Table 3). More than one-third (36.6%; 223/609) had completed a sports surgery fellowship (18,899 TSA; 34.0%). Surgeons trained in hand surgery (12.5%; 76/609) and adult reconstruction (5.3%; 32/610) also made contributions to meeting the TSA demand with 6971 (12.6%) and 2485 (4.5%) TSA, respectively. One-fifth of this cohort (18.1%; 110/609) had unknown fellowship training: they either reported no fellowship (13.6%; 83/609) or did not specify the type of training (4.4%; 27/609). Shoulder and elbow fellowship-trained surgeons performed more TSA (median: 89.0 TSA per surgeon between 2012 and 2014) than surgeons without shoulder and elbow fellowship training (median: 67.0 TSA per surgeon; P < 0.001). More than one-third (37%) of shoulder and elbow fellowship-trained surgeons’ surgical case volume was comprised of TSA, with an additional 35% from non-arthroplasty shoulder procedures (Figure 2). In order for the current supply of shoulder and elbow fellowship-trained surgeons to meet the Medicare TSA demand, each fellowship graduate would have to perform 140.6 TSA in Medicare patients annually. Shoulder and elbow fellowship-trained surgeons were more likely to practice in referral regions with an increased Medicare population (P < .001), an increased number of surgeons performing TSA (P < .001), and a higher proportion of Medicaid-eligible patients (P = .01; Table 4). Shoulder and elbow fellowship-trained surgeons (18.7 years post-medical school graduation) were also earlier in their careers than other consistent TSA surgeons (23.1 years post-graduation; P < .001).
Table 3. A Representation of Fellowships Among TSA Surgeons and Their Shoulder Arthroplasty Case Load*
Fellowship | Surgeons (%) | 2012-2014 (no, %) | SA Medicare Cases (%) | Average Surgeon Annual SA Volume |
Shoulder and elbow | 191 (31.4%) | 21,444 (38.6%) | 29.3% | 37.4 |
Hand surgery | 76 (12.5%) | 6971 (12.6%) | 17.1% | 30.6 |
Sports | 223 (36.6%) | 18,899 (34.0%) | 19.4% | 28.3 |
Trauma | 14 (2.3%) | 1270 (2.3%) | 10.9% | 30.2 |
Adult reconstruction | 32 (5.3%) | 2485 (4.5%) | 10.2% | 25.9 |
Unknown/none | 110 (18.1%) | 8489 (15.3%) | 16.3% | 25.7 |
|
|
|
| |
1 Fellowship | 459 (75.3%) | 42,065 (75.7%) | 20.7% | 30.5 |
≥2 Fellowships | 67 (11.0%) | 7122 (12.8%) | 22.5% | 35.4 |
* Not all fellowships (eg, oncology) included due to small numbers. Also, many surgeons performed multiple fellowships.
Abbreviations: SA, shoulder arthroplasty; TSA, total shoulder arthroplasty.
Table 4. Breakdown of Geographic Characteristics of Orthopedic Surgeons Consistently
Performing TSA between 2012 and 2014 Stratified by Fellowship Training
Abbreviations: HRR, hospital referral region; TSA, total shoulder arthroplasty.
Fellowship | Percentage in Non-Urban Area | Average No. of Other TSA Surgeons within HRR | Median Proportion of Patients Eligible for Medicaid within HRR | Average Proportion of Caucasian Patients within HRR | Average Population in Practicing Zip Code | Average No. of Medicare Beneficiaries in HRR | Average No. Years from Medical School Graduation |
Shoulder and elbow | 7.3% | 10.5 | 12.6 | 84.7 | 26,620.1 | 224,868.3 | 18.7 |
Other fellowships | 10.3% | 8.6 | 11.1 | 85.6 | 27,619.7 | 177,939.7 | 23.1 |
P value | 0.29 | <0.001 | 0.01 | 0.30 | 0.41 | <0.001 | <0.001 |
Hand surgery | 7.9% | 8.1 | 12.8 | 83.7 | 24,022.8 | 179,370.8 | 23.9 |
Sports | 11.2% | 8.9 | 11.9 | 85.6 | 28,588.9 | 185,902.4 | 21.2 |
Trauma | 21.4% | 7.7 | 13.8 | 85.5 | 20,065.9 | 170,807.6 | 25.6 |
Adult reconstruction | 6.3% | 8.7 | 12.8 | 86.9 | 26,601.5 | 173,280.1 | 22.4 |
None/unknown | 10.9% | 8.5 | 12.0 | 86.4 | 28,173.6 | 166,522.5 | 27.0 |
Continue to: DISCUSSION...
DISCUSSION
Utilization of TSA has continued to rise; however, access to this cost-effective procedure was recently demonstrated to be limited.11 In a separate analysis, we established the continued rise in use of TSA in the Medicare population, coupled with an increase in the number of surgeons routinely performing TSA.2 Multiple analyses have demonstrated the importance of high-volume surgeons and hospitals familiar with the intricacies of shoulder arthroplasty concepts in minimizing complications, improving the quality and decreasing the cost of TSA.6,10,17 Specifically, Singh and colleagues18 demonstrated from a multi-center registry that surgeons and hospitals with greater shoulder arthroplasty volumes had decreased intra-operative blood loss, operative time, and hospital length of stay. As the demand for TSA, both anatomic and reverse, continues to rise, it is imperative that the healthcare delivery system is optimized to provide the best possible care. Before we can determine whether specialized training in shoulder arthroplasty influences surgical outcomes, characteristics and training of surgeons performing TSA should be described.
The number of surgeons performing >10 TSA in the Medicare population rose significantly between 2012 and 2014 (29.3%). However, the number of TSAs per surgeon over this time period remained consistent (approximately 25 per surgeon). Furthermore, the increase in the number of surgeons performing a reportable volume of TSA by 2014 was from the addition of already active surgeons (ie, the growth in TSA was not from the addition of newly trained arthroplasty surgeons but originated from the existing orthopedic surgeon workforce). In a recently published analysis, Somerson and colleagues, 11 using this same dataset, demonstrated persistent limitations in access to high-volume TSA surgeons. In a more recent analysis, we showed that while still lacking for some patients, access to a high-volume TSA surgeon has improved significantly over the past 3 years, with 96.9% of the United States population residing within 200 kilometers of a high-volume TSA surgeon (>20 Medicare cases).2 This analysis validates those findings, with the caveat that the average annual volume per surgeon is not increasing. What remains unknown, due to limitations of this dataset, is how many surgeons are not identified because they are performing ≤10 TSA each year or are performing TSA in non-Medicare patients.
With the specialization of healthcare delivery, specifically in orthopedics, it is imperative that mechanisms for providing specialty-focused care be established. However, the proportion of their practice that surgeons dedicate to TSA was unknown. This study demonstrates that this proportion is increasing. Including non-arthroplasty procedures, more than half (58%) of the procedures performed by this surgeon cohort were shoulder-specific. Furthermore, this analysis demonstrates that surgeons performing TSA have significant case diversity, including nearly half of the cohort performing TKA. Repeated evidence has demonstrated the effect of case volume on improved outcomes following orthopedic procedures.8,19–21 The pre-existing location-based model for delivering orthopedic care supports case diversity; however, this model continues to be challenged with high-volume centers of excellence and patient travel.22–24 Hip and knee arthroplasty experienced a similar surge in demand, with a subsequent shift in care to high-volume surgeons and centers.25 Shoulder and elbow fellowship-trained surgeons would need to nearly quadruple their current Medicare TSA volume to meet the entire current demand for TSA in the Medicare population (and this does not account for TSA performed by very low-volume surgeons not included in this cohort). With increased utilization of TSA, policymakers and the orthopedic community must determine the structure of delivery (centers of excellence or medium-volume disseminated throughout the country) that is optimal.
For those surgeons consistently performing TSA over the study period, fellowship training was diverse. While the current focus in orthopedics is on case volume, research in other specialties, namely general surgery, has provided repeated evidence that surgical specialization (more so than high case volume) provides improved outcomes.26–29Furthermore, Leopold and colleagues30 demonstrated an inverse relationship between competency in performing a procedure and confidence in one’s ability to do so. In their study, educational intervention provided improved competency in the procedure. Less than one-third (29.8%) of TSA in this cohort were performed by a shoulder and elbow fellowship-trained surgeon consistently performing this procedure. Approximately another quarter (26.2%) were performed by consistent TSA surgeons trained in sports surgery. Meanwhile, 34.6% of TSA in this study cohort were performed by a surgeon who did not consistently meet the minimum threshold in all study years (16,435 TSA; 22.8%) or by a surgeon performing TSA without fellowship training (8,489 TSA; 11.8%). There has been a trend toward orthopedic subspecialty training with an increased demand for fellowship-trained surgeons.31 Despite this and the complexities of TSA, many continue to be performed by surgeons with an inconsistent volume and those without arthroplasty-specific fellowship training. The available evidence supports a push toward the fellowship-trained, high-volume TSA surgeon in providing reproducible high-quality shoulder arthroplasty care. For now, that surgeon is more likely to be earlier in his/her career and reside in large, referral-based centers surrounded by other surgeons performing TSA.
These findings must be considered in the light of the study limitations. First, this is a large publicly available database. While this type of database provides a unique opportunity to assess the geographic distributions and characteristics of orthopedic surgeons, specifically those performing TSA, it completely prevents any assessment of the relationship between these findings and quality. As such, while the reader may generate hypotheses regarding the implications of our findings on the quality of TSA delivery, the true effects cannot be determined. In the same vein, for the purpose of privacy, surgeons performing ≤10 TSA were not included in this dataset. This limitation prevents the identification of low-volume TSA surgeons. Also, it is likely that the observed increase in surgeons over time is likely a reflection of small increases in volume for surgeons already performing TSA. Lastly, a web-based search was undertaken to identify surgeons’ self-reported fellowship training. The results of this web-based search could not be validated, and it is possible that fellowship training, or the lack thereof, was mischaracterized and simply not obtainable through a web-based search. Furthermore, it is not possible to fully assess the extent of high-quality TSA training in these various fellowships.
CONCLUSION
In just the past decade, the utilization of TSA in the Medicare population has increased significantly. However, this increase was not achieved by the addition of highly specialized, high-volume surgeons but by the addition of many surgeons performing lower numbers of TSA surgeries. Furthermore, for those performing this cost-effective procedure, TSA constitutes a relatively small proportion of the surgeries they perform. Shoulder and elbow fellowship-trained surgeons currently account for a low percentage of the overall number of surgeons performing TSA. The implications of these findings must be considered and investigated.
1. Kim SH, Wise BL, Zhang Y, Szabo RM. Increasing incidence of shoulder arthroplasty in the United States. J Bone Joint Surg Am. 2011;93(24):2249-2254. doi:10.2106/JBJS.J.01994.
2. Zmistowski B, Padegimas EM, Howley M, Abboud J, Williams G, Namdari S. Trends and Variability in the Use of Total Shoulder Arthroplasty for Medicare Patients. J Am Acad Orthop Surg. 2018;26(4):133-141. doi:10.5435/JAAOS-D-16-00720
3. Day JS, Lau E, Ong KL, Williams GR, Ramsey ML, Kurtz SM. Prevalence and projections of total shoulder and elbow arthroplasty in the United States to 2015. J Shoulder Elbow Surg. 2010;19(8):1115-1120. doi: http://www.jshoulderelbow.org/article/S1058-2746(10)00110-2/abstract.
4. Day JS, Paxton ES, Lau E, Gordon VA, Abboud JA, Williams GR. Use of reverse total shoulder arthroplasty in the Medicare population. J Shoulder Elbow Surg. 2015;24(5):766-772. doi:10.1016/j.jse.2014.12.023.
5. Schairer WW, Nwachukwu BU, Lyman S, Craig EV, Gulotta LV. National utilization of reverse total shoulder arthroplasty in the United States. J Shoulder Elbow Surg. 2015;24(1):91-97. doi:10.1016/j.jse.2014.08.026.
6. Westermann RW, Pugely AJ, Martin CT, Gao Y, Wolf BR, Hettrich CM. Reverse shoulder arthroplasty in the United States: A comparison of national volume, patient demographics, complications, and surgical indications. Iowa Orthop J. 2015;35:1-7.
7. Acevedo DC, Mann T, Abboud JA, Getz C, Baumhauer JF, Voloshin I. Reverse total shoulder arthroplasty for the treatment of proximal humeral fractures: patterns of use among newly trained orthopedic surgeons. J Shoulder Elbow Surg. 2014;23(9):1363-1367. doi: http://www.jshoulderelbow.org/article/S1058-2746(14)00036-6/abstract.
8. Hammond JW, Queale WS, Kim TK, McFarland EG. Surgeon experience and clinical and economic outcomes for shoulder arthroplasty. J Bone Joint Surg Am. 2003;85-A(12):2318-2324.
9. Jain NB, Kuye I, Higgins LD, Warner JJP. Surgeon volume is associated with cost and variation in surgical treatment of proximal humeral fractures. Clin Orthop Relat Res. 2012;471(2):655-664. doi:10.1007/s11999-012-2481-6.
10. Lyman S, Jones EC, Bach PB, Peterson MGE, Marx RG. The association between hospital volume and total shoulder arthroplasty outcomes. Clin Orthop Relat Res. 2005;(432):132-137. doi:10.1097/01.blo.0000150571.51381.9a.
11. Somerson JS, Stein BA, Wirth MA. Distribution of high-volume shoulder arthroplasty surgeons in the United States: Data from the 2014 Medicare provider utilization and payment data release. J Bone Joint Surg Am. 2016;98(18):e77. doi:10.2106/JBJS.15.00776.
12. Fisher ES, Bell J-E, Tomek IM, Esty AR, Goodman DC. Trends and regional variation in hip, knee, and shoulder Replacement. Atlases and Reports. Dartmouth Atlas of Health Care. https://www.dartmouthatlas.org/atlases-and-reports/. Accessed December 14, 2018.
13. Daniels AH, DiGiovanni CW. Is subspecialty fellowship training emerging as a necessary component of contemporary orthopaedic surgery education? J Grad Med Educ. 2014;6(2):218-221. doi:10.4300/JGME-D-14-00120.1.
14. Department of Health and Human Services, Centers for Medicare and Medicaid Services. Physician and other supplier Data 2012 CY 2012. https://www.cms.gov/Research-Statistics-Data-and-Systems/Statistics-Tren.... Published October 5, 2015. Accessed July 25, 2016.
15. The Dartmouth Institute for Health Policy and Clinical Practice. Dartmouth Atlas of Health Care. Understanding the Efficiency and Effectiveness of the Health Care System. http://www.dartmouthatlas.org/. Accessed January 31, 2014.
16. United States Department of Commerce. United States Census Bureau. 2010 Census Urban and Rural Classification and Urban Area Criteria. https://www.census.gov/geo/reference/ua/urban-rural-2010.html. Accessed September 30, 2016.
17. Kempton LB, Ankerson E, Wiater JM. A complication-based learning curve from 200 reverse shoulder arthroplasties. Clin Orthop Relat Res. 2011;469(9):2496-2504. doi:10.1007/s11999-011-1811-4.
18. Singh A, Yian EH, Dillon MT, Takayanagi M, Burke MF, Navarro RA. The effect of surgeon and hospital volume on shoulder arthroplasty perioperative quality metrics. J Bone Joint Surg Am. 2014;23(8):1187-1194. doi:10.1016/j.jse.2013.11.017.
19. Jain N, Pietrobon R, Hocker S, Guller U, Shankar A, Higgins LD. The relationship between surgeon and hospital volume and outcomes for shoulder arthroplasty. J Bone Joint Surg Am. 2004;86(3):496-505.
20. Taylor HD, Dennis DA, Crane HS. Relationship between mortality rates and hospital patient volume for Medicare patients undergoing major orthopaedic surgery of the hip, knee, spine, and femur. J Arthroplasty. 1997;12(3):235-242. doi:10.1016/S0883-5403(97)90018-8.
21. Walch G, Bacle G, Lädermann A, Nové-Josserand L, Smithers CJ. Do the indications, results, and complications of reverse shoulder arthroplasty change with surgeon’s experience? J Bone Joint Surg Am. 2012;21(11):1470-1477. doi:10.1016/j.jse.2011.11.010.
22. FitzGerald JD, Soohoo NF, Losina E, Katz JN. Potential impact on patient residence to hospital travel distance and access to care under a policy of preferential referral to high-volume knee replacement hospitals. Arthritis Care Res. 2012;64(6):890-897. doi:10.1002/acr.21611.
23. Maradit Kremers H, Salduz A, Schleck CD, Larson DR, Berry DJ, Lewallen DG. Referral bias in primary total knee arthroplasty: retrospective analysis of 22,614 surgeries in a tertiary referral center. J Arthroplasty. doi:10.1016/j.arth.2016.08.014.
24. Robinson JC, MacPherson K. Payers test reference pricing and centers of excellence to steer patients to low-price and high-quality providers. Health Affairs. 2012;31(9):2028-2036. doi: 10.1377/hlthaff.2011.1313
25. Laucis NC, Chowdhury M, Dasgupta A, Bhattacharyya T. Trend toward high-volume hospitals and the influence on complications in knee and hip arthroplasty. J Bone Joint Surg Am. 2016;98(9):707-712. doi:10.2106/JBJS.15.00399.
26. Anwar S, Fraser S, Hill J. Surgical specialization and training–its relation to clinical outcome for colorectal cancer surgery. J Eval Clin Pract. 2012;18(1):5-11. doi:10.1111/j.1365-2753.2010.01525.x.
27. Snow BW, Catwright PC, Young MD. Does surgical subspecialization in pediatrics provide high-quality, cost-effective patient care? Pediatrics. 1996;97(1):14-17.
28. Smith J a. E, King PM, Lane RHS, Thompson MR. Evidence of the effect of ‘specialization’ on the management, surgical outcome and survival from colorectal cancer in Wessex. Br J Surg. 2003;90(5):583-592. doi:10.1002/bjs.4085.
29. Hall BL, Hsaio EY, Majercik S, Hirbe M, Hamilton BH. The impact of surgeon specialization on patient mortality: Examination of a continuous Herfindahl-Hirschman Index. Ann Surg. 2009;249(5):708-716. doi: 10.1097/SLA.0b013e3181a335f8.
30. Leopold SS, Morgan HD, Kadel NJ, Gardner GC, Schaad DC, Wolf FM. Impact of educational intervention on confidence and competence in the performance of a simple surgical task. J Bone Joint Surg Am. 2005;87(5):1031-1037. doi:10.2106/JBJS.D.02434.
31. Morrell NT, Mercer DM, Moneim MS. Trends in the orthopedic job market and the importance of fellowship subspecialty training. Orthopedics. 2012;35(4):e555-e560. doi:10.3928/01477447-20120327-13.
ABSTRACT
Total shoulder arthroplasty (TSA) has proved a cost-effective, reproducible procedure for multiple shoulder pathologies. As utilization of TSA continues to grow, it is important to investigate procedure diversity, training, and other characteristics of surgeons performing TSA. To identify surgeons performing TSA in the Medicare population, the Medicare Provider Utilization and Payment Databases from 2012 through 2014 were used. This dataset includes any provider who bills Medicare >10 times with a single billing code. A web-based search was performed for each physician performing >10 TSA in all years of the study to identify their surgical training characteristics. Between 2012 and 2014, 1374 surgeons (39 females [2.8%]) performed >10 TSA in Medicare patients in at least 1 year (71,973 TSA). Only 44.3% (609/1374) of surgeons met this threshold for all 3 years (55,538 TSA). Of these 609 surgeons, 191 (31.3%) were shoulder and elbow fellowship trained (21,444 TSA). Shoulder and elbow fellowship-trained surgeons were at earlier points in their careers and practiced in large referral-based centers with other surgeons performing TSA. In addition to TSA, surgeons performed other non-arthroplasty shoulder procedures (80.2% of surgeons), total knee arthroplasty (46.3%), repairs of traumatic injuries (29.8%), total hip arthroplasty (27.8%), non-arthroplasty knee surgeries (27.2%), elbow procedures (19.6%), and hand surgery (15.4%) during the study period. With less than one-third of TSA performed by shoulder and elbow fellowship-trained surgeons with consistent moderate-volume practices, the impact of consistent high-volume practices and targeted fellowship training on quality must be determined.
Continue to: With the adoption of reverse shoulder arthroplasty...
With the adoption of reverse shoulder arthroplasty, utilization of total shoulder arthroplasty (TSA) has increased substantially over the last decade.1–3 Such increases are likely secondary to an aging population, increased comfort with the procedure, and the adoption of broadened indications for reverse shoulder arthroplasty, especially in the setting of proximal humerus fractures in the elderly.4–7 Between 2012 and 2014 alone, the number of surgeons performing >10 TSA in Medicare patients annually increased by 28.6% (824 to 1060 surgeons) providing a 26.6% (20,824-26,365 procedures) increase in national volume in the Medicare population.2 With this boom in utilization, scrutiny of this now routine procedure and those performing it is necessary.
Prior reviews have demonstrated a strong link between surgeon and hospital TSA volume and outcomes of the procedure.8–10 Somerson and colleagues11 investigated fellowship training among surgeons performing TSA in 2012 and found that only 28% had completed a shoulder and elbow fellowship. In addition to prior analyses2, 12, Somerson and colleagues confirmed a persistent geographic variation in utilization of TSA.11 In conjunction with the evolution of shoulder arthroplasty, dedicated shoulder and elbow fellowship training has expanded. With a shift toward specialization in care, nearly 90% of orthopedic surgery residents plan to pursue shoulder and elbow fellowships, comprising 4.6% of (42/897) of available positions.13
What remains unknown is the specialization of surgeons performing TSA, the regularity of their arthroplasty volume, and trends in TSA specialization over time. Therefore, this study aims to (a) identify surgeons performing shoulder arthroplasty and cohort changes over time, (b) determine the case profile of surgeons consistently performing shoulder arthroplasty, and (c) establish the characteristics of shoulder arthroplasty surgeons with a specific focus on fellowship training.
METHODS
Prior to collecting surgeon-specific data, we identified surgeons performing TSA through the Centers for Medicare and Medicaid Services’ public release of “Medicare Provider Utilization and Payment Data: Physician and Other Supplier.”14 Datasets from 2012, 2013, and 2014 were used to identify all surgeons performing >10 TSAs (Current Procedural Terminology [CPT] Code 23472) during at least 1 of those years. This dataset provides the name, identification number, address, and all billing (by volume) for each unique CPT code submitted ≥10 times in a calendar year.
Once the cohort of surgeons had been generated, the number of surgeons consistently performing TSA year-over-year was determined. This allowed for an analysis of the consistency with which surgeons are performing moderate- to high-volume TSA. To form a case profile of surgeons performing TSA and observe how this shifted over time, a count and a description of each CPT code submitted by each surgeon was identified. To maintain patient privacy, only those claims made >10 times are reported for a provider (both physicians and physician-extenders are included in this dataset). First, all CPT codes were reviewed and tagged as surgical or non-surgical events. Then, every procedural CPT code identified was reviewed and categorized based upon anatomic location and procedure (eg, total knee arthroplasty [TKA]). It is important to note that all claims in this dataset are limited to those patients participating in Medicare’s fee-for-service program.
Specialization was defined as the number of categorized procedures as a percentage of all procedures performed on Medicare patients. The trends for national, regional, and individual specialization of TSA, arthroplasty (major joint), and shoulder procedures were determined.
Continue to: To investigate the characteristics of surgeons...
To investigate the characteristics of surgeons consistently performing TSA, all surgeons performing a minimum of 11 TSA in Medicare fee-for-service beneficiaries in all years between 2012 and 2014 were identified. Such surgeons were defined as consistent TSA surgeons. Investigation of this cohort included a web-based search of their self-reported post-graduate fellowship training and year of graduation from medical school. Using these data, the percentage of surgeons performing TSA who underwent formal shoulder and elbow training was determined. In addition, the impact of fellowship training on shoulder specialization and practice location was determined. Surgeons who had completed multiple fellowships were categorized under all of them. As such, there may be some duplication of surgeons in the comparisons. In addition, other potential characteristics of shoulder and elbow fellowship-trained surgeons were investigated: number of regional shoulder surgeons, urban area, total number of Medicare beneficiaries, average reimbursement for TSA, ethnicity of Medicare beneficiaries, and percentage of Medicare patients eligible for Medicaid. Geographic regions were defined by the Dartmouth Atlas and assigned by hospital referral region.15 These defined regions were used to assess the beneficiaries (number and characteristics) that individual surgeons were likely serving. The United States Census Bureau characterization of zip code-based regions as urban areas (population >50,000), urban clusters (2500 to 50,000), and rural region (<2500) was used to categorize practice location.16
Descriptive statistics were used initially to report these findings. To analyze predictors of utilization and specialization, comparative statistics were undertaken. For comparison of binomial variables between groups, a χ2analysis was utilized. For continuous variables, data normality was assessed. A skewness and kurtosis <2 and 12, respectively, was considered to represent parametric data. For parametric data, the mean was reported; conversely, the median is reported for non-parametric data. To assess continuous variables between groups, a t test or a Wilcoxon rank-sum test was used for parametric and non-parametric distributions, respectively.
RESULTS
Between 2012 and 2014, 1374 surgeons (39 females [2.8%]) performed >10 TSA in Medicare patients in at least 1 year, for a combined total of 71,973 TSAs (Table 1). In 2012, only 834 surgeons (13 females [1.6%]) performed a minimum of 10 TSA in Medicare patients (21,137 arthroplasties; 25.3 per surgeon). This increased to 1078 surgeons (33 females [3.1%]; P = .04) performing 26,865 TSA (24.92 per surgeon) in 2014. Utilization of non-physician assistants in TSA also increased significantly over this period, with 307 assisting in 6885 TSAs (22.4 per provider) in 2012 and 465 assisting 10,433 TSA (22.4 per provider) in 2014. When all procedures were considered, including those performed at outpatient visits, 1319 physicians (95.9% of cohort) were active in 2012—providing either surgical procedures or outpatient consults to the Medicare population. Yet, only 63.2% performed >10 TSA in Medicare patients. The number of active surgeons performing TSA increased to 79.6% (1078/1353) in 2014 (P < .001).
Table 1. Trends in the Number of Providers Performing TSA between 2012 and 2014*
2012 | 2013 | 2014 | Total | |
Providers (no.) | 1141 | 1373 | 1543 | 1994 |
Physicians | 834 | 984 | 1,078 | 1,374 |
Non-physicians | 307 | 389 | 465 | 620 |
TSA (no.) | 28,022 | 32,641 | 37,298 | 97,961 |
Physicians | 21,137 | 23,971 | 26,865 | 71,973 |
Non-physicians | 6,885 | 8,670 | 10,433 | 25,988 |
TSA per provider | 24.5 | 23.8 | 24.2 | 49.2 |
Physicians | 25.3 | 24.3 | 24.9 | 52.4 |
Non-physicians | 22.4 | 22.3 | 22.4 | 41.2 |
Procedures (no.) | 210,845 | 224,123 | 227,305 | 662,273 |
Physicians | 152,862 | 160,114 | 160,851 | 473,827 |
Non-physicians | 57,983 | 64,009 | 66,454 | 188,446 |
Procedure per provider | 114.4 | 116.8 | 116.5 | 332.13 |
Physicians | 115.9 | 118.9 | 118.9 | 344.9 |
Non-physicians | 110.7 | 111.9 | 111.1 | 303.9 |
Active providers (no.) | 1843 | 1919 | 1951 | 1994 |
Physicians | 1319 | 1347 | 1353 | 1374 |
Non-physicians | 524 | 572 | 598 | 620 |
* Included are the number of arthroplasties and total procedures over time among this cohort. The number of active providers, determined by billing Medicare for office or surgical procedures within that year, is reported.
Abbreviation: TSA, total shoulder arthroplasty.
In addition to TSA, this cohort of surgeons submitted 240 unique CPT codes with case volumes >10 annually over the 3-year study period. Of these, 80.2% (1102/1374) of surgeons performed non-arthroplasty shoulder procedures on Medicare patients, for a combined total of 202,335 procedures over the 3-year study period (Table 2). A significant proportion of these procedures were arthroscopic debridement (60,014 procedures performed by 908 surgeons) and arthroscopic rotator cuff repair (47,089 procedures performed by 809 surgeons). Just under half (49.1%; 674/1374) of surgeons performing TSA also performed TKA during this period (77,873 arthroplasties). Fewer surgeons (27.8%; 382/1374) performed total hip arthroplasty during this period (27,322 arthroplasties). Other procedure types that this group of surgeons routinely performed on Medicare patients were repairs of traumatic injuries (29.8%), non-arthroplasty knee surgeries (27.2%), elbow procedures (19.6%), and hand surgery (15.4%). By case load, non-arthroplasty shoulder procedures consisted of 43% of Medicare volume over the study period (Figure 1). Between 2012 and 2014, the average proportion of Medicare cases that were shoulder arthroplasties increased from 13.8% (21,137/152,862) to 16.7% (26,865/160,851; P = .001). Shoulder arthroplasty constituted 100% of the Medicare surgical case volume for 67 (4.9%; 67/1374) of the surgeons.
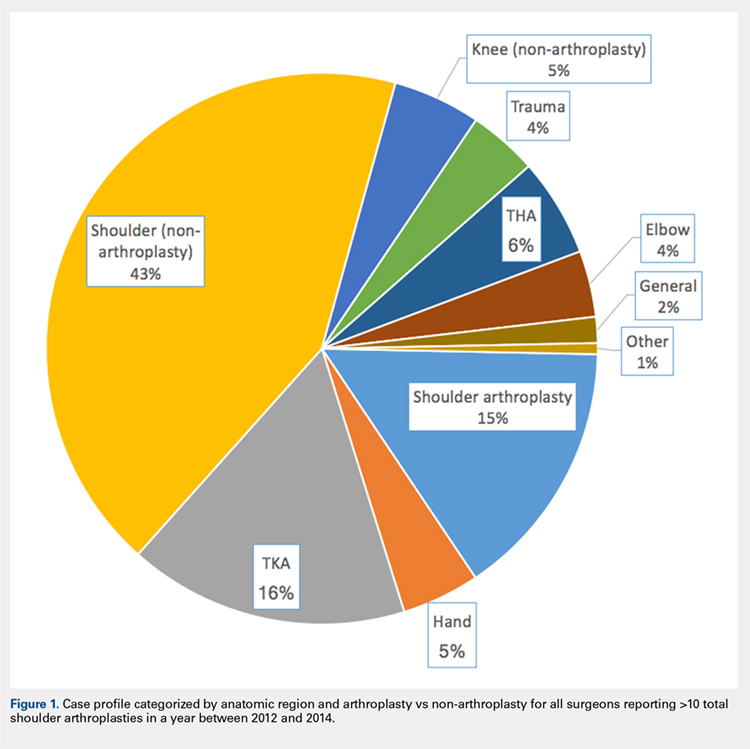
Table 2. Case Volumes over Time with All Procedures Categorized by Anatomic Region and Arthroplasty vs Non-arthroplasty*
2012 | 2013 | 2014 | Total | |
Shoulder arthroplasty | 21,351 (n=837) | 24,128 (n=984) | 26,902 (n=1,078) | 72,381 (n=1,374) |
23472: total shoulder arthroplasty | 21,137 (n=834) | 23,971 (n=984) | 26,865 (n=1,078) | 71,973 (n=1,374) |
23470: Hemiarthroplasty | 214 (n=14) | 84 (n=6) | 37 (n=2) | 335 (n=15) |
Shoulder (non-arthroplasty) | 65,947 (n=887) | 68,746 (n=942) | 67,642 (n=932) | 202,335 (n=1,102) |
29826: arthroscopic acromioplasty | 19,152 (n=724) | 20,367 (n=760) | 20,495 (n=754) | 60,014 (n=908) |
29827: arthroscopic rotator cuff repair | 14,700 (n=613) | 15,963 (n=664) | 16,426 (n=658) | 47,089 (n=809) |
23412: open rotator cuff repair | 1957 (n=88) | 2046 (n=90) | 2112 (n=2,112) | 6115 (n=143) |
23430: Open biceps tenodesis | 4063 (n=178) | 3998 (n=167) | 4601 (n=185) | 12,662 (n=288) |
29823: arthroscopic major debridement | 7428 (n=301) | 7745 (n=309) | 5202 (n=210) | 20,375 (n=417) |
Total knee arthroplasty | 25,640 (n=565) | 26,558 (n=587) | 25,675 (n=580) | 77,873 (n=637) |
Total hip arthroplasty | 8729 (n=316) | 9226 (n=318) | 9367 (n=330) | 27,322 (n=382) |
Trauma | 6454 (n=260) | 6396 (n=254) | 6364 (n=261) | 19,214 (n=410) |
27245: surgical treatment of broken thigh bone (intertrochanteric) | 2602 (n=162) | 2654 (n=164) | 2537 (n=160) | 7793 (n=274) |
27236: surgical treatment of broken thigh bone (hemiarthroplasty) | 1961 (n=123) | 1703 (n=111) | 1702 (n=112) | 5366 (n=205) |
Hand | 6343 (n=139) | 7321 (n=154) | 8006 (n=172) | 21,670 (n=211) |
Elbow | 6113 (n=198) | 6139 (n=204) | 6131 (n=198) | 18,383 (n=270) |
Knee (non-arthroplasty) | 8514 (n=275) | 8140 (n=275) | 7689 (n=230) | 24,343 (n=374) |
Outpatient visits | 879,740 (n=1,282) | 907,124 (n=1,320) | 921,291 (n=1,327) | 2,708,155 (n=1,342) |
New patient | 195,898 (n=1,276) | 192,937 (n=1,305) | 191,427 (n=1,315) | 571,203 (n=1,332) |
Existing patient | 740,307 (n=1,279) | 714,187 (n=1,316) | 729,864 (n=1,324) | 2,29,976 (n=1,338) |
* Procedures of interest with high case volumes are reported individually.
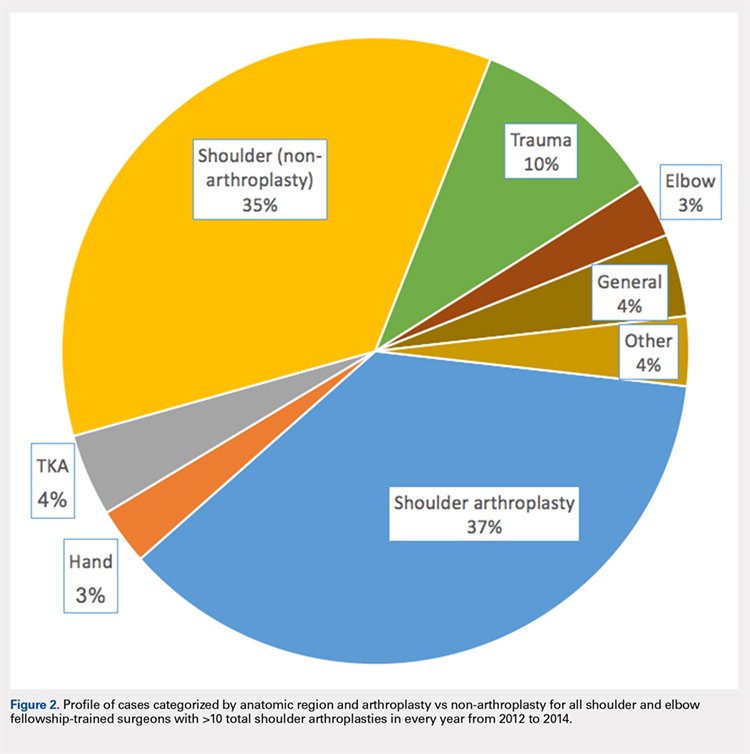
Only 44.3% (609/1374) of surgeons performed TSA in a minimum of 11 Medicare patients in all 3 years of the study period (consistent providers of TSA), providing a total of 55,538 TSA (77.2%; 55,538/71,973). When fellowship training was evaluated, 191 (31.4%; 191/609) of these surgeons were shoulder and elbow fellowship trained (21,444 TSA; 38.6%; Table 3). More than one-third (36.6%; 223/609) had completed a sports surgery fellowship (18,899 TSA; 34.0%). Surgeons trained in hand surgery (12.5%; 76/609) and adult reconstruction (5.3%; 32/610) also made contributions to meeting the TSA demand with 6971 (12.6%) and 2485 (4.5%) TSA, respectively. One-fifth of this cohort (18.1%; 110/609) had unknown fellowship training: they either reported no fellowship (13.6%; 83/609) or did not specify the type of training (4.4%; 27/609). Shoulder and elbow fellowship-trained surgeons performed more TSA (median: 89.0 TSA per surgeon between 2012 and 2014) than surgeons without shoulder and elbow fellowship training (median: 67.0 TSA per surgeon; P < 0.001). More than one-third (37%) of shoulder and elbow fellowship-trained surgeons’ surgical case volume was comprised of TSA, with an additional 35% from non-arthroplasty shoulder procedures (Figure 2). In order for the current supply of shoulder and elbow fellowship-trained surgeons to meet the Medicare TSA demand, each fellowship graduate would have to perform 140.6 TSA in Medicare patients annually. Shoulder and elbow fellowship-trained surgeons were more likely to practice in referral regions with an increased Medicare population (P < .001), an increased number of surgeons performing TSA (P < .001), and a higher proportion of Medicaid-eligible patients (P = .01; Table 4). Shoulder and elbow fellowship-trained surgeons (18.7 years post-medical school graduation) were also earlier in their careers than other consistent TSA surgeons (23.1 years post-graduation; P < .001).
Table 3. A Representation of Fellowships Among TSA Surgeons and Their Shoulder Arthroplasty Case Load*
Fellowship | Surgeons (%) | 2012-2014 (no, %) | SA Medicare Cases (%) | Average Surgeon Annual SA Volume |
Shoulder and elbow | 191 (31.4%) | 21,444 (38.6%) | 29.3% | 37.4 |
Hand surgery | 76 (12.5%) | 6971 (12.6%) | 17.1% | 30.6 |
Sports | 223 (36.6%) | 18,899 (34.0%) | 19.4% | 28.3 |
Trauma | 14 (2.3%) | 1270 (2.3%) | 10.9% | 30.2 |
Adult reconstruction | 32 (5.3%) | 2485 (4.5%) | 10.2% | 25.9 |
Unknown/none | 110 (18.1%) | 8489 (15.3%) | 16.3% | 25.7 |
|
|
|
| |
1 Fellowship | 459 (75.3%) | 42,065 (75.7%) | 20.7% | 30.5 |
≥2 Fellowships | 67 (11.0%) | 7122 (12.8%) | 22.5% | 35.4 |
* Not all fellowships (eg, oncology) included due to small numbers. Also, many surgeons performed multiple fellowships.
Abbreviations: SA, shoulder arthroplasty; TSA, total shoulder arthroplasty.
Table 4. Breakdown of Geographic Characteristics of Orthopedic Surgeons Consistently
Performing TSA between 2012 and 2014 Stratified by Fellowship Training
Abbreviations: HRR, hospital referral region; TSA, total shoulder arthroplasty.
Fellowship | Percentage in Non-Urban Area | Average No. of Other TSA Surgeons within HRR | Median Proportion of Patients Eligible for Medicaid within HRR | Average Proportion of Caucasian Patients within HRR | Average Population in Practicing Zip Code | Average No. of Medicare Beneficiaries in HRR | Average No. Years from Medical School Graduation |
Shoulder and elbow | 7.3% | 10.5 | 12.6 | 84.7 | 26,620.1 | 224,868.3 | 18.7 |
Other fellowships | 10.3% | 8.6 | 11.1 | 85.6 | 27,619.7 | 177,939.7 | 23.1 |
P value | 0.29 | <0.001 | 0.01 | 0.30 | 0.41 | <0.001 | <0.001 |
Hand surgery | 7.9% | 8.1 | 12.8 | 83.7 | 24,022.8 | 179,370.8 | 23.9 |
Sports | 11.2% | 8.9 | 11.9 | 85.6 | 28,588.9 | 185,902.4 | 21.2 |
Trauma | 21.4% | 7.7 | 13.8 | 85.5 | 20,065.9 | 170,807.6 | 25.6 |
Adult reconstruction | 6.3% | 8.7 | 12.8 | 86.9 | 26,601.5 | 173,280.1 | 22.4 |
None/unknown | 10.9% | 8.5 | 12.0 | 86.4 | 28,173.6 | 166,522.5 | 27.0 |
Continue to: DISCUSSION...
DISCUSSION
Utilization of TSA has continued to rise; however, access to this cost-effective procedure was recently demonstrated to be limited.11 In a separate analysis, we established the continued rise in use of TSA in the Medicare population, coupled with an increase in the number of surgeons routinely performing TSA.2 Multiple analyses have demonstrated the importance of high-volume surgeons and hospitals familiar with the intricacies of shoulder arthroplasty concepts in minimizing complications, improving the quality and decreasing the cost of TSA.6,10,17 Specifically, Singh and colleagues18 demonstrated from a multi-center registry that surgeons and hospitals with greater shoulder arthroplasty volumes had decreased intra-operative blood loss, operative time, and hospital length of stay. As the demand for TSA, both anatomic and reverse, continues to rise, it is imperative that the healthcare delivery system is optimized to provide the best possible care. Before we can determine whether specialized training in shoulder arthroplasty influences surgical outcomes, characteristics and training of surgeons performing TSA should be described.
The number of surgeons performing >10 TSA in the Medicare population rose significantly between 2012 and 2014 (29.3%). However, the number of TSAs per surgeon over this time period remained consistent (approximately 25 per surgeon). Furthermore, the increase in the number of surgeons performing a reportable volume of TSA by 2014 was from the addition of already active surgeons (ie, the growth in TSA was not from the addition of newly trained arthroplasty surgeons but originated from the existing orthopedic surgeon workforce). In a recently published analysis, Somerson and colleagues, 11 using this same dataset, demonstrated persistent limitations in access to high-volume TSA surgeons. In a more recent analysis, we showed that while still lacking for some patients, access to a high-volume TSA surgeon has improved significantly over the past 3 years, with 96.9% of the United States population residing within 200 kilometers of a high-volume TSA surgeon (>20 Medicare cases).2 This analysis validates those findings, with the caveat that the average annual volume per surgeon is not increasing. What remains unknown, due to limitations of this dataset, is how many surgeons are not identified because they are performing ≤10 TSA each year or are performing TSA in non-Medicare patients.
With the specialization of healthcare delivery, specifically in orthopedics, it is imperative that mechanisms for providing specialty-focused care be established. However, the proportion of their practice that surgeons dedicate to TSA was unknown. This study demonstrates that this proportion is increasing. Including non-arthroplasty procedures, more than half (58%) of the procedures performed by this surgeon cohort were shoulder-specific. Furthermore, this analysis demonstrates that surgeons performing TSA have significant case diversity, including nearly half of the cohort performing TKA. Repeated evidence has demonstrated the effect of case volume on improved outcomes following orthopedic procedures.8,19–21 The pre-existing location-based model for delivering orthopedic care supports case diversity; however, this model continues to be challenged with high-volume centers of excellence and patient travel.22–24 Hip and knee arthroplasty experienced a similar surge in demand, with a subsequent shift in care to high-volume surgeons and centers.25 Shoulder and elbow fellowship-trained surgeons would need to nearly quadruple their current Medicare TSA volume to meet the entire current demand for TSA in the Medicare population (and this does not account for TSA performed by very low-volume surgeons not included in this cohort). With increased utilization of TSA, policymakers and the orthopedic community must determine the structure of delivery (centers of excellence or medium-volume disseminated throughout the country) that is optimal.
For those surgeons consistently performing TSA over the study period, fellowship training was diverse. While the current focus in orthopedics is on case volume, research in other specialties, namely general surgery, has provided repeated evidence that surgical specialization (more so than high case volume) provides improved outcomes.26–29Furthermore, Leopold and colleagues30 demonstrated an inverse relationship between competency in performing a procedure and confidence in one’s ability to do so. In their study, educational intervention provided improved competency in the procedure. Less than one-third (29.8%) of TSA in this cohort were performed by a shoulder and elbow fellowship-trained surgeon consistently performing this procedure. Approximately another quarter (26.2%) were performed by consistent TSA surgeons trained in sports surgery. Meanwhile, 34.6% of TSA in this study cohort were performed by a surgeon who did not consistently meet the minimum threshold in all study years (16,435 TSA; 22.8%) or by a surgeon performing TSA without fellowship training (8,489 TSA; 11.8%). There has been a trend toward orthopedic subspecialty training with an increased demand for fellowship-trained surgeons.31 Despite this and the complexities of TSA, many continue to be performed by surgeons with an inconsistent volume and those without arthroplasty-specific fellowship training. The available evidence supports a push toward the fellowship-trained, high-volume TSA surgeon in providing reproducible high-quality shoulder arthroplasty care. For now, that surgeon is more likely to be earlier in his/her career and reside in large, referral-based centers surrounded by other surgeons performing TSA.
These findings must be considered in the light of the study limitations. First, this is a large publicly available database. While this type of database provides a unique opportunity to assess the geographic distributions and characteristics of orthopedic surgeons, specifically those performing TSA, it completely prevents any assessment of the relationship between these findings and quality. As such, while the reader may generate hypotheses regarding the implications of our findings on the quality of TSA delivery, the true effects cannot be determined. In the same vein, for the purpose of privacy, surgeons performing ≤10 TSA were not included in this dataset. This limitation prevents the identification of low-volume TSA surgeons. Also, it is likely that the observed increase in surgeons over time is likely a reflection of small increases in volume for surgeons already performing TSA. Lastly, a web-based search was undertaken to identify surgeons’ self-reported fellowship training. The results of this web-based search could not be validated, and it is possible that fellowship training, or the lack thereof, was mischaracterized and simply not obtainable through a web-based search. Furthermore, it is not possible to fully assess the extent of high-quality TSA training in these various fellowships.
CONCLUSION
In just the past decade, the utilization of TSA in the Medicare population has increased significantly. However, this increase was not achieved by the addition of highly specialized, high-volume surgeons but by the addition of many surgeons performing lower numbers of TSA surgeries. Furthermore, for those performing this cost-effective procedure, TSA constitutes a relatively small proportion of the surgeries they perform. Shoulder and elbow fellowship-trained surgeons currently account for a low percentage of the overall number of surgeons performing TSA. The implications of these findings must be considered and investigated.
ABSTRACT
Total shoulder arthroplasty (TSA) has proved a cost-effective, reproducible procedure for multiple shoulder pathologies. As utilization of TSA continues to grow, it is important to investigate procedure diversity, training, and other characteristics of surgeons performing TSA. To identify surgeons performing TSA in the Medicare population, the Medicare Provider Utilization and Payment Databases from 2012 through 2014 were used. This dataset includes any provider who bills Medicare >10 times with a single billing code. A web-based search was performed for each physician performing >10 TSA in all years of the study to identify their surgical training characteristics. Between 2012 and 2014, 1374 surgeons (39 females [2.8%]) performed >10 TSA in Medicare patients in at least 1 year (71,973 TSA). Only 44.3% (609/1374) of surgeons met this threshold for all 3 years (55,538 TSA). Of these 609 surgeons, 191 (31.3%) were shoulder and elbow fellowship trained (21,444 TSA). Shoulder and elbow fellowship-trained surgeons were at earlier points in their careers and practiced in large referral-based centers with other surgeons performing TSA. In addition to TSA, surgeons performed other non-arthroplasty shoulder procedures (80.2% of surgeons), total knee arthroplasty (46.3%), repairs of traumatic injuries (29.8%), total hip arthroplasty (27.8%), non-arthroplasty knee surgeries (27.2%), elbow procedures (19.6%), and hand surgery (15.4%) during the study period. With less than one-third of TSA performed by shoulder and elbow fellowship-trained surgeons with consistent moderate-volume practices, the impact of consistent high-volume practices and targeted fellowship training on quality must be determined.
Continue to: With the adoption of reverse shoulder arthroplasty...
With the adoption of reverse shoulder arthroplasty, utilization of total shoulder arthroplasty (TSA) has increased substantially over the last decade.1–3 Such increases are likely secondary to an aging population, increased comfort with the procedure, and the adoption of broadened indications for reverse shoulder arthroplasty, especially in the setting of proximal humerus fractures in the elderly.4–7 Between 2012 and 2014 alone, the number of surgeons performing >10 TSA in Medicare patients annually increased by 28.6% (824 to 1060 surgeons) providing a 26.6% (20,824-26,365 procedures) increase in national volume in the Medicare population.2 With this boom in utilization, scrutiny of this now routine procedure and those performing it is necessary.
Prior reviews have demonstrated a strong link between surgeon and hospital TSA volume and outcomes of the procedure.8–10 Somerson and colleagues11 investigated fellowship training among surgeons performing TSA in 2012 and found that only 28% had completed a shoulder and elbow fellowship. In addition to prior analyses2, 12, Somerson and colleagues confirmed a persistent geographic variation in utilization of TSA.11 In conjunction with the evolution of shoulder arthroplasty, dedicated shoulder and elbow fellowship training has expanded. With a shift toward specialization in care, nearly 90% of orthopedic surgery residents plan to pursue shoulder and elbow fellowships, comprising 4.6% of (42/897) of available positions.13
What remains unknown is the specialization of surgeons performing TSA, the regularity of their arthroplasty volume, and trends in TSA specialization over time. Therefore, this study aims to (a) identify surgeons performing shoulder arthroplasty and cohort changes over time, (b) determine the case profile of surgeons consistently performing shoulder arthroplasty, and (c) establish the characteristics of shoulder arthroplasty surgeons with a specific focus on fellowship training.
METHODS
Prior to collecting surgeon-specific data, we identified surgeons performing TSA through the Centers for Medicare and Medicaid Services’ public release of “Medicare Provider Utilization and Payment Data: Physician and Other Supplier.”14 Datasets from 2012, 2013, and 2014 were used to identify all surgeons performing >10 TSAs (Current Procedural Terminology [CPT] Code 23472) during at least 1 of those years. This dataset provides the name, identification number, address, and all billing (by volume) for each unique CPT code submitted ≥10 times in a calendar year.
Once the cohort of surgeons had been generated, the number of surgeons consistently performing TSA year-over-year was determined. This allowed for an analysis of the consistency with which surgeons are performing moderate- to high-volume TSA. To form a case profile of surgeons performing TSA and observe how this shifted over time, a count and a description of each CPT code submitted by each surgeon was identified. To maintain patient privacy, only those claims made >10 times are reported for a provider (both physicians and physician-extenders are included in this dataset). First, all CPT codes were reviewed and tagged as surgical or non-surgical events. Then, every procedural CPT code identified was reviewed and categorized based upon anatomic location and procedure (eg, total knee arthroplasty [TKA]). It is important to note that all claims in this dataset are limited to those patients participating in Medicare’s fee-for-service program.
Specialization was defined as the number of categorized procedures as a percentage of all procedures performed on Medicare patients. The trends for national, regional, and individual specialization of TSA, arthroplasty (major joint), and shoulder procedures were determined.
Continue to: To investigate the characteristics of surgeons...
To investigate the characteristics of surgeons consistently performing TSA, all surgeons performing a minimum of 11 TSA in Medicare fee-for-service beneficiaries in all years between 2012 and 2014 were identified. Such surgeons were defined as consistent TSA surgeons. Investigation of this cohort included a web-based search of their self-reported post-graduate fellowship training and year of graduation from medical school. Using these data, the percentage of surgeons performing TSA who underwent formal shoulder and elbow training was determined. In addition, the impact of fellowship training on shoulder specialization and practice location was determined. Surgeons who had completed multiple fellowships were categorized under all of them. As such, there may be some duplication of surgeons in the comparisons. In addition, other potential characteristics of shoulder and elbow fellowship-trained surgeons were investigated: number of regional shoulder surgeons, urban area, total number of Medicare beneficiaries, average reimbursement for TSA, ethnicity of Medicare beneficiaries, and percentage of Medicare patients eligible for Medicaid. Geographic regions were defined by the Dartmouth Atlas and assigned by hospital referral region.15 These defined regions were used to assess the beneficiaries (number and characteristics) that individual surgeons were likely serving. The United States Census Bureau characterization of zip code-based regions as urban areas (population >50,000), urban clusters (2500 to 50,000), and rural region (<2500) was used to categorize practice location.16
Descriptive statistics were used initially to report these findings. To analyze predictors of utilization and specialization, comparative statistics were undertaken. For comparison of binomial variables between groups, a χ2analysis was utilized. For continuous variables, data normality was assessed. A skewness and kurtosis <2 and 12, respectively, was considered to represent parametric data. For parametric data, the mean was reported; conversely, the median is reported for non-parametric data. To assess continuous variables between groups, a t test or a Wilcoxon rank-sum test was used for parametric and non-parametric distributions, respectively.
RESULTS
Between 2012 and 2014, 1374 surgeons (39 females [2.8%]) performed >10 TSA in Medicare patients in at least 1 year, for a combined total of 71,973 TSAs (Table 1). In 2012, only 834 surgeons (13 females [1.6%]) performed a minimum of 10 TSA in Medicare patients (21,137 arthroplasties; 25.3 per surgeon). This increased to 1078 surgeons (33 females [3.1%]; P = .04) performing 26,865 TSA (24.92 per surgeon) in 2014. Utilization of non-physician assistants in TSA also increased significantly over this period, with 307 assisting in 6885 TSAs (22.4 per provider) in 2012 and 465 assisting 10,433 TSA (22.4 per provider) in 2014. When all procedures were considered, including those performed at outpatient visits, 1319 physicians (95.9% of cohort) were active in 2012—providing either surgical procedures or outpatient consults to the Medicare population. Yet, only 63.2% performed >10 TSA in Medicare patients. The number of active surgeons performing TSA increased to 79.6% (1078/1353) in 2014 (P < .001).
Table 1. Trends in the Number of Providers Performing TSA between 2012 and 2014*
2012 | 2013 | 2014 | Total | |
Providers (no.) | 1141 | 1373 | 1543 | 1994 |
Physicians | 834 | 984 | 1,078 | 1,374 |
Non-physicians | 307 | 389 | 465 | 620 |
TSA (no.) | 28,022 | 32,641 | 37,298 | 97,961 |
Physicians | 21,137 | 23,971 | 26,865 | 71,973 |
Non-physicians | 6,885 | 8,670 | 10,433 | 25,988 |
TSA per provider | 24.5 | 23.8 | 24.2 | 49.2 |
Physicians | 25.3 | 24.3 | 24.9 | 52.4 |
Non-physicians | 22.4 | 22.3 | 22.4 | 41.2 |
Procedures (no.) | 210,845 | 224,123 | 227,305 | 662,273 |
Physicians | 152,862 | 160,114 | 160,851 | 473,827 |
Non-physicians | 57,983 | 64,009 | 66,454 | 188,446 |
Procedure per provider | 114.4 | 116.8 | 116.5 | 332.13 |
Physicians | 115.9 | 118.9 | 118.9 | 344.9 |
Non-physicians | 110.7 | 111.9 | 111.1 | 303.9 |
Active providers (no.) | 1843 | 1919 | 1951 | 1994 |
Physicians | 1319 | 1347 | 1353 | 1374 |
Non-physicians | 524 | 572 | 598 | 620 |
* Included are the number of arthroplasties and total procedures over time among this cohort. The number of active providers, determined by billing Medicare for office or surgical procedures within that year, is reported.
Abbreviation: TSA, total shoulder arthroplasty.
In addition to TSA, this cohort of surgeons submitted 240 unique CPT codes with case volumes >10 annually over the 3-year study period. Of these, 80.2% (1102/1374) of surgeons performed non-arthroplasty shoulder procedures on Medicare patients, for a combined total of 202,335 procedures over the 3-year study period (Table 2). A significant proportion of these procedures were arthroscopic debridement (60,014 procedures performed by 908 surgeons) and arthroscopic rotator cuff repair (47,089 procedures performed by 809 surgeons). Just under half (49.1%; 674/1374) of surgeons performing TSA also performed TKA during this period (77,873 arthroplasties). Fewer surgeons (27.8%; 382/1374) performed total hip arthroplasty during this period (27,322 arthroplasties). Other procedure types that this group of surgeons routinely performed on Medicare patients were repairs of traumatic injuries (29.8%), non-arthroplasty knee surgeries (27.2%), elbow procedures (19.6%), and hand surgery (15.4%). By case load, non-arthroplasty shoulder procedures consisted of 43% of Medicare volume over the study period (Figure 1). Between 2012 and 2014, the average proportion of Medicare cases that were shoulder arthroplasties increased from 13.8% (21,137/152,862) to 16.7% (26,865/160,851; P = .001). Shoulder arthroplasty constituted 100% of the Medicare surgical case volume for 67 (4.9%; 67/1374) of the surgeons.
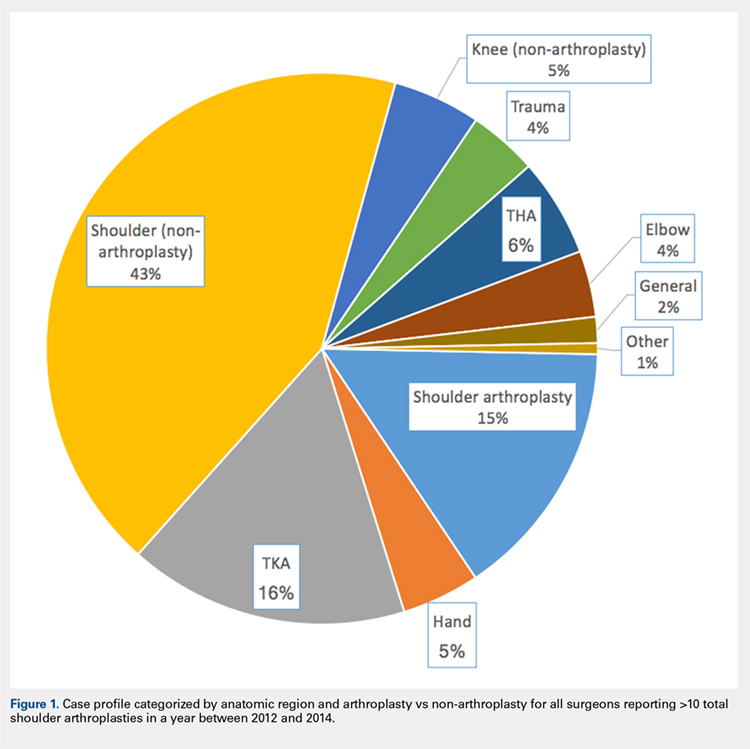
Table 2. Case Volumes over Time with All Procedures Categorized by Anatomic Region and Arthroplasty vs Non-arthroplasty*
2012 | 2013 | 2014 | Total | |
Shoulder arthroplasty | 21,351 (n=837) | 24,128 (n=984) | 26,902 (n=1,078) | 72,381 (n=1,374) |
23472: total shoulder arthroplasty | 21,137 (n=834) | 23,971 (n=984) | 26,865 (n=1,078) | 71,973 (n=1,374) |
23470: Hemiarthroplasty | 214 (n=14) | 84 (n=6) | 37 (n=2) | 335 (n=15) |
Shoulder (non-arthroplasty) | 65,947 (n=887) | 68,746 (n=942) | 67,642 (n=932) | 202,335 (n=1,102) |
29826: arthroscopic acromioplasty | 19,152 (n=724) | 20,367 (n=760) | 20,495 (n=754) | 60,014 (n=908) |
29827: arthroscopic rotator cuff repair | 14,700 (n=613) | 15,963 (n=664) | 16,426 (n=658) | 47,089 (n=809) |
23412: open rotator cuff repair | 1957 (n=88) | 2046 (n=90) | 2112 (n=2,112) | 6115 (n=143) |
23430: Open biceps tenodesis | 4063 (n=178) | 3998 (n=167) | 4601 (n=185) | 12,662 (n=288) |
29823: arthroscopic major debridement | 7428 (n=301) | 7745 (n=309) | 5202 (n=210) | 20,375 (n=417) |
Total knee arthroplasty | 25,640 (n=565) | 26,558 (n=587) | 25,675 (n=580) | 77,873 (n=637) |
Total hip arthroplasty | 8729 (n=316) | 9226 (n=318) | 9367 (n=330) | 27,322 (n=382) |
Trauma | 6454 (n=260) | 6396 (n=254) | 6364 (n=261) | 19,214 (n=410) |
27245: surgical treatment of broken thigh bone (intertrochanteric) | 2602 (n=162) | 2654 (n=164) | 2537 (n=160) | 7793 (n=274) |
27236: surgical treatment of broken thigh bone (hemiarthroplasty) | 1961 (n=123) | 1703 (n=111) | 1702 (n=112) | 5366 (n=205) |
Hand | 6343 (n=139) | 7321 (n=154) | 8006 (n=172) | 21,670 (n=211) |
Elbow | 6113 (n=198) | 6139 (n=204) | 6131 (n=198) | 18,383 (n=270) |
Knee (non-arthroplasty) | 8514 (n=275) | 8140 (n=275) | 7689 (n=230) | 24,343 (n=374) |
Outpatient visits | 879,740 (n=1,282) | 907,124 (n=1,320) | 921,291 (n=1,327) | 2,708,155 (n=1,342) |
New patient | 195,898 (n=1,276) | 192,937 (n=1,305) | 191,427 (n=1,315) | 571,203 (n=1,332) |
Existing patient | 740,307 (n=1,279) | 714,187 (n=1,316) | 729,864 (n=1,324) | 2,29,976 (n=1,338) |
* Procedures of interest with high case volumes are reported individually.
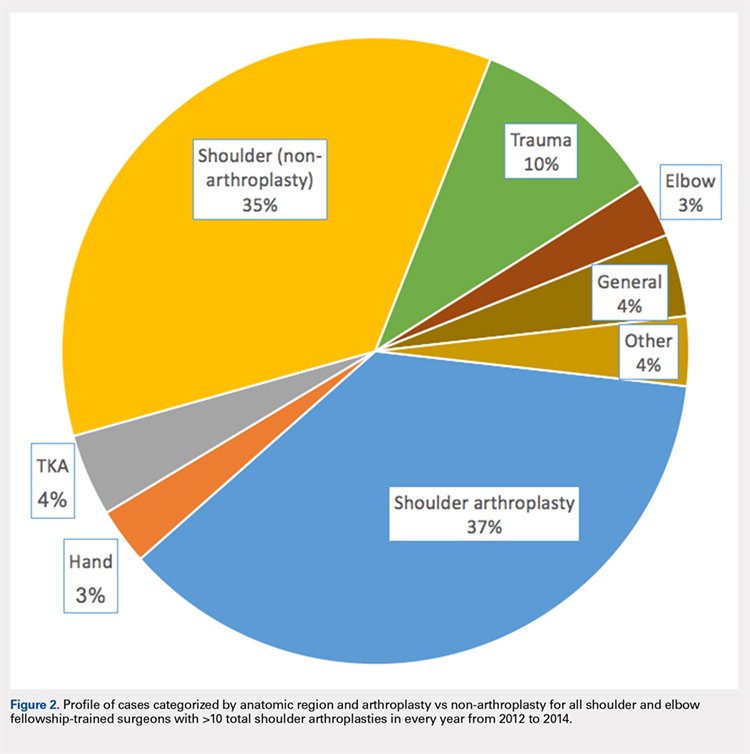
Only 44.3% (609/1374) of surgeons performed TSA in a minimum of 11 Medicare patients in all 3 years of the study period (consistent providers of TSA), providing a total of 55,538 TSA (77.2%; 55,538/71,973). When fellowship training was evaluated, 191 (31.4%; 191/609) of these surgeons were shoulder and elbow fellowship trained (21,444 TSA; 38.6%; Table 3). More than one-third (36.6%; 223/609) had completed a sports surgery fellowship (18,899 TSA; 34.0%). Surgeons trained in hand surgery (12.5%; 76/609) and adult reconstruction (5.3%; 32/610) also made contributions to meeting the TSA demand with 6971 (12.6%) and 2485 (4.5%) TSA, respectively. One-fifth of this cohort (18.1%; 110/609) had unknown fellowship training: they either reported no fellowship (13.6%; 83/609) or did not specify the type of training (4.4%; 27/609). Shoulder and elbow fellowship-trained surgeons performed more TSA (median: 89.0 TSA per surgeon between 2012 and 2014) than surgeons without shoulder and elbow fellowship training (median: 67.0 TSA per surgeon; P < 0.001). More than one-third (37%) of shoulder and elbow fellowship-trained surgeons’ surgical case volume was comprised of TSA, with an additional 35% from non-arthroplasty shoulder procedures (Figure 2). In order for the current supply of shoulder and elbow fellowship-trained surgeons to meet the Medicare TSA demand, each fellowship graduate would have to perform 140.6 TSA in Medicare patients annually. Shoulder and elbow fellowship-trained surgeons were more likely to practice in referral regions with an increased Medicare population (P < .001), an increased number of surgeons performing TSA (P < .001), and a higher proportion of Medicaid-eligible patients (P = .01; Table 4). Shoulder and elbow fellowship-trained surgeons (18.7 years post-medical school graduation) were also earlier in their careers than other consistent TSA surgeons (23.1 years post-graduation; P < .001).
Table 3. A Representation of Fellowships Among TSA Surgeons and Their Shoulder Arthroplasty Case Load*
Fellowship | Surgeons (%) | 2012-2014 (no, %) | SA Medicare Cases (%) | Average Surgeon Annual SA Volume |
Shoulder and elbow | 191 (31.4%) | 21,444 (38.6%) | 29.3% | 37.4 |
Hand surgery | 76 (12.5%) | 6971 (12.6%) | 17.1% | 30.6 |
Sports | 223 (36.6%) | 18,899 (34.0%) | 19.4% | 28.3 |
Trauma | 14 (2.3%) | 1270 (2.3%) | 10.9% | 30.2 |
Adult reconstruction | 32 (5.3%) | 2485 (4.5%) | 10.2% | 25.9 |
Unknown/none | 110 (18.1%) | 8489 (15.3%) | 16.3% | 25.7 |
|
|
|
| |
1 Fellowship | 459 (75.3%) | 42,065 (75.7%) | 20.7% | 30.5 |
≥2 Fellowships | 67 (11.0%) | 7122 (12.8%) | 22.5% | 35.4 |
* Not all fellowships (eg, oncology) included due to small numbers. Also, many surgeons performed multiple fellowships.
Abbreviations: SA, shoulder arthroplasty; TSA, total shoulder arthroplasty.
Table 4. Breakdown of Geographic Characteristics of Orthopedic Surgeons Consistently
Performing TSA between 2012 and 2014 Stratified by Fellowship Training
Abbreviations: HRR, hospital referral region; TSA, total shoulder arthroplasty.
Fellowship | Percentage in Non-Urban Area | Average No. of Other TSA Surgeons within HRR | Median Proportion of Patients Eligible for Medicaid within HRR | Average Proportion of Caucasian Patients within HRR | Average Population in Practicing Zip Code | Average No. of Medicare Beneficiaries in HRR | Average No. Years from Medical School Graduation |
Shoulder and elbow | 7.3% | 10.5 | 12.6 | 84.7 | 26,620.1 | 224,868.3 | 18.7 |
Other fellowships | 10.3% | 8.6 | 11.1 | 85.6 | 27,619.7 | 177,939.7 | 23.1 |
P value | 0.29 | <0.001 | 0.01 | 0.30 | 0.41 | <0.001 | <0.001 |
Hand surgery | 7.9% | 8.1 | 12.8 | 83.7 | 24,022.8 | 179,370.8 | 23.9 |
Sports | 11.2% | 8.9 | 11.9 | 85.6 | 28,588.9 | 185,902.4 | 21.2 |
Trauma | 21.4% | 7.7 | 13.8 | 85.5 | 20,065.9 | 170,807.6 | 25.6 |
Adult reconstruction | 6.3% | 8.7 | 12.8 | 86.9 | 26,601.5 | 173,280.1 | 22.4 |
None/unknown | 10.9% | 8.5 | 12.0 | 86.4 | 28,173.6 | 166,522.5 | 27.0 |
Continue to: DISCUSSION...
DISCUSSION
Utilization of TSA has continued to rise; however, access to this cost-effective procedure was recently demonstrated to be limited.11 In a separate analysis, we established the continued rise in use of TSA in the Medicare population, coupled with an increase in the number of surgeons routinely performing TSA.2 Multiple analyses have demonstrated the importance of high-volume surgeons and hospitals familiar with the intricacies of shoulder arthroplasty concepts in minimizing complications, improving the quality and decreasing the cost of TSA.6,10,17 Specifically, Singh and colleagues18 demonstrated from a multi-center registry that surgeons and hospitals with greater shoulder arthroplasty volumes had decreased intra-operative blood loss, operative time, and hospital length of stay. As the demand for TSA, both anatomic and reverse, continues to rise, it is imperative that the healthcare delivery system is optimized to provide the best possible care. Before we can determine whether specialized training in shoulder arthroplasty influences surgical outcomes, characteristics and training of surgeons performing TSA should be described.
The number of surgeons performing >10 TSA in the Medicare population rose significantly between 2012 and 2014 (29.3%). However, the number of TSAs per surgeon over this time period remained consistent (approximately 25 per surgeon). Furthermore, the increase in the number of surgeons performing a reportable volume of TSA by 2014 was from the addition of already active surgeons (ie, the growth in TSA was not from the addition of newly trained arthroplasty surgeons but originated from the existing orthopedic surgeon workforce). In a recently published analysis, Somerson and colleagues, 11 using this same dataset, demonstrated persistent limitations in access to high-volume TSA surgeons. In a more recent analysis, we showed that while still lacking for some patients, access to a high-volume TSA surgeon has improved significantly over the past 3 years, with 96.9% of the United States population residing within 200 kilometers of a high-volume TSA surgeon (>20 Medicare cases).2 This analysis validates those findings, with the caveat that the average annual volume per surgeon is not increasing. What remains unknown, due to limitations of this dataset, is how many surgeons are not identified because they are performing ≤10 TSA each year or are performing TSA in non-Medicare patients.
With the specialization of healthcare delivery, specifically in orthopedics, it is imperative that mechanisms for providing specialty-focused care be established. However, the proportion of their practice that surgeons dedicate to TSA was unknown. This study demonstrates that this proportion is increasing. Including non-arthroplasty procedures, more than half (58%) of the procedures performed by this surgeon cohort were shoulder-specific. Furthermore, this analysis demonstrates that surgeons performing TSA have significant case diversity, including nearly half of the cohort performing TKA. Repeated evidence has demonstrated the effect of case volume on improved outcomes following orthopedic procedures.8,19–21 The pre-existing location-based model for delivering orthopedic care supports case diversity; however, this model continues to be challenged with high-volume centers of excellence and patient travel.22–24 Hip and knee arthroplasty experienced a similar surge in demand, with a subsequent shift in care to high-volume surgeons and centers.25 Shoulder and elbow fellowship-trained surgeons would need to nearly quadruple their current Medicare TSA volume to meet the entire current demand for TSA in the Medicare population (and this does not account for TSA performed by very low-volume surgeons not included in this cohort). With increased utilization of TSA, policymakers and the orthopedic community must determine the structure of delivery (centers of excellence or medium-volume disseminated throughout the country) that is optimal.
For those surgeons consistently performing TSA over the study period, fellowship training was diverse. While the current focus in orthopedics is on case volume, research in other specialties, namely general surgery, has provided repeated evidence that surgical specialization (more so than high case volume) provides improved outcomes.26–29Furthermore, Leopold and colleagues30 demonstrated an inverse relationship between competency in performing a procedure and confidence in one’s ability to do so. In their study, educational intervention provided improved competency in the procedure. Less than one-third (29.8%) of TSA in this cohort were performed by a shoulder and elbow fellowship-trained surgeon consistently performing this procedure. Approximately another quarter (26.2%) were performed by consistent TSA surgeons trained in sports surgery. Meanwhile, 34.6% of TSA in this study cohort were performed by a surgeon who did not consistently meet the minimum threshold in all study years (16,435 TSA; 22.8%) or by a surgeon performing TSA without fellowship training (8,489 TSA; 11.8%). There has been a trend toward orthopedic subspecialty training with an increased demand for fellowship-trained surgeons.31 Despite this and the complexities of TSA, many continue to be performed by surgeons with an inconsistent volume and those without arthroplasty-specific fellowship training. The available evidence supports a push toward the fellowship-trained, high-volume TSA surgeon in providing reproducible high-quality shoulder arthroplasty care. For now, that surgeon is more likely to be earlier in his/her career and reside in large, referral-based centers surrounded by other surgeons performing TSA.
These findings must be considered in the light of the study limitations. First, this is a large publicly available database. While this type of database provides a unique opportunity to assess the geographic distributions and characteristics of orthopedic surgeons, specifically those performing TSA, it completely prevents any assessment of the relationship between these findings and quality. As such, while the reader may generate hypotheses regarding the implications of our findings on the quality of TSA delivery, the true effects cannot be determined. In the same vein, for the purpose of privacy, surgeons performing ≤10 TSA were not included in this dataset. This limitation prevents the identification of low-volume TSA surgeons. Also, it is likely that the observed increase in surgeons over time is likely a reflection of small increases in volume for surgeons already performing TSA. Lastly, a web-based search was undertaken to identify surgeons’ self-reported fellowship training. The results of this web-based search could not be validated, and it is possible that fellowship training, or the lack thereof, was mischaracterized and simply not obtainable through a web-based search. Furthermore, it is not possible to fully assess the extent of high-quality TSA training in these various fellowships.
CONCLUSION
In just the past decade, the utilization of TSA in the Medicare population has increased significantly. However, this increase was not achieved by the addition of highly specialized, high-volume surgeons but by the addition of many surgeons performing lower numbers of TSA surgeries. Furthermore, for those performing this cost-effective procedure, TSA constitutes a relatively small proportion of the surgeries they perform. Shoulder and elbow fellowship-trained surgeons currently account for a low percentage of the overall number of surgeons performing TSA. The implications of these findings must be considered and investigated.
1. Kim SH, Wise BL, Zhang Y, Szabo RM. Increasing incidence of shoulder arthroplasty in the United States. J Bone Joint Surg Am. 2011;93(24):2249-2254. doi:10.2106/JBJS.J.01994.
2. Zmistowski B, Padegimas EM, Howley M, Abboud J, Williams G, Namdari S. Trends and Variability in the Use of Total Shoulder Arthroplasty for Medicare Patients. J Am Acad Orthop Surg. 2018;26(4):133-141. doi:10.5435/JAAOS-D-16-00720
3. Day JS, Lau E, Ong KL, Williams GR, Ramsey ML, Kurtz SM. Prevalence and projections of total shoulder and elbow arthroplasty in the United States to 2015. J Shoulder Elbow Surg. 2010;19(8):1115-1120. doi: http://www.jshoulderelbow.org/article/S1058-2746(10)00110-2/abstract.
4. Day JS, Paxton ES, Lau E, Gordon VA, Abboud JA, Williams GR. Use of reverse total shoulder arthroplasty in the Medicare population. J Shoulder Elbow Surg. 2015;24(5):766-772. doi:10.1016/j.jse.2014.12.023.
5. Schairer WW, Nwachukwu BU, Lyman S, Craig EV, Gulotta LV. National utilization of reverse total shoulder arthroplasty in the United States. J Shoulder Elbow Surg. 2015;24(1):91-97. doi:10.1016/j.jse.2014.08.026.
6. Westermann RW, Pugely AJ, Martin CT, Gao Y, Wolf BR, Hettrich CM. Reverse shoulder arthroplasty in the United States: A comparison of national volume, patient demographics, complications, and surgical indications. Iowa Orthop J. 2015;35:1-7.
7. Acevedo DC, Mann T, Abboud JA, Getz C, Baumhauer JF, Voloshin I. Reverse total shoulder arthroplasty for the treatment of proximal humeral fractures: patterns of use among newly trained orthopedic surgeons. J Shoulder Elbow Surg. 2014;23(9):1363-1367. doi: http://www.jshoulderelbow.org/article/S1058-2746(14)00036-6/abstract.
8. Hammond JW, Queale WS, Kim TK, McFarland EG. Surgeon experience and clinical and economic outcomes for shoulder arthroplasty. J Bone Joint Surg Am. 2003;85-A(12):2318-2324.
9. Jain NB, Kuye I, Higgins LD, Warner JJP. Surgeon volume is associated with cost and variation in surgical treatment of proximal humeral fractures. Clin Orthop Relat Res. 2012;471(2):655-664. doi:10.1007/s11999-012-2481-6.
10. Lyman S, Jones EC, Bach PB, Peterson MGE, Marx RG. The association between hospital volume and total shoulder arthroplasty outcomes. Clin Orthop Relat Res. 2005;(432):132-137. doi:10.1097/01.blo.0000150571.51381.9a.
11. Somerson JS, Stein BA, Wirth MA. Distribution of high-volume shoulder arthroplasty surgeons in the United States: Data from the 2014 Medicare provider utilization and payment data release. J Bone Joint Surg Am. 2016;98(18):e77. doi:10.2106/JBJS.15.00776.
12. Fisher ES, Bell J-E, Tomek IM, Esty AR, Goodman DC. Trends and regional variation in hip, knee, and shoulder Replacement. Atlases and Reports. Dartmouth Atlas of Health Care. https://www.dartmouthatlas.org/atlases-and-reports/. Accessed December 14, 2018.
13. Daniels AH, DiGiovanni CW. Is subspecialty fellowship training emerging as a necessary component of contemporary orthopaedic surgery education? J Grad Med Educ. 2014;6(2):218-221. doi:10.4300/JGME-D-14-00120.1.
14. Department of Health and Human Services, Centers for Medicare and Medicaid Services. Physician and other supplier Data 2012 CY 2012. https://www.cms.gov/Research-Statistics-Data-and-Systems/Statistics-Tren.... Published October 5, 2015. Accessed July 25, 2016.
15. The Dartmouth Institute for Health Policy and Clinical Practice. Dartmouth Atlas of Health Care. Understanding the Efficiency and Effectiveness of the Health Care System. http://www.dartmouthatlas.org/. Accessed January 31, 2014.
16. United States Department of Commerce. United States Census Bureau. 2010 Census Urban and Rural Classification and Urban Area Criteria. https://www.census.gov/geo/reference/ua/urban-rural-2010.html. Accessed September 30, 2016.
17. Kempton LB, Ankerson E, Wiater JM. A complication-based learning curve from 200 reverse shoulder arthroplasties. Clin Orthop Relat Res. 2011;469(9):2496-2504. doi:10.1007/s11999-011-1811-4.
18. Singh A, Yian EH, Dillon MT, Takayanagi M, Burke MF, Navarro RA. The effect of surgeon and hospital volume on shoulder arthroplasty perioperative quality metrics. J Bone Joint Surg Am. 2014;23(8):1187-1194. doi:10.1016/j.jse.2013.11.017.
19. Jain N, Pietrobon R, Hocker S, Guller U, Shankar A, Higgins LD. The relationship between surgeon and hospital volume and outcomes for shoulder arthroplasty. J Bone Joint Surg Am. 2004;86(3):496-505.
20. Taylor HD, Dennis DA, Crane HS. Relationship between mortality rates and hospital patient volume for Medicare patients undergoing major orthopaedic surgery of the hip, knee, spine, and femur. J Arthroplasty. 1997;12(3):235-242. doi:10.1016/S0883-5403(97)90018-8.
21. Walch G, Bacle G, Lädermann A, Nové-Josserand L, Smithers CJ. Do the indications, results, and complications of reverse shoulder arthroplasty change with surgeon’s experience? J Bone Joint Surg Am. 2012;21(11):1470-1477. doi:10.1016/j.jse.2011.11.010.
22. FitzGerald JD, Soohoo NF, Losina E, Katz JN. Potential impact on patient residence to hospital travel distance and access to care under a policy of preferential referral to high-volume knee replacement hospitals. Arthritis Care Res. 2012;64(6):890-897. doi:10.1002/acr.21611.
23. Maradit Kremers H, Salduz A, Schleck CD, Larson DR, Berry DJ, Lewallen DG. Referral bias in primary total knee arthroplasty: retrospective analysis of 22,614 surgeries in a tertiary referral center. J Arthroplasty. doi:10.1016/j.arth.2016.08.014.
24. Robinson JC, MacPherson K. Payers test reference pricing and centers of excellence to steer patients to low-price and high-quality providers. Health Affairs. 2012;31(9):2028-2036. doi: 10.1377/hlthaff.2011.1313
25. Laucis NC, Chowdhury M, Dasgupta A, Bhattacharyya T. Trend toward high-volume hospitals and the influence on complications in knee and hip arthroplasty. J Bone Joint Surg Am. 2016;98(9):707-712. doi:10.2106/JBJS.15.00399.
26. Anwar S, Fraser S, Hill J. Surgical specialization and training–its relation to clinical outcome for colorectal cancer surgery. J Eval Clin Pract. 2012;18(1):5-11. doi:10.1111/j.1365-2753.2010.01525.x.
27. Snow BW, Catwright PC, Young MD. Does surgical subspecialization in pediatrics provide high-quality, cost-effective patient care? Pediatrics. 1996;97(1):14-17.
28. Smith J a. E, King PM, Lane RHS, Thompson MR. Evidence of the effect of ‘specialization’ on the management, surgical outcome and survival from colorectal cancer in Wessex. Br J Surg. 2003;90(5):583-592. doi:10.1002/bjs.4085.
29. Hall BL, Hsaio EY, Majercik S, Hirbe M, Hamilton BH. The impact of surgeon specialization on patient mortality: Examination of a continuous Herfindahl-Hirschman Index. Ann Surg. 2009;249(5):708-716. doi: 10.1097/SLA.0b013e3181a335f8.
30. Leopold SS, Morgan HD, Kadel NJ, Gardner GC, Schaad DC, Wolf FM. Impact of educational intervention on confidence and competence in the performance of a simple surgical task. J Bone Joint Surg Am. 2005;87(5):1031-1037. doi:10.2106/JBJS.D.02434.
31. Morrell NT, Mercer DM, Moneim MS. Trends in the orthopedic job market and the importance of fellowship subspecialty training. Orthopedics. 2012;35(4):e555-e560. doi:10.3928/01477447-20120327-13.
1. Kim SH, Wise BL, Zhang Y, Szabo RM. Increasing incidence of shoulder arthroplasty in the United States. J Bone Joint Surg Am. 2011;93(24):2249-2254. doi:10.2106/JBJS.J.01994.
2. Zmistowski B, Padegimas EM, Howley M, Abboud J, Williams G, Namdari S. Trends and Variability in the Use of Total Shoulder Arthroplasty for Medicare Patients. J Am Acad Orthop Surg. 2018;26(4):133-141. doi:10.5435/JAAOS-D-16-00720
3. Day JS, Lau E, Ong KL, Williams GR, Ramsey ML, Kurtz SM. Prevalence and projections of total shoulder and elbow arthroplasty in the United States to 2015. J Shoulder Elbow Surg. 2010;19(8):1115-1120. doi: http://www.jshoulderelbow.org/article/S1058-2746(10)00110-2/abstract.
4. Day JS, Paxton ES, Lau E, Gordon VA, Abboud JA, Williams GR. Use of reverse total shoulder arthroplasty in the Medicare population. J Shoulder Elbow Surg. 2015;24(5):766-772. doi:10.1016/j.jse.2014.12.023.
5. Schairer WW, Nwachukwu BU, Lyman S, Craig EV, Gulotta LV. National utilization of reverse total shoulder arthroplasty in the United States. J Shoulder Elbow Surg. 2015;24(1):91-97. doi:10.1016/j.jse.2014.08.026.
6. Westermann RW, Pugely AJ, Martin CT, Gao Y, Wolf BR, Hettrich CM. Reverse shoulder arthroplasty in the United States: A comparison of national volume, patient demographics, complications, and surgical indications. Iowa Orthop J. 2015;35:1-7.
7. Acevedo DC, Mann T, Abboud JA, Getz C, Baumhauer JF, Voloshin I. Reverse total shoulder arthroplasty for the treatment of proximal humeral fractures: patterns of use among newly trained orthopedic surgeons. J Shoulder Elbow Surg. 2014;23(9):1363-1367. doi: http://www.jshoulderelbow.org/article/S1058-2746(14)00036-6/abstract.
8. Hammond JW, Queale WS, Kim TK, McFarland EG. Surgeon experience and clinical and economic outcomes for shoulder arthroplasty. J Bone Joint Surg Am. 2003;85-A(12):2318-2324.
9. Jain NB, Kuye I, Higgins LD, Warner JJP. Surgeon volume is associated with cost and variation in surgical treatment of proximal humeral fractures. Clin Orthop Relat Res. 2012;471(2):655-664. doi:10.1007/s11999-012-2481-6.
10. Lyman S, Jones EC, Bach PB, Peterson MGE, Marx RG. The association between hospital volume and total shoulder arthroplasty outcomes. Clin Orthop Relat Res. 2005;(432):132-137. doi:10.1097/01.blo.0000150571.51381.9a.
11. Somerson JS, Stein BA, Wirth MA. Distribution of high-volume shoulder arthroplasty surgeons in the United States: Data from the 2014 Medicare provider utilization and payment data release. J Bone Joint Surg Am. 2016;98(18):e77. doi:10.2106/JBJS.15.00776.
12. Fisher ES, Bell J-E, Tomek IM, Esty AR, Goodman DC. Trends and regional variation in hip, knee, and shoulder Replacement. Atlases and Reports. Dartmouth Atlas of Health Care. https://www.dartmouthatlas.org/atlases-and-reports/. Accessed December 14, 2018.
13. Daniels AH, DiGiovanni CW. Is subspecialty fellowship training emerging as a necessary component of contemporary orthopaedic surgery education? J Grad Med Educ. 2014;6(2):218-221. doi:10.4300/JGME-D-14-00120.1.
14. Department of Health and Human Services, Centers for Medicare and Medicaid Services. Physician and other supplier Data 2012 CY 2012. https://www.cms.gov/Research-Statistics-Data-and-Systems/Statistics-Tren.... Published October 5, 2015. Accessed July 25, 2016.
15. The Dartmouth Institute for Health Policy and Clinical Practice. Dartmouth Atlas of Health Care. Understanding the Efficiency and Effectiveness of the Health Care System. http://www.dartmouthatlas.org/. Accessed January 31, 2014.
16. United States Department of Commerce. United States Census Bureau. 2010 Census Urban and Rural Classification and Urban Area Criteria. https://www.census.gov/geo/reference/ua/urban-rural-2010.html. Accessed September 30, 2016.
17. Kempton LB, Ankerson E, Wiater JM. A complication-based learning curve from 200 reverse shoulder arthroplasties. Clin Orthop Relat Res. 2011;469(9):2496-2504. doi:10.1007/s11999-011-1811-4.
18. Singh A, Yian EH, Dillon MT, Takayanagi M, Burke MF, Navarro RA. The effect of surgeon and hospital volume on shoulder arthroplasty perioperative quality metrics. J Bone Joint Surg Am. 2014;23(8):1187-1194. doi:10.1016/j.jse.2013.11.017.
19. Jain N, Pietrobon R, Hocker S, Guller U, Shankar A, Higgins LD. The relationship between surgeon and hospital volume and outcomes for shoulder arthroplasty. J Bone Joint Surg Am. 2004;86(3):496-505.
20. Taylor HD, Dennis DA, Crane HS. Relationship between mortality rates and hospital patient volume for Medicare patients undergoing major orthopaedic surgery of the hip, knee, spine, and femur. J Arthroplasty. 1997;12(3):235-242. doi:10.1016/S0883-5403(97)90018-8.
21. Walch G, Bacle G, Lädermann A, Nové-Josserand L, Smithers CJ. Do the indications, results, and complications of reverse shoulder arthroplasty change with surgeon’s experience? J Bone Joint Surg Am. 2012;21(11):1470-1477. doi:10.1016/j.jse.2011.11.010.
22. FitzGerald JD, Soohoo NF, Losina E, Katz JN. Potential impact on patient residence to hospital travel distance and access to care under a policy of preferential referral to high-volume knee replacement hospitals. Arthritis Care Res. 2012;64(6):890-897. doi:10.1002/acr.21611.
23. Maradit Kremers H, Salduz A, Schleck CD, Larson DR, Berry DJ, Lewallen DG. Referral bias in primary total knee arthroplasty: retrospective analysis of 22,614 surgeries in a tertiary referral center. J Arthroplasty. doi:10.1016/j.arth.2016.08.014.
24. Robinson JC, MacPherson K. Payers test reference pricing and centers of excellence to steer patients to low-price and high-quality providers. Health Affairs. 2012;31(9):2028-2036. doi: 10.1377/hlthaff.2011.1313
25. Laucis NC, Chowdhury M, Dasgupta A, Bhattacharyya T. Trend toward high-volume hospitals and the influence on complications in knee and hip arthroplasty. J Bone Joint Surg Am. 2016;98(9):707-712. doi:10.2106/JBJS.15.00399.
26. Anwar S, Fraser S, Hill J. Surgical specialization and training–its relation to clinical outcome for colorectal cancer surgery. J Eval Clin Pract. 2012;18(1):5-11. doi:10.1111/j.1365-2753.2010.01525.x.
27. Snow BW, Catwright PC, Young MD. Does surgical subspecialization in pediatrics provide high-quality, cost-effective patient care? Pediatrics. 1996;97(1):14-17.
28. Smith J a. E, King PM, Lane RHS, Thompson MR. Evidence of the effect of ‘specialization’ on the management, surgical outcome and survival from colorectal cancer in Wessex. Br J Surg. 2003;90(5):583-592. doi:10.1002/bjs.4085.
29. Hall BL, Hsaio EY, Majercik S, Hirbe M, Hamilton BH. The impact of surgeon specialization on patient mortality: Examination of a continuous Herfindahl-Hirschman Index. Ann Surg. 2009;249(5):708-716. doi: 10.1097/SLA.0b013e3181a335f8.
30. Leopold SS, Morgan HD, Kadel NJ, Gardner GC, Schaad DC, Wolf FM. Impact of educational intervention on confidence and competence in the performance of a simple surgical task. J Bone Joint Surg Am. 2005;87(5):1031-1037. doi:10.2106/JBJS.D.02434.
31. Morrell NT, Mercer DM, Moneim MS. Trends in the orthopedic job market and the importance of fellowship subspecialty training. Orthopedics. 2012;35(4):e555-e560. doi:10.3928/01477447-20120327-13.
TAKE-HOME POINTS
- Between 2012 and 2014, 1,374 surgeons performed >10 total shoulder arthroplasties (TSA) in Medicare patients in at least one year.
- From 2012 to 2014, the number of surgeons performing at least 10 TSA in Medicare patients increased from 834 to 1,078, while the number of TSA increased from 21,137 (25.3 per surgeon) to 26,765 (24.9 per surgeon).
- Many of these surgeons had a diverse surgical practice, with nearly one-half performing total knee arthroplasty, one-third performing non-arthroplasty knee surgeries, and >80% performing non-arthroplasty shoulder procedures.
- Only one-third of these surgeons had formal fellowship training specific to shoulder and elbow.
- In order for the current supply of shoulder and elbow fellowship-trained surgeons to meet the Medicare TSA demand, each currently practicing fellowship graduate would have to perform 140.6 TSA in Medicare patients annually.
Massive Rotator Cuff Tears in Patients Older Than Sixty-five: Indications for Cuff Repair versus Reverse Total Shoulder Arthroplasty
ABSTRACT
The decision to perform rotator cuff repair (RCR) versus reverse total shoulder arthroplasty (rTSA) for massive rotator cuff tear (MCT) without arthritis can be difficult. Our aim was to identify preoperative variables that are influential in a surgeon's decision to choose one of the two procedures and evaluate outcomes.
We retrospectively reviewed 181 patients older than 65 who underwent RCR or rTSA for MCT without arthritis. Clinical and radiographic data were collected and used to evaluate the preoperative variables in each of these two patient populations and assess outcomes.
Ninety-five shoulders underwent RCR and 92 underwent rTSA with an average followup of 44 and 47 months, respectively. Patients selected for RCR had greater preoperative flexion (113 vs 57), abduction (97 vs 53), and external rotation (42 vs 32), higher SST (3.1 vs 1.9) and ASES scores (43.8 vs 38.6), and were less likely to have had previous cuff surgery (6.3% vs 35.9%). Patients selected for rTSA had a smaller acromiohumeral interval (4.8 vs 8.7) and more superior subluxation (50.6% vs 14.1%). Similar preoperative characteristics included pain, comorbidities, and BMI. Patients were satisfied in both groups and had significant improvement in motion and function postoperatively.
Both RCR and rTSA can result in significant functional improvement and patient satisfaction in the setting of MCT without arthritis in patients older than 65. At our institution, patients who underwent rTSA had less pre-operative motion, lower function, more evidence of superior migration, and were more likely to have had previous rotator cuff surgery.
Continue to: The treatment of patients...
The treatment of patients with massive rotator cuff tears (MCTs) without osteoarthritis is challenging. This population is of considerable interest, as the prevalence of MCT has been reported to be as high as 40% of all rotator cuff tears.1Options for surgical treatment in patients who have failed conservative management are numerous and include tendon debridement, partial or complete arthroscopic or open rotator cuff repair (RCR), tendon transfers, reverse total shoulder arthroplasty (rTSA), arthroscopic superior capsular reconstruction (ASCR), and other grafting procedures.2 Arthroscopic superior capsular reconstruction shows promise as a novel technique, but it is not yet well studied. Other procedures such as tendon transfers fit into the treatment algorithm for only a small subset of patients. Open rotator cuff repair and rTSA are the 2 most commonly utilized procedures for MCT, and both have been shown to reliably achieve significant functional improvement and patient satisfaction.3–6
The dilemma for the treating surgeon is deciding which patients to treat with RCR and who to treat with rTSA. Predicting which surgical procedure will provide a better functional result is difficult and controversial.7 The RCR method is a bone-conserving procedure with relatively low surgical risk and allows the option for rTSA to be performed as a salvage surgery should repair fail. It also may be less costly in the appropriate population.8 However, large rotator cuff tears in elderly patients have low healing potential, and the prospect of participating in a lengthy rehabilitation after an operation that may not prove successful can be deterring.9,10 In the elderly population, rTSA may be a reliable option, as tendon healing of the cuff is not necessary to restore function. However, rTSA does not conserve bone, provides a non-anatomic solution, and has had historically high complication rates.4,5
In an effort to aid in the decision-making process when considering these 2 surgical options, we compared RCR and rTSA performed at a single institution for MCT in patients >65 years. Our aim was to identify preoperative patient variables that influence a surgeon’s decision to proceed with 1 of the 2 procedures. Moreover, we evaluated clinical outcomes in these 2 patient populations. We hypothesized that (1) patients selected for rTSA would have worse preoperative function, less range of motion, more comorbidities, more evidence of radiographic subluxation, and a higher likelihood of having undergone previous RCR than those selected for RCR, and (2) both RCR and rTSA would be successful and result in improved clinical outcomes with high patient satisfaction.
MATERIALS AND METHODS
PATIENT SELECTION
We performed a retrospective chart review using our practice database of all patients undergoing arthroscopic RCR and rTSA for any indication by the senior author (M.A.F.) between January 2004 and April 2015. A total of 1503 RCRs and 1973 rTSAs were conducted during the study period. Patient medical records were reviewed, and those meeting the following criteria were included in the study: >65 years at the time of surgery, MCT, no preoperative glenohumeral arthritis, minimum follow-up of 12 months, functional deltoid muscle on physical examination, and no prior shoulder surgery except for RCR or diagnostic arthroscopy. A total of 92 patients who underwent arthroscopic RCR and 89 patients who underwent rTSA met the inclusion criteria. For patients with bilateral shoulder surgery, we measured each shoulder independently. Three patients underwent bilateral rTSA, and 3 patients underwent bilateral RCR, leaving 95 shoulders in the RCR group and 92 in the rTSA group. The Western Institutional Review Board determined this study to be exempt from review.
RADIOGRAPHIC EVALUATION
All patient charts included a radiology report and documented interpretation of the images by the treating surgeon prior to surgery. Radiographs were assessed to assure the absence of preoperative glenohumeral osteoarthritis. The images were also graded based on the Hamada classification.11 Stage 1 is associated with minimal radiographic change with an acromiohumeral interval (AHI) >6 mm; stage 2 is characterized by narrowing of the AHI <6 mm; and Stage 3 is defined by narrowing of the AHI with radiographic changes of the acromion. Stages 4 and higher include arthritic changes to the glenohumeral joint, and they were not included in the study population. The AHI measurements and the presence or absence of glenohumeral subluxation were documented.
Continue to: MASSIVE CUFF TEAR DETERMINATION...
MASSIVE CUFF TEAR DETERMINATION
We defined MCT on the basis of previously described criteria of tears involving ≥2 tendons or tears measuring ≥5 cm in greatest dimension.12,13 Patient charts were screened, and those whose clinical notes or radiology reports indicated an absence of MCT were excluded. Preoperative imaging of the remaining patients was then evaluated by 3 fellowship-trained shoulder surgeons to confirm MCT in all patients with a clinically documented MCT, as well as to assess those who had insufficient documentation of tear size in the notes.
Advanced imaging was evaluated for fatty atrophy of the rotator cuff musculature, and Goutallier classification was assigned.14,15 Length of retraction was measured from the tendon end to the medial aspect of the footprint on coronal imaging, and the subscapularis and teres minor were assessed and documented as torn or intact.16,17
DATA COLLECTION
We reviewed clinical charts and patient questionnaire forms from both the preoperative and follow-up visits. Clinical data collected included gender, age at surgery, active range of motion (forward elevation, abduction, external rotation, and internal rotation), comorbidities, smoking status, BMI, history of shoulder surgery, and any postoperative complications or need for secondary surgery. All patients completed patient-centered questionnaires regarding shoulder pain and dysfunction at each visit or via telephone communication with clinic staff. Outcome measurements used for analysis included American Shoulder and Elbow Surgeons (ASES) Score, simple shoulder test (SST), visual analog score (VAS) pain scale, and patient-reported satisfaction (Graded 1-10; 1 = poor outcome; 4 = satisfactory outcome; 7 = good outcome; 10 = excellent outcome).
DATA ANALYSIS AND STATISTICAL METHODS
Statistical tests were selected based on the result of Shapiro–Wilk test for normality. Continuous variables were evaluated with either independent t test or Mann–Whitney U test. Dependent t test was used to evaluate outcome variables. For categorical variables, either Pearson’s χ2or Fisher’s exact test was performed depending on the sample size. Alpha was set at P =.05.
Continue to: RESULTS...
RESULTS
PREOPERATIVE CHARACTERISTICS
Of the 187 shoulders in the study group, 95 had RCR and 92 had rTSA. Demographic information and preoperative variables for both groups are summarized in Table 1 and Table 2. Patients in the RCR group had greater preoperative forward elevation, abduction, and external rotation and higher preoperative functional scores than those in the rTSA group. Patients in the rTSA group were older and more likely to be female than those in the RCR group. More patients in the rTSA group had undergone prior RCR compared with those in the RCR group. Each of these differences was statistically significant. Subjective pain scores, BMI, and comorbidities were similar between the 2 groups.
Table 1. Patient demographics
| RCR | rTSA | P value |
Age (yr; mean ± SD) | 71 ± 5 | 74 ± 6 | <.0001 |
Gender *male (no.; %) *female (no.; %) | 57 (60%) 38 (40%) | 30 (33%) 62 (67%) | <.0001 |
BMI (mean ± SD) | 28.5 ± 4.4 | 28.1 ± 4.5 | .578 |
Abbreviations: BMI, body mass index; RCR, rotator cuff repair; rTSA, reverse total shoulder arthroplasty.
Table 2. Preoperative variables
| RCR (n=95) | rTSA (n=92) | P value |
Radiographic parameters | |||
AB interval | 9 ± 3 | 5 ± 3 | <.0001 |
Humeral escape | 14.1% | 50.6% | <.0001 |
Hamada 1 | 76.1% | 15.6% | <.0001 |
Hamada 2 | 13.0% | 50.6% | |
Hamada 3 | 10.9% | 33.8% | |
Goutallier grade 1 | 7.8% | 19.3% | .227 |
Goutallier grade 2 | 66.7% | 52.6% | |
Goutallier grade 3 | 21.6% | 19.3% | |
Goutallier grade 4 | 3.9% | 8.8% | |
Clinical measures | |||
Preop FE | 113 ± 50 | 57 ± 34 | <.0001 |
Preop AB | 97 ± 45 | 53 ± 35 | <.0001 |
Preop ER | 42 ± 25 | 32 ± 28 | .029 |
Preop IR | 2.9 ± 1.6 | 2.6 ± 1.8 | .247 |
Preop pain | 5.7 ± 2.3 | 5.6 ± 2.5 | .927 |
Preop ASES | 44 ± 17 | 39 ± 16 | .04 |
Preop SST | 3.1 ± 2.6 | 1.9 ± 1.7 | .001 |
Patients parameters | |||
Previous cuff surgery | 6.3% | 35.9% | <.0001 |
Comorbidity count | 1.7 ± 1.4 | 2.1 ± 2.7 | .126 |
Abbreviations: AB, abduction; ASES, American Shoulder and Elbow Society score; ER, external rotation; FE, forward elevation; IR, internal rotation; preop, preoperative; SST, simple shoulder test.
Radiographically, patients selected to undergo rTSA had a smaller AHI (4.8 vs 8.7, P < .0001) and more evidence of superior subluxation (50.6% vs 14.1%, P < .0001) than those in the RCR group. Average Hamada grade was 1.4 ± 0.7 and 2.2 ± 0.7 for the RCR and rTSA groups, respectively (P < .0001). Average Goutallier grade was similar between the groups (2.2 ± 0.6 for RCR vs 2.2 ± 0.8 for rTSA, P =.227), and 25.5% of the RCR group had Grade 3 or 4 atrophy compared with 28.1% of the rTSA group.
POSTOPERATIVE OUTCOMES
The average follow-up time was 44 months for RCR and 47 months for rTSA. Patients in the RCR and rTSA groups were highly satisfied with the surgery (8.5 ± 2.6 vs 8.2 ± 2.6, P = .461) and had significantly increased range of motion in all planes and improved functional scores (Table 3). The rTSA group had greater net improvement in forward elevation, abduction, and external rotation than the RCR group. Both groups demonstrated similar improvement in ASES, SST, and VAS pain scores.
Table 3. Postoperative outcomes
| RCR (n=95) | P value | rTSA (n=92) | P value | ||
Preoperative | Postoperative | Preoperative | Postoperative | |||
FE | 113 ± 50 | 166 ± 26 | <.0001 | 57 ± 34 | 136 ± 46 | <.0001 |
AB | 97 ± 45 | 155 ± 37 | <.0001 | 53 ± 35 | 129 ± 44 | <.0001 |
ER | 42 ± 25 | 48 ± 20 | .033 | 32 ± 28 | 57 ± 32 | <.0001 |
IR | 2.9 ± 1.6 | 4.6 ± 1.6 | <.0001 | 2.6 ± 1.8 | 4.7 ± 2.4 | <.0001 |
VAS pain | 5.7 ± 2.3 | 1.7 ± 2.4 | <.0001 | 5.6 ± 2.5 | 1.6 ± 2.5 | <.0001 |
ASES | 44 ± 17 | 83 ± 18 | <.0001 | 39 ± 16 | 77 ± 22 | <.0001 |
SST | 3.1 ± 2.6 | 9.3 ± 2.9 | <.0001 | 1.9 ± 1.7 | 7.1 ± 3.4 | <.0001 |
Abbreviations: AB, abduction; ASES, American Shoulder and Elbow Society score; ER, external rotation; FE, forward elevation; IR, internal rotation; SST, simple shoulder test; VAS – visual analog score.
In the RCR group, 5 patients (5.3%) required reoperation: 3 patients underwent conversion to rTSA, 1 patient underwent biceps tenotomy with subacromial decompression, and 1 patient underwent arthroscopic irrigation and debridement for a postoperative Propionibacterium acnes infection. In the rTSA group, 2 patients (2.2%) required reoperation: 1 patient underwent open reduction internal fixation for a scapula fracture that failed conservative management, and 1 patient had an open irrigation and debridement with polyethylene exchange for an acute postoperative infection of unknown source.
DISCUSSION
Massive, retracted rotator cuff tears are a common and difficult problem.1 The treatment options are numerous and depend on a variety of preoperative factors including patient-specific characteristics and factors specific to the tear. For certain patients, nonoperative management may be a reasonable first step, as an MCT does not necessarily preclude painless, functional shoulder motion. Elderly, lower demand individuals have been shown to do well with physical rehabilitation.18 Similarly, for the same category of elderly patients who do not respond to conservative measures, arthroscopic tendon debridement with or without subacromial decompression and/or biceps tenotomy may be effective.1,19 This technique has been described as “limited goals surgery;” despite some mixed results in the literature, multiple studies have reported symptomatic and functional improvement after simple debridement.2,19–21The consensus among several authors has been that this procedure continues to play a role for elderly, low-demand patients whose functional goals are limited and whose primary complaint is pain.1,2,20
For the majority of patients with MCT who desire pain relief and a restoration of shoulder function, RCR remains the gold standard of treatment and should be the primary aim if feasible. Complete RCR has consistently outperformed both partial repair and debridement in multiple studies in terms of pain relief and functional improvement.10,21,22However, elderly patients with chronic, massive tears, particularly in the setting of muscle atrophy, are at high risk of failure with attempted cuff repair.9,23 Novel techniques such as superior capsular reconstruction and subacromial balloon spacer implantation may offer a minimally invasive method of re-centering the humeral head and stabilizing the glenohumeral joint; however, these new treatment options lack any long-term data in the literature to support their widespread use.24–26 Alternatively, rTSA has been shown to be a reliable option to restore shoulder function in the setting of a massive irreparable rotator cuff tear, even in the absence of arthritis.5,27-31
Continue to: The decision-making process...
The decision-making process for selecting RCR or rTSA in the setting of MCT without arthritis in the older population (age >65 years) remains challenging. We attempted to quantify the data of a high-volume surgeon and identify the differences and similarities between those patients selected for either procedure. At our institution, we generally performed rTSA on patients with low preoperative range of motion, poor function based on SST and ASES scores, small AHI, and strong evidence of superior subluxation. We were also more likely to perform rTSA if the patient had a history of rotator cuff surgery. There was a predilection for older age and female gender in those who underwent rTSA.
For our study, we elected to focus on patients >65 years. In our experience, the choice of which surgical procedure to perform is generally easier in younger patients. Most surgeons appropriately opt for an arthroscopic procedure or tendon transfer to preserve bone and maintain the option of rTSA as a salvage procedure if necessary in the future. Studies have reported that <60 years is a predictor of poor outcome with rTSA, and patients <65 years who undergo rTSA have been shown to have high complication rates.30-32 Furthermore, the longevity of the implant in young patients is a significant concern, and revision surgery after rTSA is technically demanding and known to result in poor functional outcomes.32,33
Although the indications for rTSA are expanding, attempts at RCR in the setting of MCT remain largely appropriate. Preserved preoperative anterior elevation >90° has been associated with loss of motion after rTSA and poor satisfaction, and one should exercise caution when considering rTSA in this setting.3 The current study confirmed that even older patients with MCT may be very satisfied with arthroscopic RCR (Figure 1). Both range of motion and function significantly improved, and patients were largely satisfied with the procedure with an average self-reported outcome of good to excellent. At the time of final follow-up for this study, only 3 shoulders in the RCR group had undergone conversion to rTSA. This number may be expected to rise with long follow-up periods, and we feel that prolonging the time before arthroplasty is generally in the best interest of the patient.
Our results were consistent with several reported studies in which RCR has been shown to be successful in the setting of MCT.34–37 Henry and colleagues36 performed a systematic review that evaluated 954 patients who underwent partial or complete anatomic RCR for MCT. Although the average age was 63 years (range, 37–87), functional outcome scores, VAS pain score, and overall range of motion consistently and significantly improved.
rTSA may be a “more reliable” option than RCR in treating MCT in the older population because it does not rely on tendon healing. However, the relationship between tendon healing and clinical outcomes after RCR is unclear. The aforementioned systematic review reported re-tear rates to be as high as 79%, but several studies have reported high satisfaction even in the setting of retear.36 Yoo and colleagues38 and Chung and colleagues9 reported re-tear rates of 45.5% and 39.8%, respectively, but both studies noted that there was no difference in outcome measures between those patients with and without re-tears. In particular, for patients who have had no prior rotator cuff surgery, an attempt at arthroscopic repair may be a prudent option with relatively low risk.
Although certain patients may clinically improve despite suffering a re-tear (or inability to heal in the first place), others continue to experience pain and dysfunction that negatively affect their quality of life.39–41 These patients are more often appropriate candidates for rTSA. Indeed, several studies have demonstrated a higher re-tear rate in patients with a history of surgery than in those without.23,31,38,42 Shamsudin and colleagues43 found revision arthroscopic RCR, even in a younger age group with tears of all sizes, to be twice as likely to re-tear. Notably, re-tear after revision repair may be more likely to be symptomatic, as these re-tears are routinely associated with pain, stiffness, and loss of function. Even in the hands of experienced surgeons in a younger population, revision repair has only been able to reverse pseudoparalysis in 43% of patients, leading to only 39% return to sport or full activity.44 In examining our data, we were much less likely to perform an RCR in patients who had a history of cuff repair surgery than in those without this history.
Continue to: Overall, those patients selected for rTSA...
Overall, those patients selected for rTSA in our study population performed well postoperatively (Figure 2 and Figure 3). Vast improvements were noted in range of motion, function, and pain scores at final follow up. Moreover, no patients in the study group required revision arthroplasty during the follow-up period. Although the average follow-up period was only 47 months, these results suggested that elderly patients with MCT without arthritis may be particularly ideal candidates for rTSA with regard to implant survival and anticipated revision rate when chosen appropriately.
Several weaknesses were noted within this paper. First, the study was retrospective, precluding randomization of treatment groups and standardization of data collection and follow-up. The outcomes of RCR and rTSA could not be compared directly due to the inherent selection bias. The groups clearly differed in many respects, and these preoperative factors likely played a role in postoperative outcomes. However, the primary goal of this study was not to compare outcomes of the treatment groups but to analyze the patterns of patient selection by an experienced treating surgeon and contribute to published data that each surgery can be successful in this patient population when chosen appropriately.
Second, our data were based on a single surgeon’s decisions, and results may not be generalizable. Furthermore, the senior author has had a longstanding interest in reverse shoulder arthroplasty and has published data illustrating successful outcomes for rTSA in patients with MCT. For this reason, one could presume that there may have been some bias toward treating patients with rTSA. However, we feel that the senior author’s unique and longstanding experience in treating MCT allows for a thorough evaluation and comparison of preoperative variables and outcomes declared within this study. Indeed, many patients included in this study were referred from outside institutions specifically for rTSA but instead were deemed more appropriate candidates for RCR and underwent successful arthroscopic repair, a common scenario which served as an impetus for this study.
CONCLUSION
RCR and rTSA are both viable options for patients >65 years with MCT without arthritis. Treatment must be individualized for each patient with careful consideration of a number of preoperative variables and patient characteristics. At our institution, patients with previous RCR, decreased range of motion, poor function, and strong radiographic evidence of subluxation are more likely to undergo rTSA. When chosen appropriately, both RCR and rTSA can result in improved range of motion, function, and high patient satisfaction in this patient population.
- Bedi A, Dines J, Warren RF, Dines DM. Massive tears of the rotator cuff. J Bone Joint Surg Am. 2010;92:1894-1908. doi:10.2106/JBJS.I.01531.
- Greenspoon JA, Petri M, Warth RJ, Millett PJ. Massive rotator cuff tears: pathomechanics, current treatment options, and clinical outcomes. J Shoulder Elbow Surg. 2015;24:1493-1505. doi:10.1016/j.jse.2015.04.005.
- Boileau P, Gonzalez JF, Chuinard C, Bicknell R, Walch G. Reverse total shoulder arthroplasty after failed rotator cuff surgery. J Shoulder Elbow Surg. 2009;18:600-606. doi:10.1016/j.jse.2009.03.011.
- Cuff D, Pupello D, Virani N, Levy J, Frankle M. Reverse shoulder arthroplasty for the treatment of rotator cuff deficiency. J Bone Joint Surg Am. 2008;90:1244-1251. doi:10.2106/JBJS.G.00775.
- Mulieri P, Dunning P, Klein S, Pupello D, Frankle M. Reverse shoulder arthroplasty for the treatment of irreparable rotator cuff tear without glenohumeral arthritis. J Bone Joint Surg Am. 2010;92:2544-2556.doi:10.2106/JBJS.I.00912.
- Wall B, Nove-Josserand L, O'Connor DP, Edwards TB, Walch G. Reverse total shoulder arthroplasty: a review of results according to etiology. J Bone Joint Surg Am. 2007;89:1476-1485. doi:10.2106/JBJS.F.00666.
- Pill SG, Walch G, Hawkins RJ, Kissenberth MJ. The role of the biceps tendon in massive rotator cuff tears. Instr Course Lect. 2012;61:113-120.
- Makhni EC, Swart E, Steinhaus ME, Mather RC 3rd, Levine WN, Bach BR Jr et al. Cost-effectiveness of reverse total shoulder arthroplasty versus arthroscopic rotator cuff repair for symptomatic large and massive rotator cuff tears. Arthroscopy. 2016;32(9):1771-1780. doi:10.1016/j.arthro.2016.01.063.
- Chung SW, Kim JY, Kim MH, Kim SH, Oh JH. Arthroscopic repair of massive rotator cuff tears: outcome and analysis of factors associated with healing failure or poor postoperative function. Am J Sports Med. 2013;41:1674-1683. doi:10.1177/0363546513485719.
- Holtby R, Razmjou H. Relationship between clinical and surgical findings and reparability of large and massive rotator cuff tears: a longitudinal study. BMC Musculoskelet Disord. 2014;15:180. doi:10.1186/1471-2474-15-180.
- Hamada K, Fukuda H, Mikasa M, Kobayashi Y. Roentgenographic findings in massive rotator cuff tears. A long-term observation. Clin Orthop Relat Res. 1990;254:92-96.
- DeOrio JK, Cofield RH. Results of a second attempt at surgical repair of a failed initial rotator-cuff repair. J Bone Joint Surg Am. 1984;66:563-567.
- Gerber C, Fuchs B, Hodler J. The results of repair of massive tears of the rotator cuff. J Bone Joint Surg Am. 2000;82:505-515.
- Fuchs B, Weishaupt D, Zanetti M, Hodler J, Gerber C. Fatty degeneration of the muscles of the rotator cuff: assessment by computed tomography versus magnetic resonance imaging. J Shoulder Elbow Surg. 1999;8:599-605.
- Goutallier D, Bernageau J, Patte D. Assessment of the trophicity of the muscles of the ruptured rotator cuff by CT scan. In: Post M, Morrey B, Hawkins R, eds. Surgery of the Shoulder. St. Louis, MO: Mosby, 1990;11-13.
- Meyer DC, Farshad M, Amacker NA, Gerber C, Wieser K. Quantitative analysis of muscle and tendon retraction in chronic rotator cuff tears. Am J Sports Med. 2012;40(3):606-610.
- Meyer DC, Wieser K, Farshad M, Gerber C. Retraction of supraspinatus muscle and tendon as predictors of success of rotator cuff repair. Am J Sports Med. 2012;40:2242-2247.
- Williams GR Jr, Rockwood CA Jr, Bigliani LU, Ianotti JP, Stanwood W. Rotator cuff tears: why do we repair them? J Bone Joint Surg Am. 2004;86-A(12):2764-2776.
- Rockwood CA Jr, Williams GR Jr, Burkhead WZ Jr. Debridement of degenerative, irreparable lesions of the rotator cuff. J Bone Joint Surg Am. 1995;77:857-866.
- Berth A, Neumann W, Awiszus F, Pap G. Massive rotator cuff tears: functional outcome after debridement or arthroscopic partial repair. J Orthopaed Traumatol. 2010;11:13-20. doi 10.1007/s10195-010-0084-0.
- Heuberer PR, Kolblinger R, Buchleitner S, Pauzenberger L, Laky B, Auffarth A, et al. Arthroscopic management of massive rotator cuff tears: an evaluation of debridement, complete, and partial repair with and without force couple restoration. Knee Surg Sports Traumatol Arthrosc. 2016;24:3828-3837.
- Moser M, Jablonski MV, Horodyski M, Wright TW. Functional outcome of surgically treated massive rotator cuff tears: a comparison of complete repair, partial repair, and debridement. Orthopedics.2007;30(6):479-482.
- Rhee YG, Cho NS, Yoo JH. Clinical outcome and repair integrity after rotator cuff repair in patients older than 70 years versus patients younger than 70 years. Arthroscopy. 2014;30:546-554. doi:10.1016/j.arthro.2014.02.006.
- Denard PJ, Brady PC, Adams CR, Tokish JM, Burkhart SS. Preliminary results of arthroscopic superior capsule reconstruction with dermal allograft. Arthroscopy. 2018;34(1):93-99. doi: 10.1016/j.arthro.2017.08.265.
- Mihata T, Lee TQ, Watanabe C, Fukunishi K, Ohue M, Tsujimura T, Kinoshita M. Clinical results of arthroscopic superior capsule reconstruction for irreparable rotator cuff tears. Arthroscopy.2013;29:459-70.
- Piekaar RSM, Bouman ICE, van Kampen PM, van Eijk F, Huijsmans PE. Early promising outcome following arthroscopic implantation of the subacromial balloon spacer for treating massive rotator cuff tear. Musculoskelet Surg. 2018;102(3):247-255. doi: 10.1007/s12306-017-0525-5.
- Al-Hadithy N, Domos P, Sewell MD, Pandit R. Reverse shoulder arthroplasty in 41 patients with cuff tear arthropathy with a mean follow-up period of 5 years. J Shoulder Elbow Surg. 2014;23:1662-1668. doi:10.1016/j.jse.2014.03.001.
- Boileau P, Watkinson DJ, Hatzidakis AM, Balg F. Grammont reverse prosthesis: design, rationale, and biomechanics. J Shoulder Elbow Surg. 2005;14:147S-161S. doi:10.1016/j.jse.2004.10.006.
- Grammont PM, Baulot E. Delta shoulder prosthesis for rotator cuff rupture. Orthopedics 1993;16:65-68. doi: 10.3928/0147-7447-19930101-11.
- Hartzler RU, Steen BM, Hussey MM, Cusick MC, Cottrell BJ, Clark RE, Frankle MA. Reverse shoulder arthroplasty for massive rotator cuff tear: risk factors for poor functional improvement. J Shoulder Elbow Surg. 2015;24:1698-1706. doi:10.1016/j.jse.2015.04.015.
- Kim HM, Caldwell JM, Buza JA, Fink LA, Ahmad CS, Bigliani LU, Levine WN. Factors affecting satisfaction and shoulder function in patients with a recurrent rotator cuff tear. J Bone Joint Surg Am. 2014;96:106-112. doi:10.2106/JBJS.L.01649.
- Ek ET, Neukom L, Catanzaro S, Gerber C. Reverse total shoulder arthroplasty for massive irreparable rotator cuff tears in patients younger than 65 years old: results after five to fifteen years. J Shoulder Elbow Surg. 2013;22:1199-1208. doi:10.1016/j.jse.2012.11.016.
- Sershon RA, Van Thiel GS, Lin EC, McGill KC, Cole BJ, Verma NN, et al. Clinical outcomes of reverse total shoulder arthroplasty in patients aged younger than 60 years. J Shoulder Elbow Surg.2014;23:395-400. doi:10.1016/j.jse.2013.07.047.
- Denard PJ, Ladermann A, Jiwani AZ, Burkhart SS. Functional outcome after arthroscopic repair of massive rotator cuff tears in individuals with pseudoparalysis. Arthroscopy. 2012;28:1214-1219. doi:10.1016/j.arthro.2012.02.026.
- Denard PJ, Ladermann A, Brady PC, Narbona P, Adams CR, Arrigoni P, et al. Pseudoparalysis from a massive rotator cuff tear is reliably reversed with an arthroscopic rotator cuff repair in patients without preoperative glenohumeral arthritis. Am J Sports Med. 2015;43:2373-2378. doi: 10.1177/0363546515597486.
- Henry P, Wasserstein D, Park S, Dwyer T, Chahal J, Slobogean G, Schemitsch E. Arthroscopic repair for chronic massive rotator cuff tears: a systematic review. Arthroscopy. 2015;31:2472-2480. doi:10.1016/j.arthro.2015.06.038.
- Oh JH, Kim SH, Shin SH, Chung SW, Kim JY, Kim SJ. Outcome of rotator cuff repair in large-to-massive tear with pseudoparalysis: a comparative study with propensity score matching. Am J Sports Med.2011;39:1413-1420.
- Yoo JC, Ahn JH, Koh KH, Lim KS. Rotator cuff integrity after arthroscopic repair for large tears with less-than-optimal footprint coverage. Arthroscopy. 2009;25:1093-1100. doi:10.1016/j.arthro.2009.07.010.
- Jost B, Pfirrmann CW, Gerber C, Switzerland Z. Clinical outcome after structural failure of rotator cuff repairs. J Bone Joint Surg Am. 2000;82:304-314.
- Klepps S, Bishop J, Lin J, Cahlon O, Strauss A, Hayes P, Flatow EL Prospective evaluation of the effect of rotator cuff integrity on the outcome of open rotator cuff repairs. Am J Sports Med. 2004;32:1716-1722.
- Liu SH, Baker CL. Arthroscopically assisted rotator cuff repair: correlation of functional results with integrity of the cuff. Arthroscopy. 1994;10:54-60.
- Papadopoulos P, Karataglis D, Boutsiadis A, Fotiadou A, Christoforidis J, Christodoulou A. Functional outcome and structural integrity following mini-open repair of large and massive rotator cuff tears: a 3-5 year follow-up study. J Shoulder Elbow Surg. 2011;20:131-137. doi:10.1016/j.jse.2010.05.026.
- Shamsudin A, Lam PH, Peters K, Rubenis I, Hackett L, Murrell GA. Revision versus primary arthroscopic rotator cuff repair: a 2-year analysis of outcomes in 360 patients. Am J Sports Med.2015;43:557-564. doi:10.1177/0363546514560729.
- Ladermann A, Denard PJ, Burkhart SS. Midterm outcome of arthroscopic revision repair of massive and nonmassive rotator cuff tears. Arthroscopy. 2011;27:1620-1627. doi:10.1016/j.arthro.2011.08.290.
ABSTRACT
The decision to perform rotator cuff repair (RCR) versus reverse total shoulder arthroplasty (rTSA) for massive rotator cuff tear (MCT) without arthritis can be difficult. Our aim was to identify preoperative variables that are influential in a surgeon's decision to choose one of the two procedures and evaluate outcomes.
We retrospectively reviewed 181 patients older than 65 who underwent RCR or rTSA for MCT without arthritis. Clinical and radiographic data were collected and used to evaluate the preoperative variables in each of these two patient populations and assess outcomes.
Ninety-five shoulders underwent RCR and 92 underwent rTSA with an average followup of 44 and 47 months, respectively. Patients selected for RCR had greater preoperative flexion (113 vs 57), abduction (97 vs 53), and external rotation (42 vs 32), higher SST (3.1 vs 1.9) and ASES scores (43.8 vs 38.6), and were less likely to have had previous cuff surgery (6.3% vs 35.9%). Patients selected for rTSA had a smaller acromiohumeral interval (4.8 vs 8.7) and more superior subluxation (50.6% vs 14.1%). Similar preoperative characteristics included pain, comorbidities, and BMI. Patients were satisfied in both groups and had significant improvement in motion and function postoperatively.
Both RCR and rTSA can result in significant functional improvement and patient satisfaction in the setting of MCT without arthritis in patients older than 65. At our institution, patients who underwent rTSA had less pre-operative motion, lower function, more evidence of superior migration, and were more likely to have had previous rotator cuff surgery.
Continue to: The treatment of patients...
The treatment of patients with massive rotator cuff tears (MCTs) without osteoarthritis is challenging. This population is of considerable interest, as the prevalence of MCT has been reported to be as high as 40% of all rotator cuff tears.1Options for surgical treatment in patients who have failed conservative management are numerous and include tendon debridement, partial or complete arthroscopic or open rotator cuff repair (RCR), tendon transfers, reverse total shoulder arthroplasty (rTSA), arthroscopic superior capsular reconstruction (ASCR), and other grafting procedures.2 Arthroscopic superior capsular reconstruction shows promise as a novel technique, but it is not yet well studied. Other procedures such as tendon transfers fit into the treatment algorithm for only a small subset of patients. Open rotator cuff repair and rTSA are the 2 most commonly utilized procedures for MCT, and both have been shown to reliably achieve significant functional improvement and patient satisfaction.3–6
The dilemma for the treating surgeon is deciding which patients to treat with RCR and who to treat with rTSA. Predicting which surgical procedure will provide a better functional result is difficult and controversial.7 The RCR method is a bone-conserving procedure with relatively low surgical risk and allows the option for rTSA to be performed as a salvage surgery should repair fail. It also may be less costly in the appropriate population.8 However, large rotator cuff tears in elderly patients have low healing potential, and the prospect of participating in a lengthy rehabilitation after an operation that may not prove successful can be deterring.9,10 In the elderly population, rTSA may be a reliable option, as tendon healing of the cuff is not necessary to restore function. However, rTSA does not conserve bone, provides a non-anatomic solution, and has had historically high complication rates.4,5
In an effort to aid in the decision-making process when considering these 2 surgical options, we compared RCR and rTSA performed at a single institution for MCT in patients >65 years. Our aim was to identify preoperative patient variables that influence a surgeon’s decision to proceed with 1 of the 2 procedures. Moreover, we evaluated clinical outcomes in these 2 patient populations. We hypothesized that (1) patients selected for rTSA would have worse preoperative function, less range of motion, more comorbidities, more evidence of radiographic subluxation, and a higher likelihood of having undergone previous RCR than those selected for RCR, and (2) both RCR and rTSA would be successful and result in improved clinical outcomes with high patient satisfaction.
MATERIALS AND METHODS
PATIENT SELECTION
We performed a retrospective chart review using our practice database of all patients undergoing arthroscopic RCR and rTSA for any indication by the senior author (M.A.F.) between January 2004 and April 2015. A total of 1503 RCRs and 1973 rTSAs were conducted during the study period. Patient medical records were reviewed, and those meeting the following criteria were included in the study: >65 years at the time of surgery, MCT, no preoperative glenohumeral arthritis, minimum follow-up of 12 months, functional deltoid muscle on physical examination, and no prior shoulder surgery except for RCR or diagnostic arthroscopy. A total of 92 patients who underwent arthroscopic RCR and 89 patients who underwent rTSA met the inclusion criteria. For patients with bilateral shoulder surgery, we measured each shoulder independently. Three patients underwent bilateral rTSA, and 3 patients underwent bilateral RCR, leaving 95 shoulders in the RCR group and 92 in the rTSA group. The Western Institutional Review Board determined this study to be exempt from review.
RADIOGRAPHIC EVALUATION
All patient charts included a radiology report and documented interpretation of the images by the treating surgeon prior to surgery. Radiographs were assessed to assure the absence of preoperative glenohumeral osteoarthritis. The images were also graded based on the Hamada classification.11 Stage 1 is associated with minimal radiographic change with an acromiohumeral interval (AHI) >6 mm; stage 2 is characterized by narrowing of the AHI <6 mm; and Stage 3 is defined by narrowing of the AHI with radiographic changes of the acromion. Stages 4 and higher include arthritic changes to the glenohumeral joint, and they were not included in the study population. The AHI measurements and the presence or absence of glenohumeral subluxation were documented.
Continue to: MASSIVE CUFF TEAR DETERMINATION...
MASSIVE CUFF TEAR DETERMINATION
We defined MCT on the basis of previously described criteria of tears involving ≥2 tendons or tears measuring ≥5 cm in greatest dimension.12,13 Patient charts were screened, and those whose clinical notes or radiology reports indicated an absence of MCT were excluded. Preoperative imaging of the remaining patients was then evaluated by 3 fellowship-trained shoulder surgeons to confirm MCT in all patients with a clinically documented MCT, as well as to assess those who had insufficient documentation of tear size in the notes.
Advanced imaging was evaluated for fatty atrophy of the rotator cuff musculature, and Goutallier classification was assigned.14,15 Length of retraction was measured from the tendon end to the medial aspect of the footprint on coronal imaging, and the subscapularis and teres minor were assessed and documented as torn or intact.16,17
DATA COLLECTION
We reviewed clinical charts and patient questionnaire forms from both the preoperative and follow-up visits. Clinical data collected included gender, age at surgery, active range of motion (forward elevation, abduction, external rotation, and internal rotation), comorbidities, smoking status, BMI, history of shoulder surgery, and any postoperative complications or need for secondary surgery. All patients completed patient-centered questionnaires regarding shoulder pain and dysfunction at each visit or via telephone communication with clinic staff. Outcome measurements used for analysis included American Shoulder and Elbow Surgeons (ASES) Score, simple shoulder test (SST), visual analog score (VAS) pain scale, and patient-reported satisfaction (Graded 1-10; 1 = poor outcome; 4 = satisfactory outcome; 7 = good outcome; 10 = excellent outcome).
DATA ANALYSIS AND STATISTICAL METHODS
Statistical tests were selected based on the result of Shapiro–Wilk test for normality. Continuous variables were evaluated with either independent t test or Mann–Whitney U test. Dependent t test was used to evaluate outcome variables. For categorical variables, either Pearson’s χ2or Fisher’s exact test was performed depending on the sample size. Alpha was set at P =.05.
Continue to: RESULTS...
RESULTS
PREOPERATIVE CHARACTERISTICS
Of the 187 shoulders in the study group, 95 had RCR and 92 had rTSA. Demographic information and preoperative variables for both groups are summarized in Table 1 and Table 2. Patients in the RCR group had greater preoperative forward elevation, abduction, and external rotation and higher preoperative functional scores than those in the rTSA group. Patients in the rTSA group were older and more likely to be female than those in the RCR group. More patients in the rTSA group had undergone prior RCR compared with those in the RCR group. Each of these differences was statistically significant. Subjective pain scores, BMI, and comorbidities were similar between the 2 groups.
Table 1. Patient demographics
| RCR | rTSA | P value |
Age (yr; mean ± SD) | 71 ± 5 | 74 ± 6 | <.0001 |
Gender *male (no.; %) *female (no.; %) | 57 (60%) 38 (40%) | 30 (33%) 62 (67%) | <.0001 |
BMI (mean ± SD) | 28.5 ± 4.4 | 28.1 ± 4.5 | .578 |
Abbreviations: BMI, body mass index; RCR, rotator cuff repair; rTSA, reverse total shoulder arthroplasty.
Table 2. Preoperative variables
| RCR (n=95) | rTSA (n=92) | P value |
Radiographic parameters | |||
AB interval | 9 ± 3 | 5 ± 3 | <.0001 |
Humeral escape | 14.1% | 50.6% | <.0001 |
Hamada 1 | 76.1% | 15.6% | <.0001 |
Hamada 2 | 13.0% | 50.6% | |
Hamada 3 | 10.9% | 33.8% | |
Goutallier grade 1 | 7.8% | 19.3% | .227 |
Goutallier grade 2 | 66.7% | 52.6% | |
Goutallier grade 3 | 21.6% | 19.3% | |
Goutallier grade 4 | 3.9% | 8.8% | |
Clinical measures | |||
Preop FE | 113 ± 50 | 57 ± 34 | <.0001 |
Preop AB | 97 ± 45 | 53 ± 35 | <.0001 |
Preop ER | 42 ± 25 | 32 ± 28 | .029 |
Preop IR | 2.9 ± 1.6 | 2.6 ± 1.8 | .247 |
Preop pain | 5.7 ± 2.3 | 5.6 ± 2.5 | .927 |
Preop ASES | 44 ± 17 | 39 ± 16 | .04 |
Preop SST | 3.1 ± 2.6 | 1.9 ± 1.7 | .001 |
Patients parameters | |||
Previous cuff surgery | 6.3% | 35.9% | <.0001 |
Comorbidity count | 1.7 ± 1.4 | 2.1 ± 2.7 | .126 |
Abbreviations: AB, abduction; ASES, American Shoulder and Elbow Society score; ER, external rotation; FE, forward elevation; IR, internal rotation; preop, preoperative; SST, simple shoulder test.
Radiographically, patients selected to undergo rTSA had a smaller AHI (4.8 vs 8.7, P < .0001) and more evidence of superior subluxation (50.6% vs 14.1%, P < .0001) than those in the RCR group. Average Hamada grade was 1.4 ± 0.7 and 2.2 ± 0.7 for the RCR and rTSA groups, respectively (P < .0001). Average Goutallier grade was similar between the groups (2.2 ± 0.6 for RCR vs 2.2 ± 0.8 for rTSA, P =.227), and 25.5% of the RCR group had Grade 3 or 4 atrophy compared with 28.1% of the rTSA group.
POSTOPERATIVE OUTCOMES
The average follow-up time was 44 months for RCR and 47 months for rTSA. Patients in the RCR and rTSA groups were highly satisfied with the surgery (8.5 ± 2.6 vs 8.2 ± 2.6, P = .461) and had significantly increased range of motion in all planes and improved functional scores (Table 3). The rTSA group had greater net improvement in forward elevation, abduction, and external rotation than the RCR group. Both groups demonstrated similar improvement in ASES, SST, and VAS pain scores.
Table 3. Postoperative outcomes
| RCR (n=95) | P value | rTSA (n=92) | P value | ||
Preoperative | Postoperative | Preoperative | Postoperative | |||
FE | 113 ± 50 | 166 ± 26 | <.0001 | 57 ± 34 | 136 ± 46 | <.0001 |
AB | 97 ± 45 | 155 ± 37 | <.0001 | 53 ± 35 | 129 ± 44 | <.0001 |
ER | 42 ± 25 | 48 ± 20 | .033 | 32 ± 28 | 57 ± 32 | <.0001 |
IR | 2.9 ± 1.6 | 4.6 ± 1.6 | <.0001 | 2.6 ± 1.8 | 4.7 ± 2.4 | <.0001 |
VAS pain | 5.7 ± 2.3 | 1.7 ± 2.4 | <.0001 | 5.6 ± 2.5 | 1.6 ± 2.5 | <.0001 |
ASES | 44 ± 17 | 83 ± 18 | <.0001 | 39 ± 16 | 77 ± 22 | <.0001 |
SST | 3.1 ± 2.6 | 9.3 ± 2.9 | <.0001 | 1.9 ± 1.7 | 7.1 ± 3.4 | <.0001 |
Abbreviations: AB, abduction; ASES, American Shoulder and Elbow Society score; ER, external rotation; FE, forward elevation; IR, internal rotation; SST, simple shoulder test; VAS – visual analog score.
In the RCR group, 5 patients (5.3%) required reoperation: 3 patients underwent conversion to rTSA, 1 patient underwent biceps tenotomy with subacromial decompression, and 1 patient underwent arthroscopic irrigation and debridement for a postoperative Propionibacterium acnes infection. In the rTSA group, 2 patients (2.2%) required reoperation: 1 patient underwent open reduction internal fixation for a scapula fracture that failed conservative management, and 1 patient had an open irrigation and debridement with polyethylene exchange for an acute postoperative infection of unknown source.
DISCUSSION
Massive, retracted rotator cuff tears are a common and difficult problem.1 The treatment options are numerous and depend on a variety of preoperative factors including patient-specific characteristics and factors specific to the tear. For certain patients, nonoperative management may be a reasonable first step, as an MCT does not necessarily preclude painless, functional shoulder motion. Elderly, lower demand individuals have been shown to do well with physical rehabilitation.18 Similarly, for the same category of elderly patients who do not respond to conservative measures, arthroscopic tendon debridement with or without subacromial decompression and/or biceps tenotomy may be effective.1,19 This technique has been described as “limited goals surgery;” despite some mixed results in the literature, multiple studies have reported symptomatic and functional improvement after simple debridement.2,19–21The consensus among several authors has been that this procedure continues to play a role for elderly, low-demand patients whose functional goals are limited and whose primary complaint is pain.1,2,20
For the majority of patients with MCT who desire pain relief and a restoration of shoulder function, RCR remains the gold standard of treatment and should be the primary aim if feasible. Complete RCR has consistently outperformed both partial repair and debridement in multiple studies in terms of pain relief and functional improvement.10,21,22However, elderly patients with chronic, massive tears, particularly in the setting of muscle atrophy, are at high risk of failure with attempted cuff repair.9,23 Novel techniques such as superior capsular reconstruction and subacromial balloon spacer implantation may offer a minimally invasive method of re-centering the humeral head and stabilizing the glenohumeral joint; however, these new treatment options lack any long-term data in the literature to support their widespread use.24–26 Alternatively, rTSA has been shown to be a reliable option to restore shoulder function in the setting of a massive irreparable rotator cuff tear, even in the absence of arthritis.5,27-31
Continue to: The decision-making process...
The decision-making process for selecting RCR or rTSA in the setting of MCT without arthritis in the older population (age >65 years) remains challenging. We attempted to quantify the data of a high-volume surgeon and identify the differences and similarities between those patients selected for either procedure. At our institution, we generally performed rTSA on patients with low preoperative range of motion, poor function based on SST and ASES scores, small AHI, and strong evidence of superior subluxation. We were also more likely to perform rTSA if the patient had a history of rotator cuff surgery. There was a predilection for older age and female gender in those who underwent rTSA.
For our study, we elected to focus on patients >65 years. In our experience, the choice of which surgical procedure to perform is generally easier in younger patients. Most surgeons appropriately opt for an arthroscopic procedure or tendon transfer to preserve bone and maintain the option of rTSA as a salvage procedure if necessary in the future. Studies have reported that <60 years is a predictor of poor outcome with rTSA, and patients <65 years who undergo rTSA have been shown to have high complication rates.30-32 Furthermore, the longevity of the implant in young patients is a significant concern, and revision surgery after rTSA is technically demanding and known to result in poor functional outcomes.32,33
Although the indications for rTSA are expanding, attempts at RCR in the setting of MCT remain largely appropriate. Preserved preoperative anterior elevation >90° has been associated with loss of motion after rTSA and poor satisfaction, and one should exercise caution when considering rTSA in this setting.3 The current study confirmed that even older patients with MCT may be very satisfied with arthroscopic RCR (Figure 1). Both range of motion and function significantly improved, and patients were largely satisfied with the procedure with an average self-reported outcome of good to excellent. At the time of final follow-up for this study, only 3 shoulders in the RCR group had undergone conversion to rTSA. This number may be expected to rise with long follow-up periods, and we feel that prolonging the time before arthroplasty is generally in the best interest of the patient.
Our results were consistent with several reported studies in which RCR has been shown to be successful in the setting of MCT.34–37 Henry and colleagues36 performed a systematic review that evaluated 954 patients who underwent partial or complete anatomic RCR for MCT. Although the average age was 63 years (range, 37–87), functional outcome scores, VAS pain score, and overall range of motion consistently and significantly improved.
rTSA may be a “more reliable” option than RCR in treating MCT in the older population because it does not rely on tendon healing. However, the relationship between tendon healing and clinical outcomes after RCR is unclear. The aforementioned systematic review reported re-tear rates to be as high as 79%, but several studies have reported high satisfaction even in the setting of retear.36 Yoo and colleagues38 and Chung and colleagues9 reported re-tear rates of 45.5% and 39.8%, respectively, but both studies noted that there was no difference in outcome measures between those patients with and without re-tears. In particular, for patients who have had no prior rotator cuff surgery, an attempt at arthroscopic repair may be a prudent option with relatively low risk.
Although certain patients may clinically improve despite suffering a re-tear (or inability to heal in the first place), others continue to experience pain and dysfunction that negatively affect their quality of life.39–41 These patients are more often appropriate candidates for rTSA. Indeed, several studies have demonstrated a higher re-tear rate in patients with a history of surgery than in those without.23,31,38,42 Shamsudin and colleagues43 found revision arthroscopic RCR, even in a younger age group with tears of all sizes, to be twice as likely to re-tear. Notably, re-tear after revision repair may be more likely to be symptomatic, as these re-tears are routinely associated with pain, stiffness, and loss of function. Even in the hands of experienced surgeons in a younger population, revision repair has only been able to reverse pseudoparalysis in 43% of patients, leading to only 39% return to sport or full activity.44 In examining our data, we were much less likely to perform an RCR in patients who had a history of cuff repair surgery than in those without this history.
Continue to: Overall, those patients selected for rTSA...
Overall, those patients selected for rTSA in our study population performed well postoperatively (Figure 2 and Figure 3). Vast improvements were noted in range of motion, function, and pain scores at final follow up. Moreover, no patients in the study group required revision arthroplasty during the follow-up period. Although the average follow-up period was only 47 months, these results suggested that elderly patients with MCT without arthritis may be particularly ideal candidates for rTSA with regard to implant survival and anticipated revision rate when chosen appropriately.
Several weaknesses were noted within this paper. First, the study was retrospective, precluding randomization of treatment groups and standardization of data collection and follow-up. The outcomes of RCR and rTSA could not be compared directly due to the inherent selection bias. The groups clearly differed in many respects, and these preoperative factors likely played a role in postoperative outcomes. However, the primary goal of this study was not to compare outcomes of the treatment groups but to analyze the patterns of patient selection by an experienced treating surgeon and contribute to published data that each surgery can be successful in this patient population when chosen appropriately.
Second, our data were based on a single surgeon’s decisions, and results may not be generalizable. Furthermore, the senior author has had a longstanding interest in reverse shoulder arthroplasty and has published data illustrating successful outcomes for rTSA in patients with MCT. For this reason, one could presume that there may have been some bias toward treating patients with rTSA. However, we feel that the senior author’s unique and longstanding experience in treating MCT allows for a thorough evaluation and comparison of preoperative variables and outcomes declared within this study. Indeed, many patients included in this study were referred from outside institutions specifically for rTSA but instead were deemed more appropriate candidates for RCR and underwent successful arthroscopic repair, a common scenario which served as an impetus for this study.
CONCLUSION
RCR and rTSA are both viable options for patients >65 years with MCT without arthritis. Treatment must be individualized for each patient with careful consideration of a number of preoperative variables and patient characteristics. At our institution, patients with previous RCR, decreased range of motion, poor function, and strong radiographic evidence of subluxation are more likely to undergo rTSA. When chosen appropriately, both RCR and rTSA can result in improved range of motion, function, and high patient satisfaction in this patient population.
ABSTRACT
The decision to perform rotator cuff repair (RCR) versus reverse total shoulder arthroplasty (rTSA) for massive rotator cuff tear (MCT) without arthritis can be difficult. Our aim was to identify preoperative variables that are influential in a surgeon's decision to choose one of the two procedures and evaluate outcomes.
We retrospectively reviewed 181 patients older than 65 who underwent RCR or rTSA for MCT without arthritis. Clinical and radiographic data were collected and used to evaluate the preoperative variables in each of these two patient populations and assess outcomes.
Ninety-five shoulders underwent RCR and 92 underwent rTSA with an average followup of 44 and 47 months, respectively. Patients selected for RCR had greater preoperative flexion (113 vs 57), abduction (97 vs 53), and external rotation (42 vs 32), higher SST (3.1 vs 1.9) and ASES scores (43.8 vs 38.6), and were less likely to have had previous cuff surgery (6.3% vs 35.9%). Patients selected for rTSA had a smaller acromiohumeral interval (4.8 vs 8.7) and more superior subluxation (50.6% vs 14.1%). Similar preoperative characteristics included pain, comorbidities, and BMI. Patients were satisfied in both groups and had significant improvement in motion and function postoperatively.
Both RCR and rTSA can result in significant functional improvement and patient satisfaction in the setting of MCT without arthritis in patients older than 65. At our institution, patients who underwent rTSA had less pre-operative motion, lower function, more evidence of superior migration, and were more likely to have had previous rotator cuff surgery.
Continue to: The treatment of patients...
The treatment of patients with massive rotator cuff tears (MCTs) without osteoarthritis is challenging. This population is of considerable interest, as the prevalence of MCT has been reported to be as high as 40% of all rotator cuff tears.1Options for surgical treatment in patients who have failed conservative management are numerous and include tendon debridement, partial or complete arthroscopic or open rotator cuff repair (RCR), tendon transfers, reverse total shoulder arthroplasty (rTSA), arthroscopic superior capsular reconstruction (ASCR), and other grafting procedures.2 Arthroscopic superior capsular reconstruction shows promise as a novel technique, but it is not yet well studied. Other procedures such as tendon transfers fit into the treatment algorithm for only a small subset of patients. Open rotator cuff repair and rTSA are the 2 most commonly utilized procedures for MCT, and both have been shown to reliably achieve significant functional improvement and patient satisfaction.3–6
The dilemma for the treating surgeon is deciding which patients to treat with RCR and who to treat with rTSA. Predicting which surgical procedure will provide a better functional result is difficult and controversial.7 The RCR method is a bone-conserving procedure with relatively low surgical risk and allows the option for rTSA to be performed as a salvage surgery should repair fail. It also may be less costly in the appropriate population.8 However, large rotator cuff tears in elderly patients have low healing potential, and the prospect of participating in a lengthy rehabilitation after an operation that may not prove successful can be deterring.9,10 In the elderly population, rTSA may be a reliable option, as tendon healing of the cuff is not necessary to restore function. However, rTSA does not conserve bone, provides a non-anatomic solution, and has had historically high complication rates.4,5
In an effort to aid in the decision-making process when considering these 2 surgical options, we compared RCR and rTSA performed at a single institution for MCT in patients >65 years. Our aim was to identify preoperative patient variables that influence a surgeon’s decision to proceed with 1 of the 2 procedures. Moreover, we evaluated clinical outcomes in these 2 patient populations. We hypothesized that (1) patients selected for rTSA would have worse preoperative function, less range of motion, more comorbidities, more evidence of radiographic subluxation, and a higher likelihood of having undergone previous RCR than those selected for RCR, and (2) both RCR and rTSA would be successful and result in improved clinical outcomes with high patient satisfaction.
MATERIALS AND METHODS
PATIENT SELECTION
We performed a retrospective chart review using our practice database of all patients undergoing arthroscopic RCR and rTSA for any indication by the senior author (M.A.F.) between January 2004 and April 2015. A total of 1503 RCRs and 1973 rTSAs were conducted during the study period. Patient medical records were reviewed, and those meeting the following criteria were included in the study: >65 years at the time of surgery, MCT, no preoperative glenohumeral arthritis, minimum follow-up of 12 months, functional deltoid muscle on physical examination, and no prior shoulder surgery except for RCR or diagnostic arthroscopy. A total of 92 patients who underwent arthroscopic RCR and 89 patients who underwent rTSA met the inclusion criteria. For patients with bilateral shoulder surgery, we measured each shoulder independently. Three patients underwent bilateral rTSA, and 3 patients underwent bilateral RCR, leaving 95 shoulders in the RCR group and 92 in the rTSA group. The Western Institutional Review Board determined this study to be exempt from review.
RADIOGRAPHIC EVALUATION
All patient charts included a radiology report and documented interpretation of the images by the treating surgeon prior to surgery. Radiographs were assessed to assure the absence of preoperative glenohumeral osteoarthritis. The images were also graded based on the Hamada classification.11 Stage 1 is associated with minimal radiographic change with an acromiohumeral interval (AHI) >6 mm; stage 2 is characterized by narrowing of the AHI <6 mm; and Stage 3 is defined by narrowing of the AHI with radiographic changes of the acromion. Stages 4 and higher include arthritic changes to the glenohumeral joint, and they were not included in the study population. The AHI measurements and the presence or absence of glenohumeral subluxation were documented.
Continue to: MASSIVE CUFF TEAR DETERMINATION...
MASSIVE CUFF TEAR DETERMINATION
We defined MCT on the basis of previously described criteria of tears involving ≥2 tendons or tears measuring ≥5 cm in greatest dimension.12,13 Patient charts were screened, and those whose clinical notes or radiology reports indicated an absence of MCT were excluded. Preoperative imaging of the remaining patients was then evaluated by 3 fellowship-trained shoulder surgeons to confirm MCT in all patients with a clinically documented MCT, as well as to assess those who had insufficient documentation of tear size in the notes.
Advanced imaging was evaluated for fatty atrophy of the rotator cuff musculature, and Goutallier classification was assigned.14,15 Length of retraction was measured from the tendon end to the medial aspect of the footprint on coronal imaging, and the subscapularis and teres minor were assessed and documented as torn or intact.16,17
DATA COLLECTION
We reviewed clinical charts and patient questionnaire forms from both the preoperative and follow-up visits. Clinical data collected included gender, age at surgery, active range of motion (forward elevation, abduction, external rotation, and internal rotation), comorbidities, smoking status, BMI, history of shoulder surgery, and any postoperative complications or need for secondary surgery. All patients completed patient-centered questionnaires regarding shoulder pain and dysfunction at each visit or via telephone communication with clinic staff. Outcome measurements used for analysis included American Shoulder and Elbow Surgeons (ASES) Score, simple shoulder test (SST), visual analog score (VAS) pain scale, and patient-reported satisfaction (Graded 1-10; 1 = poor outcome; 4 = satisfactory outcome; 7 = good outcome; 10 = excellent outcome).
DATA ANALYSIS AND STATISTICAL METHODS
Statistical tests were selected based on the result of Shapiro–Wilk test for normality. Continuous variables were evaluated with either independent t test or Mann–Whitney U test. Dependent t test was used to evaluate outcome variables. For categorical variables, either Pearson’s χ2or Fisher’s exact test was performed depending on the sample size. Alpha was set at P =.05.
Continue to: RESULTS...
RESULTS
PREOPERATIVE CHARACTERISTICS
Of the 187 shoulders in the study group, 95 had RCR and 92 had rTSA. Demographic information and preoperative variables for both groups are summarized in Table 1 and Table 2. Patients in the RCR group had greater preoperative forward elevation, abduction, and external rotation and higher preoperative functional scores than those in the rTSA group. Patients in the rTSA group were older and more likely to be female than those in the RCR group. More patients in the rTSA group had undergone prior RCR compared with those in the RCR group. Each of these differences was statistically significant. Subjective pain scores, BMI, and comorbidities were similar between the 2 groups.
Table 1. Patient demographics
| RCR | rTSA | P value |
Age (yr; mean ± SD) | 71 ± 5 | 74 ± 6 | <.0001 |
Gender *male (no.; %) *female (no.; %) | 57 (60%) 38 (40%) | 30 (33%) 62 (67%) | <.0001 |
BMI (mean ± SD) | 28.5 ± 4.4 | 28.1 ± 4.5 | .578 |
Abbreviations: BMI, body mass index; RCR, rotator cuff repair; rTSA, reverse total shoulder arthroplasty.
Table 2. Preoperative variables
| RCR (n=95) | rTSA (n=92) | P value |
Radiographic parameters | |||
AB interval | 9 ± 3 | 5 ± 3 | <.0001 |
Humeral escape | 14.1% | 50.6% | <.0001 |
Hamada 1 | 76.1% | 15.6% | <.0001 |
Hamada 2 | 13.0% | 50.6% | |
Hamada 3 | 10.9% | 33.8% | |
Goutallier grade 1 | 7.8% | 19.3% | .227 |
Goutallier grade 2 | 66.7% | 52.6% | |
Goutallier grade 3 | 21.6% | 19.3% | |
Goutallier grade 4 | 3.9% | 8.8% | |
Clinical measures | |||
Preop FE | 113 ± 50 | 57 ± 34 | <.0001 |
Preop AB | 97 ± 45 | 53 ± 35 | <.0001 |
Preop ER | 42 ± 25 | 32 ± 28 | .029 |
Preop IR | 2.9 ± 1.6 | 2.6 ± 1.8 | .247 |
Preop pain | 5.7 ± 2.3 | 5.6 ± 2.5 | .927 |
Preop ASES | 44 ± 17 | 39 ± 16 | .04 |
Preop SST | 3.1 ± 2.6 | 1.9 ± 1.7 | .001 |
Patients parameters | |||
Previous cuff surgery | 6.3% | 35.9% | <.0001 |
Comorbidity count | 1.7 ± 1.4 | 2.1 ± 2.7 | .126 |
Abbreviations: AB, abduction; ASES, American Shoulder and Elbow Society score; ER, external rotation; FE, forward elevation; IR, internal rotation; preop, preoperative; SST, simple shoulder test.
Radiographically, patients selected to undergo rTSA had a smaller AHI (4.8 vs 8.7, P < .0001) and more evidence of superior subluxation (50.6% vs 14.1%, P < .0001) than those in the RCR group. Average Hamada grade was 1.4 ± 0.7 and 2.2 ± 0.7 for the RCR and rTSA groups, respectively (P < .0001). Average Goutallier grade was similar between the groups (2.2 ± 0.6 for RCR vs 2.2 ± 0.8 for rTSA, P =.227), and 25.5% of the RCR group had Grade 3 or 4 atrophy compared with 28.1% of the rTSA group.
POSTOPERATIVE OUTCOMES
The average follow-up time was 44 months for RCR and 47 months for rTSA. Patients in the RCR and rTSA groups were highly satisfied with the surgery (8.5 ± 2.6 vs 8.2 ± 2.6, P = .461) and had significantly increased range of motion in all planes and improved functional scores (Table 3). The rTSA group had greater net improvement in forward elevation, abduction, and external rotation than the RCR group. Both groups demonstrated similar improvement in ASES, SST, and VAS pain scores.
Table 3. Postoperative outcomes
| RCR (n=95) | P value | rTSA (n=92) | P value | ||
Preoperative | Postoperative | Preoperative | Postoperative | |||
FE | 113 ± 50 | 166 ± 26 | <.0001 | 57 ± 34 | 136 ± 46 | <.0001 |
AB | 97 ± 45 | 155 ± 37 | <.0001 | 53 ± 35 | 129 ± 44 | <.0001 |
ER | 42 ± 25 | 48 ± 20 | .033 | 32 ± 28 | 57 ± 32 | <.0001 |
IR | 2.9 ± 1.6 | 4.6 ± 1.6 | <.0001 | 2.6 ± 1.8 | 4.7 ± 2.4 | <.0001 |
VAS pain | 5.7 ± 2.3 | 1.7 ± 2.4 | <.0001 | 5.6 ± 2.5 | 1.6 ± 2.5 | <.0001 |
ASES | 44 ± 17 | 83 ± 18 | <.0001 | 39 ± 16 | 77 ± 22 | <.0001 |
SST | 3.1 ± 2.6 | 9.3 ± 2.9 | <.0001 | 1.9 ± 1.7 | 7.1 ± 3.4 | <.0001 |
Abbreviations: AB, abduction; ASES, American Shoulder and Elbow Society score; ER, external rotation; FE, forward elevation; IR, internal rotation; SST, simple shoulder test; VAS – visual analog score.
In the RCR group, 5 patients (5.3%) required reoperation: 3 patients underwent conversion to rTSA, 1 patient underwent biceps tenotomy with subacromial decompression, and 1 patient underwent arthroscopic irrigation and debridement for a postoperative Propionibacterium acnes infection. In the rTSA group, 2 patients (2.2%) required reoperation: 1 patient underwent open reduction internal fixation for a scapula fracture that failed conservative management, and 1 patient had an open irrigation and debridement with polyethylene exchange for an acute postoperative infection of unknown source.
DISCUSSION
Massive, retracted rotator cuff tears are a common and difficult problem.1 The treatment options are numerous and depend on a variety of preoperative factors including patient-specific characteristics and factors specific to the tear. For certain patients, nonoperative management may be a reasonable first step, as an MCT does not necessarily preclude painless, functional shoulder motion. Elderly, lower demand individuals have been shown to do well with physical rehabilitation.18 Similarly, for the same category of elderly patients who do not respond to conservative measures, arthroscopic tendon debridement with or without subacromial decompression and/or biceps tenotomy may be effective.1,19 This technique has been described as “limited goals surgery;” despite some mixed results in the literature, multiple studies have reported symptomatic and functional improvement after simple debridement.2,19–21The consensus among several authors has been that this procedure continues to play a role for elderly, low-demand patients whose functional goals are limited and whose primary complaint is pain.1,2,20
For the majority of patients with MCT who desire pain relief and a restoration of shoulder function, RCR remains the gold standard of treatment and should be the primary aim if feasible. Complete RCR has consistently outperformed both partial repair and debridement in multiple studies in terms of pain relief and functional improvement.10,21,22However, elderly patients with chronic, massive tears, particularly in the setting of muscle atrophy, are at high risk of failure with attempted cuff repair.9,23 Novel techniques such as superior capsular reconstruction and subacromial balloon spacer implantation may offer a minimally invasive method of re-centering the humeral head and stabilizing the glenohumeral joint; however, these new treatment options lack any long-term data in the literature to support their widespread use.24–26 Alternatively, rTSA has been shown to be a reliable option to restore shoulder function in the setting of a massive irreparable rotator cuff tear, even in the absence of arthritis.5,27-31
Continue to: The decision-making process...
The decision-making process for selecting RCR or rTSA in the setting of MCT without arthritis in the older population (age >65 years) remains challenging. We attempted to quantify the data of a high-volume surgeon and identify the differences and similarities between those patients selected for either procedure. At our institution, we generally performed rTSA on patients with low preoperative range of motion, poor function based on SST and ASES scores, small AHI, and strong evidence of superior subluxation. We were also more likely to perform rTSA if the patient had a history of rotator cuff surgery. There was a predilection for older age and female gender in those who underwent rTSA.
For our study, we elected to focus on patients >65 years. In our experience, the choice of which surgical procedure to perform is generally easier in younger patients. Most surgeons appropriately opt for an arthroscopic procedure or tendon transfer to preserve bone and maintain the option of rTSA as a salvage procedure if necessary in the future. Studies have reported that <60 years is a predictor of poor outcome with rTSA, and patients <65 years who undergo rTSA have been shown to have high complication rates.30-32 Furthermore, the longevity of the implant in young patients is a significant concern, and revision surgery after rTSA is technically demanding and known to result in poor functional outcomes.32,33
Although the indications for rTSA are expanding, attempts at RCR in the setting of MCT remain largely appropriate. Preserved preoperative anterior elevation >90° has been associated with loss of motion after rTSA and poor satisfaction, and one should exercise caution when considering rTSA in this setting.3 The current study confirmed that even older patients with MCT may be very satisfied with arthroscopic RCR (Figure 1). Both range of motion and function significantly improved, and patients were largely satisfied with the procedure with an average self-reported outcome of good to excellent. At the time of final follow-up for this study, only 3 shoulders in the RCR group had undergone conversion to rTSA. This number may be expected to rise with long follow-up periods, and we feel that prolonging the time before arthroplasty is generally in the best interest of the patient.
Our results were consistent with several reported studies in which RCR has been shown to be successful in the setting of MCT.34–37 Henry and colleagues36 performed a systematic review that evaluated 954 patients who underwent partial or complete anatomic RCR for MCT. Although the average age was 63 years (range, 37–87), functional outcome scores, VAS pain score, and overall range of motion consistently and significantly improved.
rTSA may be a “more reliable” option than RCR in treating MCT in the older population because it does not rely on tendon healing. However, the relationship between tendon healing and clinical outcomes after RCR is unclear. The aforementioned systematic review reported re-tear rates to be as high as 79%, but several studies have reported high satisfaction even in the setting of retear.36 Yoo and colleagues38 and Chung and colleagues9 reported re-tear rates of 45.5% and 39.8%, respectively, but both studies noted that there was no difference in outcome measures between those patients with and without re-tears. In particular, for patients who have had no prior rotator cuff surgery, an attempt at arthroscopic repair may be a prudent option with relatively low risk.
Although certain patients may clinically improve despite suffering a re-tear (or inability to heal in the first place), others continue to experience pain and dysfunction that negatively affect their quality of life.39–41 These patients are more often appropriate candidates for rTSA. Indeed, several studies have demonstrated a higher re-tear rate in patients with a history of surgery than in those without.23,31,38,42 Shamsudin and colleagues43 found revision arthroscopic RCR, even in a younger age group with tears of all sizes, to be twice as likely to re-tear. Notably, re-tear after revision repair may be more likely to be symptomatic, as these re-tears are routinely associated with pain, stiffness, and loss of function. Even in the hands of experienced surgeons in a younger population, revision repair has only been able to reverse pseudoparalysis in 43% of patients, leading to only 39% return to sport or full activity.44 In examining our data, we were much less likely to perform an RCR in patients who had a history of cuff repair surgery than in those without this history.
Continue to: Overall, those patients selected for rTSA...
Overall, those patients selected for rTSA in our study population performed well postoperatively (Figure 2 and Figure 3). Vast improvements were noted in range of motion, function, and pain scores at final follow up. Moreover, no patients in the study group required revision arthroplasty during the follow-up period. Although the average follow-up period was only 47 months, these results suggested that elderly patients with MCT without arthritis may be particularly ideal candidates for rTSA with regard to implant survival and anticipated revision rate when chosen appropriately.
Several weaknesses were noted within this paper. First, the study was retrospective, precluding randomization of treatment groups and standardization of data collection and follow-up. The outcomes of RCR and rTSA could not be compared directly due to the inherent selection bias. The groups clearly differed in many respects, and these preoperative factors likely played a role in postoperative outcomes. However, the primary goal of this study was not to compare outcomes of the treatment groups but to analyze the patterns of patient selection by an experienced treating surgeon and contribute to published data that each surgery can be successful in this patient population when chosen appropriately.
Second, our data were based on a single surgeon’s decisions, and results may not be generalizable. Furthermore, the senior author has had a longstanding interest in reverse shoulder arthroplasty and has published data illustrating successful outcomes for rTSA in patients with MCT. For this reason, one could presume that there may have been some bias toward treating patients with rTSA. However, we feel that the senior author’s unique and longstanding experience in treating MCT allows for a thorough evaluation and comparison of preoperative variables and outcomes declared within this study. Indeed, many patients included in this study were referred from outside institutions specifically for rTSA but instead were deemed more appropriate candidates for RCR and underwent successful arthroscopic repair, a common scenario which served as an impetus for this study.
CONCLUSION
RCR and rTSA are both viable options for patients >65 years with MCT without arthritis. Treatment must be individualized for each patient with careful consideration of a number of preoperative variables and patient characteristics. At our institution, patients with previous RCR, decreased range of motion, poor function, and strong radiographic evidence of subluxation are more likely to undergo rTSA. When chosen appropriately, both RCR and rTSA can result in improved range of motion, function, and high patient satisfaction in this patient population.
- Bedi A, Dines J, Warren RF, Dines DM. Massive tears of the rotator cuff. J Bone Joint Surg Am. 2010;92:1894-1908. doi:10.2106/JBJS.I.01531.
- Greenspoon JA, Petri M, Warth RJ, Millett PJ. Massive rotator cuff tears: pathomechanics, current treatment options, and clinical outcomes. J Shoulder Elbow Surg. 2015;24:1493-1505. doi:10.1016/j.jse.2015.04.005.
- Boileau P, Gonzalez JF, Chuinard C, Bicknell R, Walch G. Reverse total shoulder arthroplasty after failed rotator cuff surgery. J Shoulder Elbow Surg. 2009;18:600-606. doi:10.1016/j.jse.2009.03.011.
- Cuff D, Pupello D, Virani N, Levy J, Frankle M. Reverse shoulder arthroplasty for the treatment of rotator cuff deficiency. J Bone Joint Surg Am. 2008;90:1244-1251. doi:10.2106/JBJS.G.00775.
- Mulieri P, Dunning P, Klein S, Pupello D, Frankle M. Reverse shoulder arthroplasty for the treatment of irreparable rotator cuff tear without glenohumeral arthritis. J Bone Joint Surg Am. 2010;92:2544-2556.doi:10.2106/JBJS.I.00912.
- Wall B, Nove-Josserand L, O'Connor DP, Edwards TB, Walch G. Reverse total shoulder arthroplasty: a review of results according to etiology. J Bone Joint Surg Am. 2007;89:1476-1485. doi:10.2106/JBJS.F.00666.
- Pill SG, Walch G, Hawkins RJ, Kissenberth MJ. The role of the biceps tendon in massive rotator cuff tears. Instr Course Lect. 2012;61:113-120.
- Makhni EC, Swart E, Steinhaus ME, Mather RC 3rd, Levine WN, Bach BR Jr et al. Cost-effectiveness of reverse total shoulder arthroplasty versus arthroscopic rotator cuff repair for symptomatic large and massive rotator cuff tears. Arthroscopy. 2016;32(9):1771-1780. doi:10.1016/j.arthro.2016.01.063.
- Chung SW, Kim JY, Kim MH, Kim SH, Oh JH. Arthroscopic repair of massive rotator cuff tears: outcome and analysis of factors associated with healing failure or poor postoperative function. Am J Sports Med. 2013;41:1674-1683. doi:10.1177/0363546513485719.
- Holtby R, Razmjou H. Relationship between clinical and surgical findings and reparability of large and massive rotator cuff tears: a longitudinal study. BMC Musculoskelet Disord. 2014;15:180. doi:10.1186/1471-2474-15-180.
- Hamada K, Fukuda H, Mikasa M, Kobayashi Y. Roentgenographic findings in massive rotator cuff tears. A long-term observation. Clin Orthop Relat Res. 1990;254:92-96.
- DeOrio JK, Cofield RH. Results of a second attempt at surgical repair of a failed initial rotator-cuff repair. J Bone Joint Surg Am. 1984;66:563-567.
- Gerber C, Fuchs B, Hodler J. The results of repair of massive tears of the rotator cuff. J Bone Joint Surg Am. 2000;82:505-515.
- Fuchs B, Weishaupt D, Zanetti M, Hodler J, Gerber C. Fatty degeneration of the muscles of the rotator cuff: assessment by computed tomography versus magnetic resonance imaging. J Shoulder Elbow Surg. 1999;8:599-605.
- Goutallier D, Bernageau J, Patte D. Assessment of the trophicity of the muscles of the ruptured rotator cuff by CT scan. In: Post M, Morrey B, Hawkins R, eds. Surgery of the Shoulder. St. Louis, MO: Mosby, 1990;11-13.
- Meyer DC, Farshad M, Amacker NA, Gerber C, Wieser K. Quantitative analysis of muscle and tendon retraction in chronic rotator cuff tears. Am J Sports Med. 2012;40(3):606-610.
- Meyer DC, Wieser K, Farshad M, Gerber C. Retraction of supraspinatus muscle and tendon as predictors of success of rotator cuff repair. Am J Sports Med. 2012;40:2242-2247.
- Williams GR Jr, Rockwood CA Jr, Bigliani LU, Ianotti JP, Stanwood W. Rotator cuff tears: why do we repair them? J Bone Joint Surg Am. 2004;86-A(12):2764-2776.
- Rockwood CA Jr, Williams GR Jr, Burkhead WZ Jr. Debridement of degenerative, irreparable lesions of the rotator cuff. J Bone Joint Surg Am. 1995;77:857-866.
- Berth A, Neumann W, Awiszus F, Pap G. Massive rotator cuff tears: functional outcome after debridement or arthroscopic partial repair. J Orthopaed Traumatol. 2010;11:13-20. doi 10.1007/s10195-010-0084-0.
- Heuberer PR, Kolblinger R, Buchleitner S, Pauzenberger L, Laky B, Auffarth A, et al. Arthroscopic management of massive rotator cuff tears: an evaluation of debridement, complete, and partial repair with and without force couple restoration. Knee Surg Sports Traumatol Arthrosc. 2016;24:3828-3837.
- Moser M, Jablonski MV, Horodyski M, Wright TW. Functional outcome of surgically treated massive rotator cuff tears: a comparison of complete repair, partial repair, and debridement. Orthopedics.2007;30(6):479-482.
- Rhee YG, Cho NS, Yoo JH. Clinical outcome and repair integrity after rotator cuff repair in patients older than 70 years versus patients younger than 70 years. Arthroscopy. 2014;30:546-554. doi:10.1016/j.arthro.2014.02.006.
- Denard PJ, Brady PC, Adams CR, Tokish JM, Burkhart SS. Preliminary results of arthroscopic superior capsule reconstruction with dermal allograft. Arthroscopy. 2018;34(1):93-99. doi: 10.1016/j.arthro.2017.08.265.
- Mihata T, Lee TQ, Watanabe C, Fukunishi K, Ohue M, Tsujimura T, Kinoshita M. Clinical results of arthroscopic superior capsule reconstruction for irreparable rotator cuff tears. Arthroscopy.2013;29:459-70.
- Piekaar RSM, Bouman ICE, van Kampen PM, van Eijk F, Huijsmans PE. Early promising outcome following arthroscopic implantation of the subacromial balloon spacer for treating massive rotator cuff tear. Musculoskelet Surg. 2018;102(3):247-255. doi: 10.1007/s12306-017-0525-5.
- Al-Hadithy N, Domos P, Sewell MD, Pandit R. Reverse shoulder arthroplasty in 41 patients with cuff tear arthropathy with a mean follow-up period of 5 years. J Shoulder Elbow Surg. 2014;23:1662-1668. doi:10.1016/j.jse.2014.03.001.
- Boileau P, Watkinson DJ, Hatzidakis AM, Balg F. Grammont reverse prosthesis: design, rationale, and biomechanics. J Shoulder Elbow Surg. 2005;14:147S-161S. doi:10.1016/j.jse.2004.10.006.
- Grammont PM, Baulot E. Delta shoulder prosthesis for rotator cuff rupture. Orthopedics 1993;16:65-68. doi: 10.3928/0147-7447-19930101-11.
- Hartzler RU, Steen BM, Hussey MM, Cusick MC, Cottrell BJ, Clark RE, Frankle MA. Reverse shoulder arthroplasty for massive rotator cuff tear: risk factors for poor functional improvement. J Shoulder Elbow Surg. 2015;24:1698-1706. doi:10.1016/j.jse.2015.04.015.
- Kim HM, Caldwell JM, Buza JA, Fink LA, Ahmad CS, Bigliani LU, Levine WN. Factors affecting satisfaction and shoulder function in patients with a recurrent rotator cuff tear. J Bone Joint Surg Am. 2014;96:106-112. doi:10.2106/JBJS.L.01649.
- Ek ET, Neukom L, Catanzaro S, Gerber C. Reverse total shoulder arthroplasty for massive irreparable rotator cuff tears in patients younger than 65 years old: results after five to fifteen years. J Shoulder Elbow Surg. 2013;22:1199-1208. doi:10.1016/j.jse.2012.11.016.
- Sershon RA, Van Thiel GS, Lin EC, McGill KC, Cole BJ, Verma NN, et al. Clinical outcomes of reverse total shoulder arthroplasty in patients aged younger than 60 years. J Shoulder Elbow Surg.2014;23:395-400. doi:10.1016/j.jse.2013.07.047.
- Denard PJ, Ladermann A, Jiwani AZ, Burkhart SS. Functional outcome after arthroscopic repair of massive rotator cuff tears in individuals with pseudoparalysis. Arthroscopy. 2012;28:1214-1219. doi:10.1016/j.arthro.2012.02.026.
- Denard PJ, Ladermann A, Brady PC, Narbona P, Adams CR, Arrigoni P, et al. Pseudoparalysis from a massive rotator cuff tear is reliably reversed with an arthroscopic rotator cuff repair in patients without preoperative glenohumeral arthritis. Am J Sports Med. 2015;43:2373-2378. doi: 10.1177/0363546515597486.
- Henry P, Wasserstein D, Park S, Dwyer T, Chahal J, Slobogean G, Schemitsch E. Arthroscopic repair for chronic massive rotator cuff tears: a systematic review. Arthroscopy. 2015;31:2472-2480. doi:10.1016/j.arthro.2015.06.038.
- Oh JH, Kim SH, Shin SH, Chung SW, Kim JY, Kim SJ. Outcome of rotator cuff repair in large-to-massive tear with pseudoparalysis: a comparative study with propensity score matching. Am J Sports Med.2011;39:1413-1420.
- Yoo JC, Ahn JH, Koh KH, Lim KS. Rotator cuff integrity after arthroscopic repair for large tears with less-than-optimal footprint coverage. Arthroscopy. 2009;25:1093-1100. doi:10.1016/j.arthro.2009.07.010.
- Jost B, Pfirrmann CW, Gerber C, Switzerland Z. Clinical outcome after structural failure of rotator cuff repairs. J Bone Joint Surg Am. 2000;82:304-314.
- Klepps S, Bishop J, Lin J, Cahlon O, Strauss A, Hayes P, Flatow EL Prospective evaluation of the effect of rotator cuff integrity on the outcome of open rotator cuff repairs. Am J Sports Med. 2004;32:1716-1722.
- Liu SH, Baker CL. Arthroscopically assisted rotator cuff repair: correlation of functional results with integrity of the cuff. Arthroscopy. 1994;10:54-60.
- Papadopoulos P, Karataglis D, Boutsiadis A, Fotiadou A, Christoforidis J, Christodoulou A. Functional outcome and structural integrity following mini-open repair of large and massive rotator cuff tears: a 3-5 year follow-up study. J Shoulder Elbow Surg. 2011;20:131-137. doi:10.1016/j.jse.2010.05.026.
- Shamsudin A, Lam PH, Peters K, Rubenis I, Hackett L, Murrell GA. Revision versus primary arthroscopic rotator cuff repair: a 2-year analysis of outcomes in 360 patients. Am J Sports Med.2015;43:557-564. doi:10.1177/0363546514560729.
- Ladermann A, Denard PJ, Burkhart SS. Midterm outcome of arthroscopic revision repair of massive and nonmassive rotator cuff tears. Arthroscopy. 2011;27:1620-1627. doi:10.1016/j.arthro.2011.08.290.
- Bedi A, Dines J, Warren RF, Dines DM. Massive tears of the rotator cuff. J Bone Joint Surg Am. 2010;92:1894-1908. doi:10.2106/JBJS.I.01531.
- Greenspoon JA, Petri M, Warth RJ, Millett PJ. Massive rotator cuff tears: pathomechanics, current treatment options, and clinical outcomes. J Shoulder Elbow Surg. 2015;24:1493-1505. doi:10.1016/j.jse.2015.04.005.
- Boileau P, Gonzalez JF, Chuinard C, Bicknell R, Walch G. Reverse total shoulder arthroplasty after failed rotator cuff surgery. J Shoulder Elbow Surg. 2009;18:600-606. doi:10.1016/j.jse.2009.03.011.
- Cuff D, Pupello D, Virani N, Levy J, Frankle M. Reverse shoulder arthroplasty for the treatment of rotator cuff deficiency. J Bone Joint Surg Am. 2008;90:1244-1251. doi:10.2106/JBJS.G.00775.
- Mulieri P, Dunning P, Klein S, Pupello D, Frankle M. Reverse shoulder arthroplasty for the treatment of irreparable rotator cuff tear without glenohumeral arthritis. J Bone Joint Surg Am. 2010;92:2544-2556.doi:10.2106/JBJS.I.00912.
- Wall B, Nove-Josserand L, O'Connor DP, Edwards TB, Walch G. Reverse total shoulder arthroplasty: a review of results according to etiology. J Bone Joint Surg Am. 2007;89:1476-1485. doi:10.2106/JBJS.F.00666.
- Pill SG, Walch G, Hawkins RJ, Kissenberth MJ. The role of the biceps tendon in massive rotator cuff tears. Instr Course Lect. 2012;61:113-120.
- Makhni EC, Swart E, Steinhaus ME, Mather RC 3rd, Levine WN, Bach BR Jr et al. Cost-effectiveness of reverse total shoulder arthroplasty versus arthroscopic rotator cuff repair for symptomatic large and massive rotator cuff tears. Arthroscopy. 2016;32(9):1771-1780. doi:10.1016/j.arthro.2016.01.063.
- Chung SW, Kim JY, Kim MH, Kim SH, Oh JH. Arthroscopic repair of massive rotator cuff tears: outcome and analysis of factors associated with healing failure or poor postoperative function. Am J Sports Med. 2013;41:1674-1683. doi:10.1177/0363546513485719.
- Holtby R, Razmjou H. Relationship between clinical and surgical findings and reparability of large and massive rotator cuff tears: a longitudinal study. BMC Musculoskelet Disord. 2014;15:180. doi:10.1186/1471-2474-15-180.
- Hamada K, Fukuda H, Mikasa M, Kobayashi Y. Roentgenographic findings in massive rotator cuff tears. A long-term observation. Clin Orthop Relat Res. 1990;254:92-96.
- DeOrio JK, Cofield RH. Results of a second attempt at surgical repair of a failed initial rotator-cuff repair. J Bone Joint Surg Am. 1984;66:563-567.
- Gerber C, Fuchs B, Hodler J. The results of repair of massive tears of the rotator cuff. J Bone Joint Surg Am. 2000;82:505-515.
- Fuchs B, Weishaupt D, Zanetti M, Hodler J, Gerber C. Fatty degeneration of the muscles of the rotator cuff: assessment by computed tomography versus magnetic resonance imaging. J Shoulder Elbow Surg. 1999;8:599-605.
- Goutallier D, Bernageau J, Patte D. Assessment of the trophicity of the muscles of the ruptured rotator cuff by CT scan. In: Post M, Morrey B, Hawkins R, eds. Surgery of the Shoulder. St. Louis, MO: Mosby, 1990;11-13.
- Meyer DC, Farshad M, Amacker NA, Gerber C, Wieser K. Quantitative analysis of muscle and tendon retraction in chronic rotator cuff tears. Am J Sports Med. 2012;40(3):606-610.
- Meyer DC, Wieser K, Farshad M, Gerber C. Retraction of supraspinatus muscle and tendon as predictors of success of rotator cuff repair. Am J Sports Med. 2012;40:2242-2247.
- Williams GR Jr, Rockwood CA Jr, Bigliani LU, Ianotti JP, Stanwood W. Rotator cuff tears: why do we repair them? J Bone Joint Surg Am. 2004;86-A(12):2764-2776.
- Rockwood CA Jr, Williams GR Jr, Burkhead WZ Jr. Debridement of degenerative, irreparable lesions of the rotator cuff. J Bone Joint Surg Am. 1995;77:857-866.
- Berth A, Neumann W, Awiszus F, Pap G. Massive rotator cuff tears: functional outcome after debridement or arthroscopic partial repair. J Orthopaed Traumatol. 2010;11:13-20. doi 10.1007/s10195-010-0084-0.
- Heuberer PR, Kolblinger R, Buchleitner S, Pauzenberger L, Laky B, Auffarth A, et al. Arthroscopic management of massive rotator cuff tears: an evaluation of debridement, complete, and partial repair with and without force couple restoration. Knee Surg Sports Traumatol Arthrosc. 2016;24:3828-3837.
- Moser M, Jablonski MV, Horodyski M, Wright TW. Functional outcome of surgically treated massive rotator cuff tears: a comparison of complete repair, partial repair, and debridement. Orthopedics.2007;30(6):479-482.
- Rhee YG, Cho NS, Yoo JH. Clinical outcome and repair integrity after rotator cuff repair in patients older than 70 years versus patients younger than 70 years. Arthroscopy. 2014;30:546-554. doi:10.1016/j.arthro.2014.02.006.
- Denard PJ, Brady PC, Adams CR, Tokish JM, Burkhart SS. Preliminary results of arthroscopic superior capsule reconstruction with dermal allograft. Arthroscopy. 2018;34(1):93-99. doi: 10.1016/j.arthro.2017.08.265.
- Mihata T, Lee TQ, Watanabe C, Fukunishi K, Ohue M, Tsujimura T, Kinoshita M. Clinical results of arthroscopic superior capsule reconstruction for irreparable rotator cuff tears. Arthroscopy.2013;29:459-70.
- Piekaar RSM, Bouman ICE, van Kampen PM, van Eijk F, Huijsmans PE. Early promising outcome following arthroscopic implantation of the subacromial balloon spacer for treating massive rotator cuff tear. Musculoskelet Surg. 2018;102(3):247-255. doi: 10.1007/s12306-017-0525-5.
- Al-Hadithy N, Domos P, Sewell MD, Pandit R. Reverse shoulder arthroplasty in 41 patients with cuff tear arthropathy with a mean follow-up period of 5 years. J Shoulder Elbow Surg. 2014;23:1662-1668. doi:10.1016/j.jse.2014.03.001.
- Boileau P, Watkinson DJ, Hatzidakis AM, Balg F. Grammont reverse prosthesis: design, rationale, and biomechanics. J Shoulder Elbow Surg. 2005;14:147S-161S. doi:10.1016/j.jse.2004.10.006.
- Grammont PM, Baulot E. Delta shoulder prosthesis for rotator cuff rupture. Orthopedics 1993;16:65-68. doi: 10.3928/0147-7447-19930101-11.
- Hartzler RU, Steen BM, Hussey MM, Cusick MC, Cottrell BJ, Clark RE, Frankle MA. Reverse shoulder arthroplasty for massive rotator cuff tear: risk factors for poor functional improvement. J Shoulder Elbow Surg. 2015;24:1698-1706. doi:10.1016/j.jse.2015.04.015.
- Kim HM, Caldwell JM, Buza JA, Fink LA, Ahmad CS, Bigliani LU, Levine WN. Factors affecting satisfaction and shoulder function in patients with a recurrent rotator cuff tear. J Bone Joint Surg Am. 2014;96:106-112. doi:10.2106/JBJS.L.01649.
- Ek ET, Neukom L, Catanzaro S, Gerber C. Reverse total shoulder arthroplasty for massive irreparable rotator cuff tears in patients younger than 65 years old: results after five to fifteen years. J Shoulder Elbow Surg. 2013;22:1199-1208. doi:10.1016/j.jse.2012.11.016.
- Sershon RA, Van Thiel GS, Lin EC, McGill KC, Cole BJ, Verma NN, et al. Clinical outcomes of reverse total shoulder arthroplasty in patients aged younger than 60 years. J Shoulder Elbow Surg.2014;23:395-400. doi:10.1016/j.jse.2013.07.047.
- Denard PJ, Ladermann A, Jiwani AZ, Burkhart SS. Functional outcome after arthroscopic repair of massive rotator cuff tears in individuals with pseudoparalysis. Arthroscopy. 2012;28:1214-1219. doi:10.1016/j.arthro.2012.02.026.
- Denard PJ, Ladermann A, Brady PC, Narbona P, Adams CR, Arrigoni P, et al. Pseudoparalysis from a massive rotator cuff tear is reliably reversed with an arthroscopic rotator cuff repair in patients without preoperative glenohumeral arthritis. Am J Sports Med. 2015;43:2373-2378. doi: 10.1177/0363546515597486.
- Henry P, Wasserstein D, Park S, Dwyer T, Chahal J, Slobogean G, Schemitsch E. Arthroscopic repair for chronic massive rotator cuff tears: a systematic review. Arthroscopy. 2015;31:2472-2480. doi:10.1016/j.arthro.2015.06.038.
- Oh JH, Kim SH, Shin SH, Chung SW, Kim JY, Kim SJ. Outcome of rotator cuff repair in large-to-massive tear with pseudoparalysis: a comparative study with propensity score matching. Am J Sports Med.2011;39:1413-1420.
- Yoo JC, Ahn JH, Koh KH, Lim KS. Rotator cuff integrity after arthroscopic repair for large tears with less-than-optimal footprint coverage. Arthroscopy. 2009;25:1093-1100. doi:10.1016/j.arthro.2009.07.010.
- Jost B, Pfirrmann CW, Gerber C, Switzerland Z. Clinical outcome after structural failure of rotator cuff repairs. J Bone Joint Surg Am. 2000;82:304-314.
- Klepps S, Bishop J, Lin J, Cahlon O, Strauss A, Hayes P, Flatow EL Prospective evaluation of the effect of rotator cuff integrity on the outcome of open rotator cuff repairs. Am J Sports Med. 2004;32:1716-1722.
- Liu SH, Baker CL. Arthroscopically assisted rotator cuff repair: correlation of functional results with integrity of the cuff. Arthroscopy. 1994;10:54-60.
- Papadopoulos P, Karataglis D, Boutsiadis A, Fotiadou A, Christoforidis J, Christodoulou A. Functional outcome and structural integrity following mini-open repair of large and massive rotator cuff tears: a 3-5 year follow-up study. J Shoulder Elbow Surg. 2011;20:131-137. doi:10.1016/j.jse.2010.05.026.
- Shamsudin A, Lam PH, Peters K, Rubenis I, Hackett L, Murrell GA. Revision versus primary arthroscopic rotator cuff repair: a 2-year analysis of outcomes in 360 patients. Am J Sports Med.2015;43:557-564. doi:10.1177/0363546514560729.
- Ladermann A, Denard PJ, Burkhart SS. Midterm outcome of arthroscopic revision repair of massive and nonmassive rotator cuff tears. Arthroscopy. 2011;27:1620-1627. doi:10.1016/j.arthro.2011.08.290.
TAKE-HOME POINTS
- Rotator cuff repair and reverse total shoulder arthroplasty are both viable options for patients >65 years with massive rotator cuff tears without arthritis.
- Treatment must be individualized for each patient, with careful consideration of a number of preoperative variables and patient characteristics.
- At our institution, patients with previous rotator cuff repair, decreased range of motion, poor function, and strong radiographic evidence of subluxation were more likely to undergo reverse total shoulder arthroplasty.
- Patients selected for rotator cuff repair had greater preoperative flexion, abduction, and external rotation, as well as higher functional scores, and were less likely to have had previous cuff surgery.
- When chosen appropriately, both rotator cuff repair and reverse total shoulder arthroplasty can result in improved range of motion, function, and high patient satisfaction in this patient population.
Patellofemoral Instability in the Skeletally Immature Patient: A Review and Technical Description of Medial Patellofemoral Ligament Reconstruction in Patients with Open Physes
ABSTRACT
Patellofemoral instability commonly occurs in the young patient, and, often, skeletal immaturity may be a risk factor for possible recurrence. Treatment considerations, including operative and nonoperative management, are based on anatomic factors. A medial patellofemoral ligament (MPFL) reconstruction is a treatment option for a skeletally immature patient with recurrent instability or for patients with a high risk of patellofemoral instability recurrence. A physeal-sparing MPFL reconstruction technique that considers the origin of the MPFL to be distal to the distal femoral physis may be employed.
Continue to: Patellofemoral instability (PFI)...
Patellofemoral instability (PFI), 1 of the most common patellofemoral disorders observed in skeletally immature patients,1-4 has a reported incidence of 43 per 100,000 skeletally immature patients.3 The incidence is even higher in patients 9 to 15 years, with dislocations occurring in 107 per 100,000 individuals.5,6 In recent years, there has been an increasing interest in studying PFI in skeletally immature pediatric patients, who are particularly susceptible to recurrent dislocations. Studies have indicated that children <16 years are at the highest risk for recurrence.7 Lewallen and colleagues8 noted a 69% failure rate and a 3-fold increase in the probability of recurrent dislocation in skeletally immature patients treated nonoperatively.
Anatomic factors that contribute to PFI include ligament laxity, trochlear dysplasia, patella alta, excessive femoral anteversion or tibial torsion, genu valgum, and increased tibial tubercle-to-trochlear groove distance.1,3,8-11 When considering surgical treatment for PFI, all anatomic factors should be considered, with an emphasis on, and understanding of, the role of residual growth and development. One must also consider balancing static soft tissue, dynamic soft tissue, and osseous constraints of the patellofemoral joint to optimize the overall health and balance of the patella (Figure 1).
CHALLENGES IN TREATING PEDIATRIC PFI
A primary challenge in the treatment of PFI in pediatric patients is accounting for the impact of anatomic changes occurring secondary to growth and maturity. From birth to adulthood, the collagen composition of soft tissue changes from an elastic type III collagen to a stiffer type I collagen.12 These physiologic changes may influence the rigidity of the soft tissue restraints around the patellofemoral joint during periods of rapid growth. Longitudinal growth and rotational changes can also occur at the distal femoral and proximal tibial physes. The position of the tibial tubercle and the magnitude of femoral anteversion may also change after growth in adolescents.
Developing effective technical analogs of surgical procedures performed in mature patients with PFI for use in skeletally immature patients has been a second challenge. For example, a varus-producing distal femoral osteotomy to address genu valgum and PFI13 is contraindicated in immature patients, when a hemiepiphysiodesis for guided growth should be considered.14 Similarly, a periosteal sleeve medialization of the insertion of the patellar tendon may be used instead of a tibial tubercle transfer.15
If a medial patellofemoral ligament (MPFL) reconstruction is considered in immature patients, careful consideration of the position of the MPFL in relation to the distal femoral physis is paramount. Shea and colleagues16 originally described the position of the MPFL, based on Schottle’s point17 on lateral radiographs, to be proximal to the distal femoral physis (Figure 2). However, due to the undulation of the physis, the lateral projection may falsely demonstrate Schottle’s point to be on or just proximal to the physis. Other researchers have evaluated the position of the distal femoral physis to be proximal to the origin of the MPFL by a range of 2.9 mm to 8.5 mm on AP radiograph and MRI, respectively.18,19 More recent cadaveric studies have demonstrated the origin of the MPFL in pediatric specimens in relation to the physis to be variable.20 Although we believe that a relative anatomic femoral position of the MPFL origin can be achieved without disrupting the physis using Schottle’s point, some have indicated concerns that this may produce a nonisometric position, which has not been our experience.21
Continue to: TREATMENT OPTIONS...
TREATMENT OPTIONS
The evaluation of a skeletally immature patient may differ from that of an adult patient. (Table 1) represents a standard evaluation of a skeletally immature patient with PFI. The injury, physical examination findings, activity level, presence of an osteochondral fracture, and severity of bony dysplasia may indicate surgical treatment and influence selection of the surgical technique. Indications for surgical treatment include recurrent patellar instability, symptomatic patellar instability, the presence of a chondral or an osteochondral fracture, and a primary patella dislocation in patients with a high risk for recurrent dislocations. Table 2 represents a list of the possible surgical treatment options for the skeletally immature patient with PFI.21,22 Variable outcomes and recurrence rates are noted with each procedure.21,22
MPFL REPAIR VS RECONSTRUCTION
Although there is increased consensus for restoring normal anatomy, continued controversy exists regarding the utility of an MPFL repair versus reconstruction. An MPFL repair to the patella has been reported in a large series and was noted to have a 72% success rate.23 Although a perceived benefit of the procedure is the absence of risk to the physis, concerns exist regarding the unacceptable rate of continued patellar instability after a repair of native tissue.
Several studies have demonstrated that reconstruction of the MPFL yields lower rates of recurrent dislocation, improved knee function, and an ability to return to prior levels of activity with little to no functional impairment.1,2,24-26 Studies have also shown that MPFL reconstruction can be performed safely in skeletally immature patients, with little risk of growth impairment, good to excellent results, and low re-rupture rates.27,28
We propose that MPFL reconstruction for the skeletally immature patient with PFI should be the primary surgical treatment. Any patient with atypical or severe bony dysplasia may warrant additional intervention. Additional correction of valgus, in the form of guided growth, should be considered in conjunction with an MPFL reconstruction if the patient demonstrates a valgus of grade >2 or a lateral distal femoral angle of <84°.
GRAFT OPTIONS
When an MPFL reconstruction is indicated, graft options include autologous hamstring, quadriceps, adductor, or patellar tendon grafts. Allograft tendon may be an acceptable choice, and use of synthetic graft has also been described. A recent systematic review29 concluded that there was no difference in recurrence rates or outcomes based on graft type. However, in this study, the overall complication rates were higher in patients who had autologous graft than in those who had allograft. Although the use of allograft has not been specifically reported in a pediatric cohort, allografts have been successfully used in this age group. Obviating graft harvest eliminates an additional or extended incision, limits postoperative weakness, and may speed early recovery.
Continue to: PREFERRED SURGICAL TECHNIQUE FOR MPFL RECONSTRUCTION WITH ALLOGRAFT...
PREFERRED SURGICAL TECHNIQUE FOR MPFL RECONSTRUCTION WITH ALLOGRAFT
Surgery should be scheduled and performed as an outpatient procedure. Preoperative instructions may include crutch training, when indicated, and postoperative education regarding expected early therapy and pain management strategy may be employed. Chlorhexidine wipes are provided to patients preoperatively with instruction to use daily starting 3 days prior to surgery. A home exercise program with a focus on quadriceps activation and range of motion is provided and requested to be performed postoperatively.
A standard operating room setup for knee arthroscopy is employed (preference list noted in Table 3; items bolded may be unique to an MPFL reconstruction). Regional, single-shot anesthesia is employed using a sensory-only adductor canal block. A universal surgical timeout is performed before beginning the surgery to include verification of prophylactic antibiotics (Ancef or clindamycin in penicillin-allergic patients) and consideration of the use of a sequential compression device in children aged >10 years when ≥1 venous thromboembolic risk factors are detected in preoperative screening.
Nonirradiated allografts (semitendinosus or tibialis anterior), preferably from a donor <30 years, are our preferred graft choice. The minimum length of the graft should be 240 mm and the doubled thickness should be 5 to 6 mm. After thawing, the graft is lavaged with a mixture of antibiotic saline consisting of 50,000 U of bacitracin in 1 l of normal saline. Tension is then placed on the graft using the graft preparation board, and a whip stitch is placed on each side of the graft using a #2 FiberWire (Arthrex). The graft is sized with care to ensure that it is not thicker than 6.5 mm with an optimal goal of 6 mm.
A standard knee arthroscopy is performed with an emphasis on evaluating the patellofemoral anatomy. Although insufflation can cause distortion and, often, lateralize the patella, the surgeon should consider an estimate of when the patella engages the trochlea during knee flexion. This position of knee flexion will determine the appropriate position of the knee during graft fixation. A bipolar device (90°Arthrocare wand) may be used to debride synovial folds (ie, plica) when affecting the patellar tracking. To maximize visualization of the patella within the knee, we recommend switching the arthroscope from the anterolateral portal to the anteromedial portal. This allows for improved visualization of the lateral femoral condyle and the inferomedial patellar facet, common locations for chondral and osteochondral damage, as well as optimal visualization of patellar engagement within the trochlea. During an arthroscopic dynamic exam of the patella tracking, a lateralized patella may be observed. If the patella tilts upon manual medial translation toward the trochlear groove during the dynamic exam, the lateral retinaculum may be tight or constraining the patella laterally. If this occurs, a partial or complete lateral release may be indicated with a bipolar wand. Visualization of the posteromedial and the posterolateral compartments is required as loose chondral bodies may be present in these locations.
Any osteochondral or chondral injuries are addressed during the arthroscopy. Large osteochondral fractures of the lateral femoral condyle or the patella are repaired using headless compression screws (Acutrak Screws, Acumed) or headed low-profile screws (1.5-mm screws, DePuy Synthes). Headless screws can be buried 2 to 3 mm below the chondral surface when the fragment has an appropriate thickness, whereas thin fragments may necessitate headed screws for adequate fixation and subsequent implant removal in 8 to 10 weeks. Defects in the patella most often require an open arthrotomy to evert the patella 90° to allow adequate exposure for treatment. Chronic chondral fragmentation may be debrided using the motorized chondrotome or removed and indicated for microfracture (<2 cm2 in surface area and <4 mm in depth) or other types of chondral replacement. Chondral lesions over the inferior medial facet are typically not symptomatic and often require minimal treatment.
Continue to: There are 3 methods to identify...
There are 3 methods to identify the insertion of the MPFL into the patella. During the diagnostic arthroscopy, an 18-gauge needle can be used to mark the insertion of the MPFL as visualization of the ligament arthroscopically is often possible (Figure 3). Another useful technique is to follow the inferior aspect of the distal insertion of the vastus medialis oblique (VMO) into the patella. The typical insertion point of the MPFL is immediately distal to the insertion of the inferior aspect of the VMO (Figure 4). It is also helpful to note that the center point of the insertion of the MPFL is at the junction of the proximal one-third and distal two-thirds of the palpable osseous patella. The MPFL origin has been noted to be at the exact midpoint of the chondral surface of the patella or 5 mm proximal (41% of the length of the patella) to the midpoint of the osseous patella.30
Following arthroscopic examination and treatment, a linear incision is made at the superior two-thirds of the patella, 1-finger breadth medial to the patella (Figure 5). During the subcutaneous dissection, the goal is to visualize the fascia overlying the VMO. Once this is identified, the dissection, in this layer, is carried over to expose the anterior and central surface of the patella. Army/navy retractors are used to retract the skin, and the assistant will place manual pressure on the patella to stabilize it for preparation of the patellar surface.
A bovie cautery or a knife is used to mark the insertion of the MPFL, which is immediately distal to the inferior border of the VMO. An incision over the medial surface of the patella creates a T-incision with elevation of the subsequent flaps proximal and distal. This allows exposure of the superficial and medial surface of the patella. During medial exposure of the patella, care is taken to avoid an arthrotomy by leaving the synovial lining attachment. A rongeur is used to decorticate the medial patella and the superficial surface (Figure 6). If an MPFL avulsion is present, it is often embedded within the soft tissues adjacent to the medial patellar. The MPFL avulsion can be exposed and removed if small. During an MPFL reconstruction, a repair of the avulsion is typically not performed. A double-loaded suture anchor is inserted at the site identified as the insertion of the native MPFL (3.0 Biocomposite SutureTak, Arthrex). A single suture anchor is used instead of an interosseous tunnel or double tunnels to avoid creating a large defect that may increase the risk of fracture in a small, skeletally immature patella.31
A hemostat is used to identify the layer between the medial retinaculum and the synovium over the medial soft tissue of the knee. The MPFL is a well-defined thickening of the medial retinaculum, and the ideal placement of the reconstruction is immediately inferior to this layer. Once this layer has been identified, a blunt hemostat is inserted to mark the end of a blind pouch that is apparent in this layer. This blind pouch is marked on the surface of the skin as the origin of the MPFL.
Fluoroscopy is now used to identify the origin of the MPFL on the medial femoral condyle. A perfect lateral is obtained by lining up the posterior condyle. Often, existent trochlear dysplasia will modify the normal appearance of the anterior structures of the condyles. Schottle’s point17 is USED to locate the origin of the MPFL. This is defined by drawing a line from the posterior cortex distally through the condyles (Figure 7). Two perpendicular lines are drawn, one to the extension of the posterior cortex at the level of the posterior extent of Blumensaat’s line and a second at the metaphyseal flare. Schottle’s point is located just anterior to the posterior cortex and in between these perpendicular lines. In a skeletally immature patient, this point appears to be at the level of the physis, on the lateral projection.
Continue to: An anteroposterior and/or a notch view projection...
An anteroposterior and/or a notch view projection is then performed to confirm the location of Schottle’s point, distal to the physis. Because the origin of the MPFL is posterior to the condyle, the notch view should be used to visualize Schottle’s point in relation to the physis in the coronal plane. This location has been confirmed to approximate the origin of the MPFL by published anatomic studies. If the graft measures 6 mm, an ideal distance of 5 to 8 mm distal to the physis will ensure a tunnel location with a proximal margin 2 to 4 mm distal to the physis. In our experience, Schottle’s point is approximately 5 to 7 mm distal to the physis, and rarely do we adjust our position. The guide wire is placed on the medial femoral condyle at this point and then angled 30° proximal to distal to allow tunnel trajectory distal to the undulating physis (Figure 8).
A 6-mm reamer is used over the guide wire to a depth of 20 mm. The newly created tunnel is now exposed and visualized. The graft is folded over at the center aspect and marked 20 mm from the tip of a Bio-tenodesis screwdriver (Arthrex). A 6.25 × 15 mm Bio-tenodesis screw is used. The graft and the screw are inserted into the condyle, and the appropriate graft fixation is confirmed by longitudinal tension placed upon the graft.
The hemostat or the passing suture previously placed into the blind pouch below the native MPFL is used to pass the graft immediately superficial to the synovial lining, in an extra-articular location. The ends of the graft are now exposed through the incision adjacent to the patella. The knee is confirmed to be flexed to approximately 45° on the surgical positioning triangleor at the flexion position determined during diagnostic arthroscopy at which the patella engages the trochlea. The graft is set to length alongside the medial surface of the patella at the position of the suture anchor, and a single stitch is placed through both ends of the graft at this adjacent position using a free needle (Davis Tonsil ½ Circle Taper Point; Vessel Medical). A hemostat is placed on the single suture throw to provisionally secure the graft in this trial position. This allows the surgeon to trial and examine the MPFL graft in extension, 45° of flexion, and terminal flexion. The goal is to provide a check rein to lateral translation of the patella and provide a firm endpoint to avoid further dislocation without overtension of the graft that may lead to increased contact pressures in the patellofemoral joint.
Once appropriate graft position is confirmed, using the double-loaded suture, we prefer to secure each limb separately to the decorticated medial patella. One end of the suture is threaded through the graft once and will act as a post. The other end of the suture is threaded through the graft 3 times in a modified Mason-Allen stitch. With the knee in that same position of flexion, the knot is tied and the graft is secured to the medial side of the patella. For secondary fixation, the remaining ends of the graft are passed below the periosteum on the anterior surface of the patella. Using a 0 Vicryl (Ethicon US), the periosteal flaps are sutured on top of the graft, incorporating the residual graft. The ends are cut and a repeat dynamic examination is performed to confirm the position of the patella and the patellar tracking and to ensure that overtensioning did not occur. Following irrigation, a standard closure is performed. The patient is placed in a hinged knee brace and cryotherapy is applied (Polar Care, Breg Inc).
POSTOPERATIVE PROTOCOL
For the initial or the acute postoperative phases, an emphasis on edema control, early quadriceps activation, and range of motion is recommended. We recommend weight-bearing as tolerated with the leg locked in extension until adequate quadriceps control is achieved. The patient must be able to perform 10 straight leg raises without an extension loss to be cleared to weight-bear as tolerated without motion restriction in the brace. Full motion is allowed immediately.
OUTCOMES
In our experience using isolated MPFL reconstruction in the skeletally immature patient, we have had no evidence of physeal arrest, leg-length inequality, or angular deformity, and only11.4% of patients have had recurrent instability. The mean Kujala score in this cohort was 90.4, with a mean Tegner activity of 7, after the procedure. All failures in our cohort had had severe trochlear dysplasia.
1. Chotel F, Berard J, Raux S. Patellar instability in children and adolescents. Orthop Traumatol Surg Res. 2014;100(1 Suppl.):S125-S137. doi: 10.1016/j.otsr.2013.06.014.
2. Gao B, Dwivedi S, Fabricant PD, Cruz AI Jr. Patterns in outcomes reporting of operatively managed pediatric patellofemoral instability: a systematic review and meta-analysis. Am J Sports Med. 2018;2:36354651876515. doi: 10.1177/0363546518765152.
3. Hennrikus W, Pylawka T. Patellofemoral instability in skeletally immature athletes. Instr Course Lect. 2013;62:445-453.
4. Vavken P, Wimmer MD, Camathias C, Quidde J, Valderrabano V, Pagenstert G. Treating patella instability in skeletally immature patients. Arthroscopy. 2013;29(8):1410-1422. doi: 10.1016/j.arthro.2013.03.075.
5. Askenberger M, Ekstrom W, Finnbogason T, Janarv PM. Occult intra-articular knee injuries in children With hemarthrosis. Am J Sports Med. 2014;42(7):1600-1606. doi: 10.1177/0363546514529639.
6. Seeley MA, Knesek M, Vanderhave KL. Osteochondral injury after acute patellar dislocation in children and adolescents. J Pediatr Orthop. 2013;33(5):511-518. doi: 10.1097/BPO.0b013e318288b7a0.
7. Cruz AI Jr, Milewski MD. Patellofemoral instability and other common knee issues in the skeletally immature knee (other knee pathology). In: Miller MD, ed. Orthopaedic Knowledge Update: Sports Medicine 5. 5th ed. Rosemont, IL: American Academy of Orthopaedic Surgeons; 2015.
8. Lewallen LW, McIntosh AL, Dahm DL. Predictors of recurrent instability after acute patellofemoral dislocation in pediatric and adolescent patients. Am J Sports Med. 2013;41(3):575-581. doi: 10.1177/0363546512472873.
9. Arshi A, Cohen JR, Wang JC, Hame SL, McAllister DR, Jones KJ. Operative management of patellar instability in the United States: an evaluation of national practice patterns, surgical trends, and complications. Orthop J Sports Med. 2016;4(8):2325967116662873. doi: 10.1177/2325967116662873.
10. Camathias C, Studer K, Kiapour A, Rutz E, Vavken P. Trochleoplasty as a solitary treatment for recurrent patellar dislocation results in good clinical outcome in adolescents. Am J Sports Med. 2016;44(11):2855-2863. doi: 10.1177/0363546516652894.
11. Jaquith BP, Parikh SN. Predictors of recurrent patellar iInstability in children and adolescents after first-time dislocation. J Pediatr Orthop. 2017;37(7):484-490. doi: 10.1097/BPO.0000000000000674.
12. Rong YH, Zhang GA, Wang C, Ning FG. [Quantification of type I and III collagen content in normal human skin in different age groups]. Zhonghua Shao Shang Za Zhi. 2008;24(1):51-53.
13. Wilson PL, Black SR, Ellis HB, Podeszwa DA. Distal femoral valgus and recurrent traumatic patellar instability: is an isolated varus producing distal femoral osteotomy a treatment option? J Pediatr Orthop. 2018;38(3):e162-e167. doi: 10.1097/BPO.0000000000001128.
14. Kearney SP, Mosca VS. Selective hemiepiphyseodesis for patellar instability with associated genu valgum. J Orthop. 2015;12(1):17-22. doi: 10.1016/j.jor.2015.01.005.
15. Kraus T, Lidder S, Svehlik M, Rippel K, Schneider F, Eberl R, Linhart W. Patella re-alignment in children with a modified Grammont technique. Acta Orthop. 2012;83(5):504-510. doi: 10.3109/17453674.2012.736168.
16. Shea KG, Grimm NL, Belzer J, Burks RT, Pfeiffer R. The relation of the femoral physis and the medial patellofemoral ligament. Arthroscopy. 2010;26(8):1083-1087. doi: 10.1016/j.arthro.2009.12.020.
17. Schottle PB, Schmeling A, Rosenstiel N, Weiler A. Radiographic landmarks for femoral tunnel placement in medial patellofemoral ligament reconstruction. Am J Sports Med. 2007;35(5):801-804. doi: 10.1177/0363546506296415.
18. Kepler CK, Bogner EA, Hammoud S, Malcolmson G, Potter HG, Green DW. Zone of injury of the medial patellofemoral ligament after acute patellar dislocation in children and adolescents. Am J Sports Med. 2011;39(7):1444-1449. doi: 10.1177/0363546510397174.
19. Nelitz M, Dornacher D, Dreyhaupt J, Reichel H, Lippacher S. The relation of the distal femoral physis and the medial patellofemoral ligament. Knee Surg Sports Traumatol Arthrosc. 2011;19(12):2067-2071. doi: 10.1007/s00167-011-1548-3.
20. Shea KG, Styhl AC, Jacobs JC Jr, Ganley TJ, Milewski MD, Cannamela PC, et al. The relationship of the femoral physis and the medial patellofemoral ligament in children: A Cadaveric Study. Am J Sports Med. 2016;44(11):2833-2837. doi: 10.1177/0363546516656366.
21. Popkin CA, Bayomy AF, Trupia EP, Chan CM, Redler LH. Patellar instability in the skeletally immature. Curr Rev Musculoskelet Med. 2018;11(2):172-181. doi: 10.1007/s12178-018-9472-5.
22. Keyes S, Price M, Green DW, Parikh SN. Special considerations for pediatric patellar instability. Am J Orthop Belle Mead NJ. 2018;47(3). doi: 10.12788/ajo.2018.0017.
23. Camp CL, Krych AJ, Dahm DL, Levy BA, Stuart MJ. Medial patellofemoral ligament repair for recurrent patellar dislocation. Am J Sports Med. 2010;38(11):2248-2254. doi: 10.1177/0363546510376230.
24. Fabricant PD, Ladenhauf HN, Salvati EA, Green DW, Green DW. Medial patellofemoral ligament (MPFL) reconstruction improves radiographic measures of Patella alta in children. Knee. 2014;21(6):1180-1184. doi: 10.1016/j.knee.2014.07.023.
25. Matic GT, Magnussen RA, Kolovich GP, Flanigan DC. Return to activity after medial patellofemoral ligament repair or reconstruction. Arthroscopy. 2014;30(8):1018-1025. doi: 10.1016/j.arthro.2014.02.044.
26. Mostrom EB, Mikkelsen C, Weidenhielm L, Janarv PM. Long-term follow-up of nonoperatively and operatively treated acute primary patellar dislocation in skeletally immature patients. Sciworldj. 2014;2014:473281. doi: 10.1155/2014/473281.
27. Antinolfi P, Bartoli M, Placella G, Speziali A, Pace V, Delcogliano M, Mazzola C. Acute patellofemoral instability in children and adolescents. Joints. 2016;4(1):47-51. doi: 10.11138/jts/2016.4.1.047.
28. Nelitz M, Dreyhaupt J, Reichel H, Woelfle J, Lippacher S. Anatomic reconstruction of the medial patellofemoral ligament in children and adolescents with open growth plates: surgical technique and clinical outcome. Am J Sports Med. 2013;41(1):58-63. doi: 10.1177/0363546512463683.
29. McNeilan RJ, Everhart JS, Mescher PK, Abouljoud M, Magnussen RA, Flanigan DC. Graft choice in isolated medial patellofemoral ligament reconstruction: A systematic review with meta-analysis of rates of recurrent instability and patient-reported outcomes for autograft, allograft, and synthetic options. Arthroscopy. 2018;34(4):1340-1354. doi: 10.1016/j.arthro.2017.11.027.
30. Shea KG, Polousky JD, Jacobs JC Jr, Ganley TJ, Aoki SK, Grimm NL, Parikh SN. The patellar insertion of the medial patellofemoral ligament in children: a cadaveric study. J Pediatr Orthop. 2015;35(4):e31-e35. doi: 10.1097/BPO.0000000000000399.
31. Parikh SN, Wall EJ. Patellar fracture after medial patellofemoral ligament surgery: a report of five cases. J Bone Joint Surg, (Am.). 2011;93(17):e97(1-8). doi: 10.2106/JBJS.J.01558.
ABSTRACT
Patellofemoral instability commonly occurs in the young patient, and, often, skeletal immaturity may be a risk factor for possible recurrence. Treatment considerations, including operative and nonoperative management, are based on anatomic factors. A medial patellofemoral ligament (MPFL) reconstruction is a treatment option for a skeletally immature patient with recurrent instability or for patients with a high risk of patellofemoral instability recurrence. A physeal-sparing MPFL reconstruction technique that considers the origin of the MPFL to be distal to the distal femoral physis may be employed.
Continue to: Patellofemoral instability (PFI)...
Patellofemoral instability (PFI), 1 of the most common patellofemoral disorders observed in skeletally immature patients,1-4 has a reported incidence of 43 per 100,000 skeletally immature patients.3 The incidence is even higher in patients 9 to 15 years, with dislocations occurring in 107 per 100,000 individuals.5,6 In recent years, there has been an increasing interest in studying PFI in skeletally immature pediatric patients, who are particularly susceptible to recurrent dislocations. Studies have indicated that children <16 years are at the highest risk for recurrence.7 Lewallen and colleagues8 noted a 69% failure rate and a 3-fold increase in the probability of recurrent dislocation in skeletally immature patients treated nonoperatively.
Anatomic factors that contribute to PFI include ligament laxity, trochlear dysplasia, patella alta, excessive femoral anteversion or tibial torsion, genu valgum, and increased tibial tubercle-to-trochlear groove distance.1,3,8-11 When considering surgical treatment for PFI, all anatomic factors should be considered, with an emphasis on, and understanding of, the role of residual growth and development. One must also consider balancing static soft tissue, dynamic soft tissue, and osseous constraints of the patellofemoral joint to optimize the overall health and balance of the patella (Figure 1).
CHALLENGES IN TREATING PEDIATRIC PFI
A primary challenge in the treatment of PFI in pediatric patients is accounting for the impact of anatomic changes occurring secondary to growth and maturity. From birth to adulthood, the collagen composition of soft tissue changes from an elastic type III collagen to a stiffer type I collagen.12 These physiologic changes may influence the rigidity of the soft tissue restraints around the patellofemoral joint during periods of rapid growth. Longitudinal growth and rotational changes can also occur at the distal femoral and proximal tibial physes. The position of the tibial tubercle and the magnitude of femoral anteversion may also change after growth in adolescents.
Developing effective technical analogs of surgical procedures performed in mature patients with PFI for use in skeletally immature patients has been a second challenge. For example, a varus-producing distal femoral osteotomy to address genu valgum and PFI13 is contraindicated in immature patients, when a hemiepiphysiodesis for guided growth should be considered.14 Similarly, a periosteal sleeve medialization of the insertion of the patellar tendon may be used instead of a tibial tubercle transfer.15
If a medial patellofemoral ligament (MPFL) reconstruction is considered in immature patients, careful consideration of the position of the MPFL in relation to the distal femoral physis is paramount. Shea and colleagues16 originally described the position of the MPFL, based on Schottle’s point17 on lateral radiographs, to be proximal to the distal femoral physis (Figure 2). However, due to the undulation of the physis, the lateral projection may falsely demonstrate Schottle’s point to be on or just proximal to the physis. Other researchers have evaluated the position of the distal femoral physis to be proximal to the origin of the MPFL by a range of 2.9 mm to 8.5 mm on AP radiograph and MRI, respectively.18,19 More recent cadaveric studies have demonstrated the origin of the MPFL in pediatric specimens in relation to the physis to be variable.20 Although we believe that a relative anatomic femoral position of the MPFL origin can be achieved without disrupting the physis using Schottle’s point, some have indicated concerns that this may produce a nonisometric position, which has not been our experience.21
Continue to: TREATMENT OPTIONS...
TREATMENT OPTIONS
The evaluation of a skeletally immature patient may differ from that of an adult patient. (Table 1) represents a standard evaluation of a skeletally immature patient with PFI. The injury, physical examination findings, activity level, presence of an osteochondral fracture, and severity of bony dysplasia may indicate surgical treatment and influence selection of the surgical technique. Indications for surgical treatment include recurrent patellar instability, symptomatic patellar instability, the presence of a chondral or an osteochondral fracture, and a primary patella dislocation in patients with a high risk for recurrent dislocations. Table 2 represents a list of the possible surgical treatment options for the skeletally immature patient with PFI.21,22 Variable outcomes and recurrence rates are noted with each procedure.21,22
MPFL REPAIR VS RECONSTRUCTION
Although there is increased consensus for restoring normal anatomy, continued controversy exists regarding the utility of an MPFL repair versus reconstruction. An MPFL repair to the patella has been reported in a large series and was noted to have a 72% success rate.23 Although a perceived benefit of the procedure is the absence of risk to the physis, concerns exist regarding the unacceptable rate of continued patellar instability after a repair of native tissue.
Several studies have demonstrated that reconstruction of the MPFL yields lower rates of recurrent dislocation, improved knee function, and an ability to return to prior levels of activity with little to no functional impairment.1,2,24-26 Studies have also shown that MPFL reconstruction can be performed safely in skeletally immature patients, with little risk of growth impairment, good to excellent results, and low re-rupture rates.27,28
We propose that MPFL reconstruction for the skeletally immature patient with PFI should be the primary surgical treatment. Any patient with atypical or severe bony dysplasia may warrant additional intervention. Additional correction of valgus, in the form of guided growth, should be considered in conjunction with an MPFL reconstruction if the patient demonstrates a valgus of grade >2 or a lateral distal femoral angle of <84°.
GRAFT OPTIONS
When an MPFL reconstruction is indicated, graft options include autologous hamstring, quadriceps, adductor, or patellar tendon grafts. Allograft tendon may be an acceptable choice, and use of synthetic graft has also been described. A recent systematic review29 concluded that there was no difference in recurrence rates or outcomes based on graft type. However, in this study, the overall complication rates were higher in patients who had autologous graft than in those who had allograft. Although the use of allograft has not been specifically reported in a pediatric cohort, allografts have been successfully used in this age group. Obviating graft harvest eliminates an additional or extended incision, limits postoperative weakness, and may speed early recovery.
Continue to: PREFERRED SURGICAL TECHNIQUE FOR MPFL RECONSTRUCTION WITH ALLOGRAFT...
PREFERRED SURGICAL TECHNIQUE FOR MPFL RECONSTRUCTION WITH ALLOGRAFT
Surgery should be scheduled and performed as an outpatient procedure. Preoperative instructions may include crutch training, when indicated, and postoperative education regarding expected early therapy and pain management strategy may be employed. Chlorhexidine wipes are provided to patients preoperatively with instruction to use daily starting 3 days prior to surgery. A home exercise program with a focus on quadriceps activation and range of motion is provided and requested to be performed postoperatively.
A standard operating room setup for knee arthroscopy is employed (preference list noted in Table 3; items bolded may be unique to an MPFL reconstruction). Regional, single-shot anesthesia is employed using a sensory-only adductor canal block. A universal surgical timeout is performed before beginning the surgery to include verification of prophylactic antibiotics (Ancef or clindamycin in penicillin-allergic patients) and consideration of the use of a sequential compression device in children aged >10 years when ≥1 venous thromboembolic risk factors are detected in preoperative screening.
Nonirradiated allografts (semitendinosus or tibialis anterior), preferably from a donor <30 years, are our preferred graft choice. The minimum length of the graft should be 240 mm and the doubled thickness should be 5 to 6 mm. After thawing, the graft is lavaged with a mixture of antibiotic saline consisting of 50,000 U of bacitracin in 1 l of normal saline. Tension is then placed on the graft using the graft preparation board, and a whip stitch is placed on each side of the graft using a #2 FiberWire (Arthrex). The graft is sized with care to ensure that it is not thicker than 6.5 mm with an optimal goal of 6 mm.
A standard knee arthroscopy is performed with an emphasis on evaluating the patellofemoral anatomy. Although insufflation can cause distortion and, often, lateralize the patella, the surgeon should consider an estimate of when the patella engages the trochlea during knee flexion. This position of knee flexion will determine the appropriate position of the knee during graft fixation. A bipolar device (90°Arthrocare wand) may be used to debride synovial folds (ie, plica) when affecting the patellar tracking. To maximize visualization of the patella within the knee, we recommend switching the arthroscope from the anterolateral portal to the anteromedial portal. This allows for improved visualization of the lateral femoral condyle and the inferomedial patellar facet, common locations for chondral and osteochondral damage, as well as optimal visualization of patellar engagement within the trochlea. During an arthroscopic dynamic exam of the patella tracking, a lateralized patella may be observed. If the patella tilts upon manual medial translation toward the trochlear groove during the dynamic exam, the lateral retinaculum may be tight or constraining the patella laterally. If this occurs, a partial or complete lateral release may be indicated with a bipolar wand. Visualization of the posteromedial and the posterolateral compartments is required as loose chondral bodies may be present in these locations.
Any osteochondral or chondral injuries are addressed during the arthroscopy. Large osteochondral fractures of the lateral femoral condyle or the patella are repaired using headless compression screws (Acutrak Screws, Acumed) or headed low-profile screws (1.5-mm screws, DePuy Synthes). Headless screws can be buried 2 to 3 mm below the chondral surface when the fragment has an appropriate thickness, whereas thin fragments may necessitate headed screws for adequate fixation and subsequent implant removal in 8 to 10 weeks. Defects in the patella most often require an open arthrotomy to evert the patella 90° to allow adequate exposure for treatment. Chronic chondral fragmentation may be debrided using the motorized chondrotome or removed and indicated for microfracture (<2 cm2 in surface area and <4 mm in depth) or other types of chondral replacement. Chondral lesions over the inferior medial facet are typically not symptomatic and often require minimal treatment.
Continue to: There are 3 methods to identify...
There are 3 methods to identify the insertion of the MPFL into the patella. During the diagnostic arthroscopy, an 18-gauge needle can be used to mark the insertion of the MPFL as visualization of the ligament arthroscopically is often possible (Figure 3). Another useful technique is to follow the inferior aspect of the distal insertion of the vastus medialis oblique (VMO) into the patella. The typical insertion point of the MPFL is immediately distal to the insertion of the inferior aspect of the VMO (Figure 4). It is also helpful to note that the center point of the insertion of the MPFL is at the junction of the proximal one-third and distal two-thirds of the palpable osseous patella. The MPFL origin has been noted to be at the exact midpoint of the chondral surface of the patella or 5 mm proximal (41% of the length of the patella) to the midpoint of the osseous patella.30
Following arthroscopic examination and treatment, a linear incision is made at the superior two-thirds of the patella, 1-finger breadth medial to the patella (Figure 5). During the subcutaneous dissection, the goal is to visualize the fascia overlying the VMO. Once this is identified, the dissection, in this layer, is carried over to expose the anterior and central surface of the patella. Army/navy retractors are used to retract the skin, and the assistant will place manual pressure on the patella to stabilize it for preparation of the patellar surface.
A bovie cautery or a knife is used to mark the insertion of the MPFL, which is immediately distal to the inferior border of the VMO. An incision over the medial surface of the patella creates a T-incision with elevation of the subsequent flaps proximal and distal. This allows exposure of the superficial and medial surface of the patella. During medial exposure of the patella, care is taken to avoid an arthrotomy by leaving the synovial lining attachment. A rongeur is used to decorticate the medial patella and the superficial surface (Figure 6). If an MPFL avulsion is present, it is often embedded within the soft tissues adjacent to the medial patellar. The MPFL avulsion can be exposed and removed if small. During an MPFL reconstruction, a repair of the avulsion is typically not performed. A double-loaded suture anchor is inserted at the site identified as the insertion of the native MPFL (3.0 Biocomposite SutureTak, Arthrex). A single suture anchor is used instead of an interosseous tunnel or double tunnels to avoid creating a large defect that may increase the risk of fracture in a small, skeletally immature patella.31
A hemostat is used to identify the layer between the medial retinaculum and the synovium over the medial soft tissue of the knee. The MPFL is a well-defined thickening of the medial retinaculum, and the ideal placement of the reconstruction is immediately inferior to this layer. Once this layer has been identified, a blunt hemostat is inserted to mark the end of a blind pouch that is apparent in this layer. This blind pouch is marked on the surface of the skin as the origin of the MPFL.
Fluoroscopy is now used to identify the origin of the MPFL on the medial femoral condyle. A perfect lateral is obtained by lining up the posterior condyle. Often, existent trochlear dysplasia will modify the normal appearance of the anterior structures of the condyles. Schottle’s point17 is USED to locate the origin of the MPFL. This is defined by drawing a line from the posterior cortex distally through the condyles (Figure 7). Two perpendicular lines are drawn, one to the extension of the posterior cortex at the level of the posterior extent of Blumensaat’s line and a second at the metaphyseal flare. Schottle’s point is located just anterior to the posterior cortex and in between these perpendicular lines. In a skeletally immature patient, this point appears to be at the level of the physis, on the lateral projection.
Continue to: An anteroposterior and/or a notch view projection...
An anteroposterior and/or a notch view projection is then performed to confirm the location of Schottle’s point, distal to the physis. Because the origin of the MPFL is posterior to the condyle, the notch view should be used to visualize Schottle’s point in relation to the physis in the coronal plane. This location has been confirmed to approximate the origin of the MPFL by published anatomic studies. If the graft measures 6 mm, an ideal distance of 5 to 8 mm distal to the physis will ensure a tunnel location with a proximal margin 2 to 4 mm distal to the physis. In our experience, Schottle’s point is approximately 5 to 7 mm distal to the physis, and rarely do we adjust our position. The guide wire is placed on the medial femoral condyle at this point and then angled 30° proximal to distal to allow tunnel trajectory distal to the undulating physis (Figure 8).
A 6-mm reamer is used over the guide wire to a depth of 20 mm. The newly created tunnel is now exposed and visualized. The graft is folded over at the center aspect and marked 20 mm from the tip of a Bio-tenodesis screwdriver (Arthrex). A 6.25 × 15 mm Bio-tenodesis screw is used. The graft and the screw are inserted into the condyle, and the appropriate graft fixation is confirmed by longitudinal tension placed upon the graft.
The hemostat or the passing suture previously placed into the blind pouch below the native MPFL is used to pass the graft immediately superficial to the synovial lining, in an extra-articular location. The ends of the graft are now exposed through the incision adjacent to the patella. The knee is confirmed to be flexed to approximately 45° on the surgical positioning triangleor at the flexion position determined during diagnostic arthroscopy at which the patella engages the trochlea. The graft is set to length alongside the medial surface of the patella at the position of the suture anchor, and a single stitch is placed through both ends of the graft at this adjacent position using a free needle (Davis Tonsil ½ Circle Taper Point; Vessel Medical). A hemostat is placed on the single suture throw to provisionally secure the graft in this trial position. This allows the surgeon to trial and examine the MPFL graft in extension, 45° of flexion, and terminal flexion. The goal is to provide a check rein to lateral translation of the patella and provide a firm endpoint to avoid further dislocation without overtension of the graft that may lead to increased contact pressures in the patellofemoral joint.
Once appropriate graft position is confirmed, using the double-loaded suture, we prefer to secure each limb separately to the decorticated medial patella. One end of the suture is threaded through the graft once and will act as a post. The other end of the suture is threaded through the graft 3 times in a modified Mason-Allen stitch. With the knee in that same position of flexion, the knot is tied and the graft is secured to the medial side of the patella. For secondary fixation, the remaining ends of the graft are passed below the periosteum on the anterior surface of the patella. Using a 0 Vicryl (Ethicon US), the periosteal flaps are sutured on top of the graft, incorporating the residual graft. The ends are cut and a repeat dynamic examination is performed to confirm the position of the patella and the patellar tracking and to ensure that overtensioning did not occur. Following irrigation, a standard closure is performed. The patient is placed in a hinged knee brace and cryotherapy is applied (Polar Care, Breg Inc).
POSTOPERATIVE PROTOCOL
For the initial or the acute postoperative phases, an emphasis on edema control, early quadriceps activation, and range of motion is recommended. We recommend weight-bearing as tolerated with the leg locked in extension until adequate quadriceps control is achieved. The patient must be able to perform 10 straight leg raises without an extension loss to be cleared to weight-bear as tolerated without motion restriction in the brace. Full motion is allowed immediately.
OUTCOMES
In our experience using isolated MPFL reconstruction in the skeletally immature patient, we have had no evidence of physeal arrest, leg-length inequality, or angular deformity, and only11.4% of patients have had recurrent instability. The mean Kujala score in this cohort was 90.4, with a mean Tegner activity of 7, after the procedure. All failures in our cohort had had severe trochlear dysplasia.
ABSTRACT
Patellofemoral instability commonly occurs in the young patient, and, often, skeletal immaturity may be a risk factor for possible recurrence. Treatment considerations, including operative and nonoperative management, are based on anatomic factors. A medial patellofemoral ligament (MPFL) reconstruction is a treatment option for a skeletally immature patient with recurrent instability or for patients with a high risk of patellofemoral instability recurrence. A physeal-sparing MPFL reconstruction technique that considers the origin of the MPFL to be distal to the distal femoral physis may be employed.
Continue to: Patellofemoral instability (PFI)...
Patellofemoral instability (PFI), 1 of the most common patellofemoral disorders observed in skeletally immature patients,1-4 has a reported incidence of 43 per 100,000 skeletally immature patients.3 The incidence is even higher in patients 9 to 15 years, with dislocations occurring in 107 per 100,000 individuals.5,6 In recent years, there has been an increasing interest in studying PFI in skeletally immature pediatric patients, who are particularly susceptible to recurrent dislocations. Studies have indicated that children <16 years are at the highest risk for recurrence.7 Lewallen and colleagues8 noted a 69% failure rate and a 3-fold increase in the probability of recurrent dislocation in skeletally immature patients treated nonoperatively.
Anatomic factors that contribute to PFI include ligament laxity, trochlear dysplasia, patella alta, excessive femoral anteversion or tibial torsion, genu valgum, and increased tibial tubercle-to-trochlear groove distance.1,3,8-11 When considering surgical treatment for PFI, all anatomic factors should be considered, with an emphasis on, and understanding of, the role of residual growth and development. One must also consider balancing static soft tissue, dynamic soft tissue, and osseous constraints of the patellofemoral joint to optimize the overall health and balance of the patella (Figure 1).
CHALLENGES IN TREATING PEDIATRIC PFI
A primary challenge in the treatment of PFI in pediatric patients is accounting for the impact of anatomic changes occurring secondary to growth and maturity. From birth to adulthood, the collagen composition of soft tissue changes from an elastic type III collagen to a stiffer type I collagen.12 These physiologic changes may influence the rigidity of the soft tissue restraints around the patellofemoral joint during periods of rapid growth. Longitudinal growth and rotational changes can also occur at the distal femoral and proximal tibial physes. The position of the tibial tubercle and the magnitude of femoral anteversion may also change after growth in adolescents.
Developing effective technical analogs of surgical procedures performed in mature patients with PFI for use in skeletally immature patients has been a second challenge. For example, a varus-producing distal femoral osteotomy to address genu valgum and PFI13 is contraindicated in immature patients, when a hemiepiphysiodesis for guided growth should be considered.14 Similarly, a periosteal sleeve medialization of the insertion of the patellar tendon may be used instead of a tibial tubercle transfer.15
If a medial patellofemoral ligament (MPFL) reconstruction is considered in immature patients, careful consideration of the position of the MPFL in relation to the distal femoral physis is paramount. Shea and colleagues16 originally described the position of the MPFL, based on Schottle’s point17 on lateral radiographs, to be proximal to the distal femoral physis (Figure 2). However, due to the undulation of the physis, the lateral projection may falsely demonstrate Schottle’s point to be on or just proximal to the physis. Other researchers have evaluated the position of the distal femoral physis to be proximal to the origin of the MPFL by a range of 2.9 mm to 8.5 mm on AP radiograph and MRI, respectively.18,19 More recent cadaveric studies have demonstrated the origin of the MPFL in pediatric specimens in relation to the physis to be variable.20 Although we believe that a relative anatomic femoral position of the MPFL origin can be achieved without disrupting the physis using Schottle’s point, some have indicated concerns that this may produce a nonisometric position, which has not been our experience.21
Continue to: TREATMENT OPTIONS...
TREATMENT OPTIONS
The evaluation of a skeletally immature patient may differ from that of an adult patient. (Table 1) represents a standard evaluation of a skeletally immature patient with PFI. The injury, physical examination findings, activity level, presence of an osteochondral fracture, and severity of bony dysplasia may indicate surgical treatment and influence selection of the surgical technique. Indications for surgical treatment include recurrent patellar instability, symptomatic patellar instability, the presence of a chondral or an osteochondral fracture, and a primary patella dislocation in patients with a high risk for recurrent dislocations. Table 2 represents a list of the possible surgical treatment options for the skeletally immature patient with PFI.21,22 Variable outcomes and recurrence rates are noted with each procedure.21,22
MPFL REPAIR VS RECONSTRUCTION
Although there is increased consensus for restoring normal anatomy, continued controversy exists regarding the utility of an MPFL repair versus reconstruction. An MPFL repair to the patella has been reported in a large series and was noted to have a 72% success rate.23 Although a perceived benefit of the procedure is the absence of risk to the physis, concerns exist regarding the unacceptable rate of continued patellar instability after a repair of native tissue.
Several studies have demonstrated that reconstruction of the MPFL yields lower rates of recurrent dislocation, improved knee function, and an ability to return to prior levels of activity with little to no functional impairment.1,2,24-26 Studies have also shown that MPFL reconstruction can be performed safely in skeletally immature patients, with little risk of growth impairment, good to excellent results, and low re-rupture rates.27,28
We propose that MPFL reconstruction for the skeletally immature patient with PFI should be the primary surgical treatment. Any patient with atypical or severe bony dysplasia may warrant additional intervention. Additional correction of valgus, in the form of guided growth, should be considered in conjunction with an MPFL reconstruction if the patient demonstrates a valgus of grade >2 or a lateral distal femoral angle of <84°.
GRAFT OPTIONS
When an MPFL reconstruction is indicated, graft options include autologous hamstring, quadriceps, adductor, or patellar tendon grafts. Allograft tendon may be an acceptable choice, and use of synthetic graft has also been described. A recent systematic review29 concluded that there was no difference in recurrence rates or outcomes based on graft type. However, in this study, the overall complication rates were higher in patients who had autologous graft than in those who had allograft. Although the use of allograft has not been specifically reported in a pediatric cohort, allografts have been successfully used in this age group. Obviating graft harvest eliminates an additional or extended incision, limits postoperative weakness, and may speed early recovery.
Continue to: PREFERRED SURGICAL TECHNIQUE FOR MPFL RECONSTRUCTION WITH ALLOGRAFT...
PREFERRED SURGICAL TECHNIQUE FOR MPFL RECONSTRUCTION WITH ALLOGRAFT
Surgery should be scheduled and performed as an outpatient procedure. Preoperative instructions may include crutch training, when indicated, and postoperative education regarding expected early therapy and pain management strategy may be employed. Chlorhexidine wipes are provided to patients preoperatively with instruction to use daily starting 3 days prior to surgery. A home exercise program with a focus on quadriceps activation and range of motion is provided and requested to be performed postoperatively.
A standard operating room setup for knee arthroscopy is employed (preference list noted in Table 3; items bolded may be unique to an MPFL reconstruction). Regional, single-shot anesthesia is employed using a sensory-only adductor canal block. A universal surgical timeout is performed before beginning the surgery to include verification of prophylactic antibiotics (Ancef or clindamycin in penicillin-allergic patients) and consideration of the use of a sequential compression device in children aged >10 years when ≥1 venous thromboembolic risk factors are detected in preoperative screening.
Nonirradiated allografts (semitendinosus or tibialis anterior), preferably from a donor <30 years, are our preferred graft choice. The minimum length of the graft should be 240 mm and the doubled thickness should be 5 to 6 mm. After thawing, the graft is lavaged with a mixture of antibiotic saline consisting of 50,000 U of bacitracin in 1 l of normal saline. Tension is then placed on the graft using the graft preparation board, and a whip stitch is placed on each side of the graft using a #2 FiberWire (Arthrex). The graft is sized with care to ensure that it is not thicker than 6.5 mm with an optimal goal of 6 mm.
A standard knee arthroscopy is performed with an emphasis on evaluating the patellofemoral anatomy. Although insufflation can cause distortion and, often, lateralize the patella, the surgeon should consider an estimate of when the patella engages the trochlea during knee flexion. This position of knee flexion will determine the appropriate position of the knee during graft fixation. A bipolar device (90°Arthrocare wand) may be used to debride synovial folds (ie, plica) when affecting the patellar tracking. To maximize visualization of the patella within the knee, we recommend switching the arthroscope from the anterolateral portal to the anteromedial portal. This allows for improved visualization of the lateral femoral condyle and the inferomedial patellar facet, common locations for chondral and osteochondral damage, as well as optimal visualization of patellar engagement within the trochlea. During an arthroscopic dynamic exam of the patella tracking, a lateralized patella may be observed. If the patella tilts upon manual medial translation toward the trochlear groove during the dynamic exam, the lateral retinaculum may be tight or constraining the patella laterally. If this occurs, a partial or complete lateral release may be indicated with a bipolar wand. Visualization of the posteromedial and the posterolateral compartments is required as loose chondral bodies may be present in these locations.
Any osteochondral or chondral injuries are addressed during the arthroscopy. Large osteochondral fractures of the lateral femoral condyle or the patella are repaired using headless compression screws (Acutrak Screws, Acumed) or headed low-profile screws (1.5-mm screws, DePuy Synthes). Headless screws can be buried 2 to 3 mm below the chondral surface when the fragment has an appropriate thickness, whereas thin fragments may necessitate headed screws for adequate fixation and subsequent implant removal in 8 to 10 weeks. Defects in the patella most often require an open arthrotomy to evert the patella 90° to allow adequate exposure for treatment. Chronic chondral fragmentation may be debrided using the motorized chondrotome or removed and indicated for microfracture (<2 cm2 in surface area and <4 mm in depth) or other types of chondral replacement. Chondral lesions over the inferior medial facet are typically not symptomatic and often require minimal treatment.
Continue to: There are 3 methods to identify...
There are 3 methods to identify the insertion of the MPFL into the patella. During the diagnostic arthroscopy, an 18-gauge needle can be used to mark the insertion of the MPFL as visualization of the ligament arthroscopically is often possible (Figure 3). Another useful technique is to follow the inferior aspect of the distal insertion of the vastus medialis oblique (VMO) into the patella. The typical insertion point of the MPFL is immediately distal to the insertion of the inferior aspect of the VMO (Figure 4). It is also helpful to note that the center point of the insertion of the MPFL is at the junction of the proximal one-third and distal two-thirds of the palpable osseous patella. The MPFL origin has been noted to be at the exact midpoint of the chondral surface of the patella or 5 mm proximal (41% of the length of the patella) to the midpoint of the osseous patella.30
Following arthroscopic examination and treatment, a linear incision is made at the superior two-thirds of the patella, 1-finger breadth medial to the patella (Figure 5). During the subcutaneous dissection, the goal is to visualize the fascia overlying the VMO. Once this is identified, the dissection, in this layer, is carried over to expose the anterior and central surface of the patella. Army/navy retractors are used to retract the skin, and the assistant will place manual pressure on the patella to stabilize it for preparation of the patellar surface.
A bovie cautery or a knife is used to mark the insertion of the MPFL, which is immediately distal to the inferior border of the VMO. An incision over the medial surface of the patella creates a T-incision with elevation of the subsequent flaps proximal and distal. This allows exposure of the superficial and medial surface of the patella. During medial exposure of the patella, care is taken to avoid an arthrotomy by leaving the synovial lining attachment. A rongeur is used to decorticate the medial patella and the superficial surface (Figure 6). If an MPFL avulsion is present, it is often embedded within the soft tissues adjacent to the medial patellar. The MPFL avulsion can be exposed and removed if small. During an MPFL reconstruction, a repair of the avulsion is typically not performed. A double-loaded suture anchor is inserted at the site identified as the insertion of the native MPFL (3.0 Biocomposite SutureTak, Arthrex). A single suture anchor is used instead of an interosseous tunnel or double tunnels to avoid creating a large defect that may increase the risk of fracture in a small, skeletally immature patella.31
A hemostat is used to identify the layer between the medial retinaculum and the synovium over the medial soft tissue of the knee. The MPFL is a well-defined thickening of the medial retinaculum, and the ideal placement of the reconstruction is immediately inferior to this layer. Once this layer has been identified, a blunt hemostat is inserted to mark the end of a blind pouch that is apparent in this layer. This blind pouch is marked on the surface of the skin as the origin of the MPFL.
Fluoroscopy is now used to identify the origin of the MPFL on the medial femoral condyle. A perfect lateral is obtained by lining up the posterior condyle. Often, existent trochlear dysplasia will modify the normal appearance of the anterior structures of the condyles. Schottle’s point17 is USED to locate the origin of the MPFL. This is defined by drawing a line from the posterior cortex distally through the condyles (Figure 7). Two perpendicular lines are drawn, one to the extension of the posterior cortex at the level of the posterior extent of Blumensaat’s line and a second at the metaphyseal flare. Schottle’s point is located just anterior to the posterior cortex and in between these perpendicular lines. In a skeletally immature patient, this point appears to be at the level of the physis, on the lateral projection.
Continue to: An anteroposterior and/or a notch view projection...
An anteroposterior and/or a notch view projection is then performed to confirm the location of Schottle’s point, distal to the physis. Because the origin of the MPFL is posterior to the condyle, the notch view should be used to visualize Schottle’s point in relation to the physis in the coronal plane. This location has been confirmed to approximate the origin of the MPFL by published anatomic studies. If the graft measures 6 mm, an ideal distance of 5 to 8 mm distal to the physis will ensure a tunnel location with a proximal margin 2 to 4 mm distal to the physis. In our experience, Schottle’s point is approximately 5 to 7 mm distal to the physis, and rarely do we adjust our position. The guide wire is placed on the medial femoral condyle at this point and then angled 30° proximal to distal to allow tunnel trajectory distal to the undulating physis (Figure 8).
A 6-mm reamer is used over the guide wire to a depth of 20 mm. The newly created tunnel is now exposed and visualized. The graft is folded over at the center aspect and marked 20 mm from the tip of a Bio-tenodesis screwdriver (Arthrex). A 6.25 × 15 mm Bio-tenodesis screw is used. The graft and the screw are inserted into the condyle, and the appropriate graft fixation is confirmed by longitudinal tension placed upon the graft.
The hemostat or the passing suture previously placed into the blind pouch below the native MPFL is used to pass the graft immediately superficial to the synovial lining, in an extra-articular location. The ends of the graft are now exposed through the incision adjacent to the patella. The knee is confirmed to be flexed to approximately 45° on the surgical positioning triangleor at the flexion position determined during diagnostic arthroscopy at which the patella engages the trochlea. The graft is set to length alongside the medial surface of the patella at the position of the suture anchor, and a single stitch is placed through both ends of the graft at this adjacent position using a free needle (Davis Tonsil ½ Circle Taper Point; Vessel Medical). A hemostat is placed on the single suture throw to provisionally secure the graft in this trial position. This allows the surgeon to trial and examine the MPFL graft in extension, 45° of flexion, and terminal flexion. The goal is to provide a check rein to lateral translation of the patella and provide a firm endpoint to avoid further dislocation without overtension of the graft that may lead to increased contact pressures in the patellofemoral joint.
Once appropriate graft position is confirmed, using the double-loaded suture, we prefer to secure each limb separately to the decorticated medial patella. One end of the suture is threaded through the graft once and will act as a post. The other end of the suture is threaded through the graft 3 times in a modified Mason-Allen stitch. With the knee in that same position of flexion, the knot is tied and the graft is secured to the medial side of the patella. For secondary fixation, the remaining ends of the graft are passed below the periosteum on the anterior surface of the patella. Using a 0 Vicryl (Ethicon US), the periosteal flaps are sutured on top of the graft, incorporating the residual graft. The ends are cut and a repeat dynamic examination is performed to confirm the position of the patella and the patellar tracking and to ensure that overtensioning did not occur. Following irrigation, a standard closure is performed. The patient is placed in a hinged knee brace and cryotherapy is applied (Polar Care, Breg Inc).
POSTOPERATIVE PROTOCOL
For the initial or the acute postoperative phases, an emphasis on edema control, early quadriceps activation, and range of motion is recommended. We recommend weight-bearing as tolerated with the leg locked in extension until adequate quadriceps control is achieved. The patient must be able to perform 10 straight leg raises without an extension loss to be cleared to weight-bear as tolerated without motion restriction in the brace. Full motion is allowed immediately.
OUTCOMES
In our experience using isolated MPFL reconstruction in the skeletally immature patient, we have had no evidence of physeal arrest, leg-length inequality, or angular deformity, and only11.4% of patients have had recurrent instability. The mean Kujala score in this cohort was 90.4, with a mean Tegner activity of 7, after the procedure. All failures in our cohort had had severe trochlear dysplasia.
1. Chotel F, Berard J, Raux S. Patellar instability in children and adolescents. Orthop Traumatol Surg Res. 2014;100(1 Suppl.):S125-S137. doi: 10.1016/j.otsr.2013.06.014.
2. Gao B, Dwivedi S, Fabricant PD, Cruz AI Jr. Patterns in outcomes reporting of operatively managed pediatric patellofemoral instability: a systematic review and meta-analysis. Am J Sports Med. 2018;2:36354651876515. doi: 10.1177/0363546518765152.
3. Hennrikus W, Pylawka T. Patellofemoral instability in skeletally immature athletes. Instr Course Lect. 2013;62:445-453.
4. Vavken P, Wimmer MD, Camathias C, Quidde J, Valderrabano V, Pagenstert G. Treating patella instability in skeletally immature patients. Arthroscopy. 2013;29(8):1410-1422. doi: 10.1016/j.arthro.2013.03.075.
5. Askenberger M, Ekstrom W, Finnbogason T, Janarv PM. Occult intra-articular knee injuries in children With hemarthrosis. Am J Sports Med. 2014;42(7):1600-1606. doi: 10.1177/0363546514529639.
6. Seeley MA, Knesek M, Vanderhave KL. Osteochondral injury after acute patellar dislocation in children and adolescents. J Pediatr Orthop. 2013;33(5):511-518. doi: 10.1097/BPO.0b013e318288b7a0.
7. Cruz AI Jr, Milewski MD. Patellofemoral instability and other common knee issues in the skeletally immature knee (other knee pathology). In: Miller MD, ed. Orthopaedic Knowledge Update: Sports Medicine 5. 5th ed. Rosemont, IL: American Academy of Orthopaedic Surgeons; 2015.
8. Lewallen LW, McIntosh AL, Dahm DL. Predictors of recurrent instability after acute patellofemoral dislocation in pediatric and adolescent patients. Am J Sports Med. 2013;41(3):575-581. doi: 10.1177/0363546512472873.
9. Arshi A, Cohen JR, Wang JC, Hame SL, McAllister DR, Jones KJ. Operative management of patellar instability in the United States: an evaluation of national practice patterns, surgical trends, and complications. Orthop J Sports Med. 2016;4(8):2325967116662873. doi: 10.1177/2325967116662873.
10. Camathias C, Studer K, Kiapour A, Rutz E, Vavken P. Trochleoplasty as a solitary treatment for recurrent patellar dislocation results in good clinical outcome in adolescents. Am J Sports Med. 2016;44(11):2855-2863. doi: 10.1177/0363546516652894.
11. Jaquith BP, Parikh SN. Predictors of recurrent patellar iInstability in children and adolescents after first-time dislocation. J Pediatr Orthop. 2017;37(7):484-490. doi: 10.1097/BPO.0000000000000674.
12. Rong YH, Zhang GA, Wang C, Ning FG. [Quantification of type I and III collagen content in normal human skin in different age groups]. Zhonghua Shao Shang Za Zhi. 2008;24(1):51-53.
13. Wilson PL, Black SR, Ellis HB, Podeszwa DA. Distal femoral valgus and recurrent traumatic patellar instability: is an isolated varus producing distal femoral osteotomy a treatment option? J Pediatr Orthop. 2018;38(3):e162-e167. doi: 10.1097/BPO.0000000000001128.
14. Kearney SP, Mosca VS. Selective hemiepiphyseodesis for patellar instability with associated genu valgum. J Orthop. 2015;12(1):17-22. doi: 10.1016/j.jor.2015.01.005.
15. Kraus T, Lidder S, Svehlik M, Rippel K, Schneider F, Eberl R, Linhart W. Patella re-alignment in children with a modified Grammont technique. Acta Orthop. 2012;83(5):504-510. doi: 10.3109/17453674.2012.736168.
16. Shea KG, Grimm NL, Belzer J, Burks RT, Pfeiffer R. The relation of the femoral physis and the medial patellofemoral ligament. Arthroscopy. 2010;26(8):1083-1087. doi: 10.1016/j.arthro.2009.12.020.
17. Schottle PB, Schmeling A, Rosenstiel N, Weiler A. Radiographic landmarks for femoral tunnel placement in medial patellofemoral ligament reconstruction. Am J Sports Med. 2007;35(5):801-804. doi: 10.1177/0363546506296415.
18. Kepler CK, Bogner EA, Hammoud S, Malcolmson G, Potter HG, Green DW. Zone of injury of the medial patellofemoral ligament after acute patellar dislocation in children and adolescents. Am J Sports Med. 2011;39(7):1444-1449. doi: 10.1177/0363546510397174.
19. Nelitz M, Dornacher D, Dreyhaupt J, Reichel H, Lippacher S. The relation of the distal femoral physis and the medial patellofemoral ligament. Knee Surg Sports Traumatol Arthrosc. 2011;19(12):2067-2071. doi: 10.1007/s00167-011-1548-3.
20. Shea KG, Styhl AC, Jacobs JC Jr, Ganley TJ, Milewski MD, Cannamela PC, et al. The relationship of the femoral physis and the medial patellofemoral ligament in children: A Cadaveric Study. Am J Sports Med. 2016;44(11):2833-2837. doi: 10.1177/0363546516656366.
21. Popkin CA, Bayomy AF, Trupia EP, Chan CM, Redler LH. Patellar instability in the skeletally immature. Curr Rev Musculoskelet Med. 2018;11(2):172-181. doi: 10.1007/s12178-018-9472-5.
22. Keyes S, Price M, Green DW, Parikh SN. Special considerations for pediatric patellar instability. Am J Orthop Belle Mead NJ. 2018;47(3). doi: 10.12788/ajo.2018.0017.
23. Camp CL, Krych AJ, Dahm DL, Levy BA, Stuart MJ. Medial patellofemoral ligament repair for recurrent patellar dislocation. Am J Sports Med. 2010;38(11):2248-2254. doi: 10.1177/0363546510376230.
24. Fabricant PD, Ladenhauf HN, Salvati EA, Green DW, Green DW. Medial patellofemoral ligament (MPFL) reconstruction improves radiographic measures of Patella alta in children. Knee. 2014;21(6):1180-1184. doi: 10.1016/j.knee.2014.07.023.
25. Matic GT, Magnussen RA, Kolovich GP, Flanigan DC. Return to activity after medial patellofemoral ligament repair or reconstruction. Arthroscopy. 2014;30(8):1018-1025. doi: 10.1016/j.arthro.2014.02.044.
26. Mostrom EB, Mikkelsen C, Weidenhielm L, Janarv PM. Long-term follow-up of nonoperatively and operatively treated acute primary patellar dislocation in skeletally immature patients. Sciworldj. 2014;2014:473281. doi: 10.1155/2014/473281.
27. Antinolfi P, Bartoli M, Placella G, Speziali A, Pace V, Delcogliano M, Mazzola C. Acute patellofemoral instability in children and adolescents. Joints. 2016;4(1):47-51. doi: 10.11138/jts/2016.4.1.047.
28. Nelitz M, Dreyhaupt J, Reichel H, Woelfle J, Lippacher S. Anatomic reconstruction of the medial patellofemoral ligament in children and adolescents with open growth plates: surgical technique and clinical outcome. Am J Sports Med. 2013;41(1):58-63. doi: 10.1177/0363546512463683.
29. McNeilan RJ, Everhart JS, Mescher PK, Abouljoud M, Magnussen RA, Flanigan DC. Graft choice in isolated medial patellofemoral ligament reconstruction: A systematic review with meta-analysis of rates of recurrent instability and patient-reported outcomes for autograft, allograft, and synthetic options. Arthroscopy. 2018;34(4):1340-1354. doi: 10.1016/j.arthro.2017.11.027.
30. Shea KG, Polousky JD, Jacobs JC Jr, Ganley TJ, Aoki SK, Grimm NL, Parikh SN. The patellar insertion of the medial patellofemoral ligament in children: a cadaveric study. J Pediatr Orthop. 2015;35(4):e31-e35. doi: 10.1097/BPO.0000000000000399.
31. Parikh SN, Wall EJ. Patellar fracture after medial patellofemoral ligament surgery: a report of five cases. J Bone Joint Surg, (Am.). 2011;93(17):e97(1-8). doi: 10.2106/JBJS.J.01558.
1. Chotel F, Berard J, Raux S. Patellar instability in children and adolescents. Orthop Traumatol Surg Res. 2014;100(1 Suppl.):S125-S137. doi: 10.1016/j.otsr.2013.06.014.
2. Gao B, Dwivedi S, Fabricant PD, Cruz AI Jr. Patterns in outcomes reporting of operatively managed pediatric patellofemoral instability: a systematic review and meta-analysis. Am J Sports Med. 2018;2:36354651876515. doi: 10.1177/0363546518765152.
3. Hennrikus W, Pylawka T. Patellofemoral instability in skeletally immature athletes. Instr Course Lect. 2013;62:445-453.
4. Vavken P, Wimmer MD, Camathias C, Quidde J, Valderrabano V, Pagenstert G. Treating patella instability in skeletally immature patients. Arthroscopy. 2013;29(8):1410-1422. doi: 10.1016/j.arthro.2013.03.075.
5. Askenberger M, Ekstrom W, Finnbogason T, Janarv PM. Occult intra-articular knee injuries in children With hemarthrosis. Am J Sports Med. 2014;42(7):1600-1606. doi: 10.1177/0363546514529639.
6. Seeley MA, Knesek M, Vanderhave KL. Osteochondral injury after acute patellar dislocation in children and adolescents. J Pediatr Orthop. 2013;33(5):511-518. doi: 10.1097/BPO.0b013e318288b7a0.
7. Cruz AI Jr, Milewski MD. Patellofemoral instability and other common knee issues in the skeletally immature knee (other knee pathology). In: Miller MD, ed. Orthopaedic Knowledge Update: Sports Medicine 5. 5th ed. Rosemont, IL: American Academy of Orthopaedic Surgeons; 2015.
8. Lewallen LW, McIntosh AL, Dahm DL. Predictors of recurrent instability after acute patellofemoral dislocation in pediatric and adolescent patients. Am J Sports Med. 2013;41(3):575-581. doi: 10.1177/0363546512472873.
9. Arshi A, Cohen JR, Wang JC, Hame SL, McAllister DR, Jones KJ. Operative management of patellar instability in the United States: an evaluation of national practice patterns, surgical trends, and complications. Orthop J Sports Med. 2016;4(8):2325967116662873. doi: 10.1177/2325967116662873.
10. Camathias C, Studer K, Kiapour A, Rutz E, Vavken P. Trochleoplasty as a solitary treatment for recurrent patellar dislocation results in good clinical outcome in adolescents. Am J Sports Med. 2016;44(11):2855-2863. doi: 10.1177/0363546516652894.
11. Jaquith BP, Parikh SN. Predictors of recurrent patellar iInstability in children and adolescents after first-time dislocation. J Pediatr Orthop. 2017;37(7):484-490. doi: 10.1097/BPO.0000000000000674.
12. Rong YH, Zhang GA, Wang C, Ning FG. [Quantification of type I and III collagen content in normal human skin in different age groups]. Zhonghua Shao Shang Za Zhi. 2008;24(1):51-53.
13. Wilson PL, Black SR, Ellis HB, Podeszwa DA. Distal femoral valgus and recurrent traumatic patellar instability: is an isolated varus producing distal femoral osteotomy a treatment option? J Pediatr Orthop. 2018;38(3):e162-e167. doi: 10.1097/BPO.0000000000001128.
14. Kearney SP, Mosca VS. Selective hemiepiphyseodesis for patellar instability with associated genu valgum. J Orthop. 2015;12(1):17-22. doi: 10.1016/j.jor.2015.01.005.
15. Kraus T, Lidder S, Svehlik M, Rippel K, Schneider F, Eberl R, Linhart W. Patella re-alignment in children with a modified Grammont technique. Acta Orthop. 2012;83(5):504-510. doi: 10.3109/17453674.2012.736168.
16. Shea KG, Grimm NL, Belzer J, Burks RT, Pfeiffer R. The relation of the femoral physis and the medial patellofemoral ligament. Arthroscopy. 2010;26(8):1083-1087. doi: 10.1016/j.arthro.2009.12.020.
17. Schottle PB, Schmeling A, Rosenstiel N, Weiler A. Radiographic landmarks for femoral tunnel placement in medial patellofemoral ligament reconstruction. Am J Sports Med. 2007;35(5):801-804. doi: 10.1177/0363546506296415.
18. Kepler CK, Bogner EA, Hammoud S, Malcolmson G, Potter HG, Green DW. Zone of injury of the medial patellofemoral ligament after acute patellar dislocation in children and adolescents. Am J Sports Med. 2011;39(7):1444-1449. doi: 10.1177/0363546510397174.
19. Nelitz M, Dornacher D, Dreyhaupt J, Reichel H, Lippacher S. The relation of the distal femoral physis and the medial patellofemoral ligament. Knee Surg Sports Traumatol Arthrosc. 2011;19(12):2067-2071. doi: 10.1007/s00167-011-1548-3.
20. Shea KG, Styhl AC, Jacobs JC Jr, Ganley TJ, Milewski MD, Cannamela PC, et al. The relationship of the femoral physis and the medial patellofemoral ligament in children: A Cadaveric Study. Am J Sports Med. 2016;44(11):2833-2837. doi: 10.1177/0363546516656366.
21. Popkin CA, Bayomy AF, Trupia EP, Chan CM, Redler LH. Patellar instability in the skeletally immature. Curr Rev Musculoskelet Med. 2018;11(2):172-181. doi: 10.1007/s12178-018-9472-5.
22. Keyes S, Price M, Green DW, Parikh SN. Special considerations for pediatric patellar instability. Am J Orthop Belle Mead NJ. 2018;47(3). doi: 10.12788/ajo.2018.0017.
23. Camp CL, Krych AJ, Dahm DL, Levy BA, Stuart MJ. Medial patellofemoral ligament repair for recurrent patellar dislocation. Am J Sports Med. 2010;38(11):2248-2254. doi: 10.1177/0363546510376230.
24. Fabricant PD, Ladenhauf HN, Salvati EA, Green DW, Green DW. Medial patellofemoral ligament (MPFL) reconstruction improves radiographic measures of Patella alta in children. Knee. 2014;21(6):1180-1184. doi: 10.1016/j.knee.2014.07.023.
25. Matic GT, Magnussen RA, Kolovich GP, Flanigan DC. Return to activity after medial patellofemoral ligament repair or reconstruction. Arthroscopy. 2014;30(8):1018-1025. doi: 10.1016/j.arthro.2014.02.044.
26. Mostrom EB, Mikkelsen C, Weidenhielm L, Janarv PM. Long-term follow-up of nonoperatively and operatively treated acute primary patellar dislocation in skeletally immature patients. Sciworldj. 2014;2014:473281. doi: 10.1155/2014/473281.
27. Antinolfi P, Bartoli M, Placella G, Speziali A, Pace V, Delcogliano M, Mazzola C. Acute patellofemoral instability in children and adolescents. Joints. 2016;4(1):47-51. doi: 10.11138/jts/2016.4.1.047.
28. Nelitz M, Dreyhaupt J, Reichel H, Woelfle J, Lippacher S. Anatomic reconstruction of the medial patellofemoral ligament in children and adolescents with open growth plates: surgical technique and clinical outcome. Am J Sports Med. 2013;41(1):58-63. doi: 10.1177/0363546512463683.
29. McNeilan RJ, Everhart JS, Mescher PK, Abouljoud M, Magnussen RA, Flanigan DC. Graft choice in isolated medial patellofemoral ligament reconstruction: A systematic review with meta-analysis of rates of recurrent instability and patient-reported outcomes for autograft, allograft, and synthetic options. Arthroscopy. 2018;34(4):1340-1354. doi: 10.1016/j.arthro.2017.11.027.
30. Shea KG, Polousky JD, Jacobs JC Jr, Ganley TJ, Aoki SK, Grimm NL, Parikh SN. The patellar insertion of the medial patellofemoral ligament in children: a cadaveric study. J Pediatr Orthop. 2015;35(4):e31-e35. doi: 10.1097/BPO.0000000000000399.
31. Parikh SN, Wall EJ. Patellar fracture after medial patellofemoral ligament surgery: a report of five cases. J Bone Joint Surg, (Am.). 2011;93(17):e97(1-8). doi: 10.2106/JBJS.J.01558.
TAKE-HOME POINTS
- Patellofemoral instability is common in the skeletally immature age group.
- Many treatment options exist for patellofemoral instability; however, a medial patellofemoral ligament (MPFL) reconstruction is an option in a skeletally immature patient.
- Allograft or autografts may be utilized for the MPFL reconstruction.
- Three options exist to confirm location of the MPFL origin on the patella, but only one drill tunnel is recommended in pediatrics.
- During identification of MPFL origin, special views in the coronal plane should be considered to avoid injury or damage to the growth plate.
Current Concepts: Evaluation and Treatment of Discoid Meniscus in the Pediatric Athlete
ABSTRACT
Discoid meniscus is a rare anatomical variant with altered morphology and structure that can sometimes present symptomatically, typically in the pediatric population. The discoid meniscus is usually in the lateral compartment of the knee and is characterized by a partial or complete filling-in of central meniscal tissue, increased meniscal thickness, disorganization of longitudinal collagen fibers, and sometimes lack of peripheral attachments. These changes to both the macro- and micro-structure of the meniscus predispose affected patients to increased rates of both meniscal tears and mechanical symptoms. Surgical management of symptomatic discoid meniscus is directed toward symptom resolution while preserving sufficient functional meniscal tissue to delay or prevent the development of osteoarthritis. Modern surgical techniques consist of arthroscopic saucerization of the discoid meniscus with repair of associated meniscal tears and stabilization of peripheral attachments. Although long-term outcome data are lacking, short- and mid-term outcomes for patients treated with arthroscopic partial meniscectomy and meniscal repair and/or stabilization as needed are generally good.
Continue to: The discoid meniscus...
The discoid meniscus is an uncommon anatomical meniscal variant that may present with pain, snapping, motion loss, swelling, and locking of the affected knee, typically during childhood or adolescence.1 Although the etiology of discoid meniscus is not completely understood, it is considered a congenital anomaly with a possible genetic component.2, 3 Incidence is estimated at 0.4% to 5.2% in the Western European population but is reportedly much higher (roughly 17%) in Asian populations.4-6, Discoid menisci, when present, are almost always in the lateral compartment, although cases of medial discoid meniscus have also been reported. The overall incidence of medial discoid meniscus has been estimated to be between 0.06% and 0.3% while that of lateral discoid meniscus, as detailed above, is significantly higher.7-11
ANATOMY AND MORPHOLOGY
The menisci differentiate from mesenchymal tissue early during fetal development with a clear definition by 8 weeks and a mature anatomical shape by 14 weeks in utero.12 Interestingly, menisci never possess a discoid shape during the normal course of development.13,14 The meniscus is fully vascularized at birth, with the central one-third becoming avascular by 9 months as the vascular supply regresses.14 By 10 years, only the peripheral one-third maintains its vascular supply, and the menisci have adult-like characteristics with tissue composed mainly of circumferential collagen fibers.4,14-16
A normal meniscus is wedge-shaped in the coronal plane and crescent-shaped in the axial plane. The medial meniscus is shaped like the letter “C,” is connected firmly to the joint capsule, and covers approximately 50% of the medial tibial plateau. The normal lateral meniscus covers 70% of the lateral tibial plateau and has firm anterior and posterior attachments while the lateral portion is less securely tethered.17 A discoid meniscus is characterized by an atypical shape—the center being partially or completely filled in (or “disc-like”)—often associated with anomalous attachments to the tibia, femur, and surrounding joint capsule. In addition to an atypical morphology, the discoid meniscus may also be much thicker than normal, resulting in a “block-like” appearance. The increased thickness may be responsible for the pathognomonic snapping of the knee as it is brought passively into flexion or extension (hence, the term “snapping knee” was traditionally used to describe a symptomatic discoid lateral meniscus). While the discoid shape is relatively straightforward to address, the increased meniscal thickness may be quite difficult to correct surgically.4-6,18
In addition to the macromorphological differences characteristic of discoid menisci, histological differences have also been described, including differences in collagen density and disorganization of the circumferential collagen network.19-21 Taken together, these differences may compromise the ability of the discoid meniscus to withstand normal forces placed across the knee and predispose it to tear. This is important because the normal meniscus plays a critical role in facilitating load distribution across the knee joint, in addition to assisting with functions including shock absorption, proprioception, and stabilization of the knee. Torn and/or unstable discoid menisci are unable to perform these functions adequately, which may ultimately result in degenerative joint disease and progress to end-stage osteoarthritis (OA).
CLASSIFICATION
The traditional Watanabe classification of discoid meniscus consists of 3 variants: type I, a complete discoid shape; type II, an incomplete discoid shape; and type III, the Wrisberg variant.22 The Wrisberg variant is described as a more normal-appearing meniscus but lacks normal peripheral attachments.5, 6, 15 This classification system was expanded by Monllau and colleagues, 23 who described a ring-type meniscus variant with normal posterior tibial attachments. Although the Watanabe classification is commonly used, its utility in assisting with clinical decision-making may be limited.
Continue to: Several other classification schemes...
Several other classification schemes exist. Jordan and colleagues24 described a classification scheme defining a meniscal type as complete or incomplete, also noting the presence of symptoms, tearing, and peripheral rim instability. They grouped stable types together, regardless of morphology, and then further classified them based on the presence of symptoms and tears. Similarly, the unstable types were grouped together and then subclassified in the same manner.17,24 Klingele and colleagues25 also described a contemporary classification scheme of discoid meniscus evaluating peripheral stability patterns that may be more clinically and surgically relevant. This classification is based on the type of discoid morphology (complete vs incomplete), the presence or absence of peripheral rim stability (stable vs unstable), and the presence or absence of a meniscal tear (torn vs untorn).5,25
EVALUATION
A stable discoid meniscus is often an incidental finding, seen either on advanced diagnostic imaging performed for another reason or at the time of arthroscopy to address another problem. Younger children with discoid meniscus tend to present with symptoms such as popping and snapping related to instability and the abnormal morphology of the discoid meniscus. Older patients tend to present with symptoms related to acute tears through the abnormal meniscal tissue. Although discoid menisci can become acutely symptomatic in the presence of a tear, the onset of symptoms may occur in the absence of a discrete traumatic event.1 Alternatively, some patients will report a clear history of injury, often a noncontact, rotational injury mechanism related to an athletic activity. Patients with torn discoid menisci may report pain, catching, locking, and/or giving way of the knee, and on examination may have limited extension, snapping, effusion, quadriceps atrophy, and joint line tenderness. Eponymous meniscal compression tests including the McMurray, Apley, and Thessaly tests, may also be performed when meniscal tear is suspected, although this may be tricky for younger children.1
Considering the high association of meniscal tears with ligamentous injuries, examination of knee stability is important. Plain radiographs of the knee should be taken, although the results will often be negative for osseous injury in the case of an isolated meniscal tear. Radiographs of a discoid knee may show subtle differences compared with radiographs of a non-discoid knee. A recent comparison of children with symptomatic lateral discoid menisci with age-matched controls found statistically significant increased lateral joint space, elevated fibular head, increased height of the lateral tibial spine, and increased obliquity of the tibial plateau.26 They did not find statistically significant increased squaring of the lateral femoral condyle or cupping of the lateral tibial plateau. Radiographic signs can be subtle and may not all be present in a patient with a discoid meniscus.
Magnetic resonance imaging (MRI) is the assessment technique of choice for the diagnosis of discoid meniscus, although MRI may not reliably identify a Wrisberg variant or incomplete discoid menisci (Figure 1).
Gans and colleagues27 examined preoperative MRI and clinical examination compared with pathology found during arthroscopy. Although they found that MRI and clinical examination had excellent diagnostic accuracy of 92.7% and 95.3%, respectively, the most common missed pathology on MRI later found on diagnostic arthroscopy was the presence of a lateral discoid meniscus, which occurred in 26.7% of missed diagnoses. Adult diagnostic criteria of discoid meniscus include ≥3 contiguous 5-mm sagittal cuts showing continuity between the anterior and posterior horns of the meniscus. Other criteria include a minimal meniscal width >15 mm on the coronal view or a minimum meniscal width that is >20% of the width of the maximal tibial width.28 These criteria are often applied to children as well. Additionally, if >50% of the lateral joint space is covered by meniscal tissue, a diagnosis of discoid meniscus should be considered.6
Continue to: TREATMENT
TREATMENT
Management of symptomatic discoid meniscus is directed toward resolving symptoms while preserving meniscal tissue and preventing the development of OA. Incidentally found discoid menisci in asymptomatic patients should not be treated surgically and can have periodic follow-ups for detection of any functional deterioration or symptom development. Surgical treatment may be beneficial for patients with symptoms related to their discoid menisci such as pain, recurrent effusion, limited knee motion, mechanical symptoms (e.g., locking and catching), and activity restrictions.
Traditionally, surgical treatment of a symptomatic discoid meniscus consisted of subtotal (meaning nearly complete, or <3mm of peripheral rim remaining) or complete meniscectomy, often performed in an open fashion. Surgical techniques have evolved; current surgical approaches typically include diagnostic arthroscopy followed by arthroscopic saucerization (also called partial meniscectomy) of the central portion of the “disc,” leaving a peripheral rim of 6 mm to 8 mm to approximate that of the normal meniscus (Figure 2).29,30 Saucerization removes the redundant central meniscal tissue in an attempt to create a more “normal” C-shaped morphology, although it does not address the increased thickness characteristic of many discoid menisci. It may be particularly difficult to “debulk” an abnormally thick discoid meniscus in the coronal plane, and there is little in the outcomes literature to support this approach. Following partial meniscectomy, the remaining meniscal tissue should be inspected thoroughly both for the presence of instability and for residual tears. Meniscal tears should be repaired in standard fashion; commonly, this may be a combination of all-inside sutures placed into the posterior horn, inside-out sutures placed into the midbody, and outside-in sutures placed into the anterior horn. Peripheral rim instability—identified, for example, by the ability to translate the posterior horn of the meniscus fully onto the anterior tibial plateau—should be addressed by suturing the meniscus to the adjacent capsule (Figure 2).25, 31 Menisci that have residual complex tears that are not amenable to repair may be treated by subtotal meniscectomy. These patients may be candidates for subsequent meniscal allograft transplantation.32,33
TREATMENT OUTCOMES
Performing a partial meniscectomy has consistently been shown in the laboratory to increase contact stresses proportionally to the percentage of tissue removed.34 It follows logically that performing a subtotal or complete meniscectomy in a young patient would yield increased, abnormal contact stresses throughout the knee with resultant degenerative changes and progression to OA over time. While long-term outcome studies of subtotal or complete meniscectomy have shown somewhat variable results, the majority report the development of pain, instability, poor function, osteoarthritic changes and even the development of osteochondritis desiccans.5,35
In an early investigation of long-term outcomes associated with surgical resection of a discoid lateral meniscus, Räber and colleagues36 retrospectively examined 17 knees that had undergone total meniscectomy for the lateral discoid meniscus. At a mean follow-up of 19.8 years, these authors reported that patients accounting for 10 of 17 operative knees had developed symptoms of osteoarthrosis such as pain. In addition, two-thirds of the knees that had follow-up radiographs performed (10 of 15 knees) had visible osteoarthritic changes present.
In 2011, Stilli and colleagues37 conducted a mid-term follow-up study examining 104 knees over an average follow-up of 8.5 years, with an average age of 8 years at the time of surgery. Younger patients, 2 to 7 years, underwent subtotal meniscectomy whereas patients, 8 to 14 years, underwent arthroscopic partial meniscectomy. Patients with a Wrisberg variant underwent removal of the posterior horn. The authors of this study reported that younger patients who had undergone subtotal meniscectomy had the best outcomes (defined by self-reported questionnaire responses and clinical evaluation). However, these results should be interpreted with caution: at the time of follow-up, patients in this study would still have been in adolescence and may not yet have developed the symptomatic degenerative changes in the knee joint that are strongly associated with meniscectomy.
Continue to: There are a few long-term...
There are few long-term outcome studies for arthroscopic saucerization. In 2014, Ahn and colleagues38 examined patient-reported outcomes and radiographic changes for 48 knees in pediatric patients treated arthroscopically for a symptomatic discoid meniscus. Patients were treated with partial meniscectomy (22 knees), partial meniscectomy with meniscal repair (18 knees), or subtotal meniscectomy (8 knees). Out of 48 knees evaluated, 45 knees (94%) were rated as “excellent” or “good” at an average 10-year follow-up. However, a significant percentage of patients had evidence of degenerative changes on follow-up radiographs, specifically: 88% of the subtotal meniscectomy group, 39% of the partial meniscectomy with repair group, and 23% in the partial meniscectomy-alone group. This finding suggests that the early appearance of radiographic changes suggestive of OA does not necessarily correlate with the development of knee symptoms in this cohort, although longer-term follow-up of these patients into adulthood and middle age is clearly needed.
Recently, short-term outcomes have been reported for contemporary arthroscopic saucerization of discoid menisci with peripheral rim stabilization performed as deemed necessary at the time of surgery. In 2012, Carter and colleagues39 examined 57 knees that underwent saucerization alone or saucerization with peripheral rim stabilization. At an average follow-up of 15 months, both patient populations had equivalent self-reported outcomes and clinical examination findings. The authors concluded that peripheral stabilization does not negatively affect short-term outcomes if instability is recognized and treated.
Yoo and colleagues40 found equivalent results between patients undergoing saucerization, saucerization with rim stabilization, and subtotal meniscectomy for 100 pediatric knees with an average follow-up of 4.7 years. Subtotal meniscectomy was defined as <3 mm of peripheral rim remaining and was performed when there was severe degeneration or complex tearing of the remnant rim following arthroscopic saucerization. Taken together, these data seem to suggest that short- and mid-term outcomes for pediatric patients treated surgically are generally good in terms of reported clinical function and development of OA, although long-term follow-up studies will be essential for understanding the true impact of surgical interventions.
Shieh and colleagues41,42 investigated risk factors for failed meniscal surgery in 324 menisci from 2008 to 2012, including in 46 discoid saucerization procedures with or without stabilization. At a mean of 40 months, 15% of the discoid saucerization cohort required a revision procedure, with increased odds of revision surgeries in patients who had undergone meniscal tear repair. The most frequent indication of revision surgery was sustaining a meniscal tear during intense physical activity in the first year after the index procedure, and patients underwent either debridement or repair for their revision procedure.
SUMMARY
Discoid meniscus is a rare anatomical meniscal variant characterized by an abnormal “O-like” shape, increased meniscal thickness, disorganization of collagen fibers, and variable absence of peripheral attachments. These morphological changes predispose patients with discoid menisci to increased rates of symptomatic meniscal instability and tearing. An MRI remains the most sensitive imaging modality for the diagnosis of a discoid meniscus, with ≥3 contiguous 5-mm sagittal cuts showing continuity between the anterior and posterior horns indicative of a discoid variant. Surgical treatment for symptomatic discoid menisci has evolved from subtotal meniscectomy to contemporary arthroscopic saucerization techniques with repair of meniscal tears and peripheral stabilization performed as needed. Long-term outcome studies for patients who undergo subtotal or complete meniscectomy reveal an association with osteoarthritic changes of the knee. Short- and mid-term outcome studies for patients who undergo arthroscopic saucerization with or without repair and/or peripheral stabilization are generally good, although reoperation rates have been estimated at 15% in the first 3 to 4 years. Longer-term follow-up, with the inclusion of validated functional outcomes measures, will be essential for understanding the true impact of various surgical interventions over time.
1. Yaniv M, Blumberg N. The discoid meniscus. J Child Orthop. 2007;1(2):89-96. doi: 10.1007/s11832-007-0029-1.
2. Gebhardt M, Rosenthal R. Bilateral lateral discoid meniscus in identical twins. J Bone Joint Surg Am. 1979;61(7):1110-1111. doi: 10.2106/00004623-197961070-00027.
3. Dashefsky JH. Discoid lateral meniscus in three members of a family. J Bone Joint Surg, (Am.). 1971;53(6):1208-1210. doi: 10.2106/00004623-197153060-00018.
4. Francavilla ML, Restrepo R, Zamora KW, Sarode V, Swirsky SM, Mintz D. Meniscal pathology in children: differences and similarities with the adult meniscus. Pediatr Radiol. 2014;44(8):910-925. doi: 10.1007/s00247-014-3022-0.
5. Kushare I, Klingele K, Samora W. Discoid meniscus: diagnosis and management. Orthop Clin North Am. 2015;46(4):533-540. doi: 10.1016/j.ocl.2015.06.007.
6. McKay S, Chen C, Rosenfeld S. Orthopedic perspective on selected pediatric and adolescent knee conditions. Pediatr Radiol. 2013;43(1):99-106. doi: 10.1007/s00247-012-2587-8.
7. Greis PE, Bardana DD, Holmstrom MC, Burks RT. Meniscal injury: I. Basic science and evaluation. J Am Acad Orthop Surg. 2002;10(3):168-176. doi: 10.5435/00124635-200205000-00003.
8. Ikeuchi H. Arthroscopic treatment of the discoid lateral meniscus. Technique and long-term results. Clin Orthop Relat Res. 1982;167(167):19-28.
9. Nathan PA, Cole SC. 12 Discoid meniscus: a clinical and pathologic study. Clin Orthop Relat Res: SC: Cole. 1969;64:107-113.
10. Jeannopoulos CL. Observations on discoid menisci. J Bone Joint Surg, (Am.). 1950;32(3):649-652. doi: 10.2106/00004623-195032030-00019.
11. Dickason J, Del WP, Blazina ME, Fox JM, Friedman MJ, Snyder SJ. A series of ten discoid medial menisci. Clin Orthop Relat Res. 1982;168:75-79. doi: 10.1097/00003086-198208000-00014
12. Andrish JT. Meniscal injuries in children and adolescents: diagnosis and management. J Am Acad Orthop Surg. 1996;4(5):231-237. doi: 10.5435/00124635-199609000-00001.
13. Kaplan E. The embryology of the menisci of the knee joint. Bull Hosp Joint Dis. 1955;16(2):111-124.
14. Clark C, Ogden J. Development of the menisci of the human knee joint. Morphological changes and their potential role in childhood meniscal injury. J Bone Joint Surg, (Am.). 1983;65(4):538-547 doi: 10.2106/00004623-198365040-00018.
15. Carter CW, Kocher MS. Meniscus repair in children. Clin Sports Med. 2012;31(1):135-154. doi: 10.1016/j.csm.2011.09.002.
16. Shieh A, Bastrom T, Roocroft J, Edmonds EW, Pennock AT. Meniscus tear patterns in relation to skeletal immaturity: children versus adolescents. Am J Sports Med. 2013;41(12):2779-2783. doi: 10.1177/0363546513504286.
17. Jordan MR. Lateral meniscal variants: evaluation and treatment. J Am Acad Orthopsurg. 1996;4(4):191-200. doi: 10.5435/00124635-199607000-00003.
18. Flouzat-Lachaniette C, Pujol N, Boisrenoult P, Beaufils P. Discoid medial meniscus: report of four cases and literature review. Orthop Traumatol Surg Res. 2011;97(8):826-832. doi: 10.1016/j.otsr.2011.07.011.
19. Choi Y-H, Seo Y-J, Ha JM, Jung KH, Kim J, Song SY. Collagenous ultrastructure of the discoid meniscus: A Transmission Electron Microscopy Study. Am J Sports Med. 2017;45(3):598-603. doi: 10.1177/0363546516674181.
20. Atay OA, Pekmezci M, Doral MN, Sargon MF, Ayvaz M, Johnson DL. Discoid meniscus: an ultrastructural study with transmission electron microscopy. Am J Sports Med. 2007;35(3):475-478. doi: 10.1177/0363546506294678.
21. Papadopoulos A, Kirkos JM, Kapetanos GA. Histomorphologic study of discoid meniscus. Arthroscopy. 2009;25(3):262-268. doi: 10.1016/j.arthro.2008.10.006.
22. Watanabe M, Takeda S, Ikeuchi H. Atlas of Arthroscopy. Tokyo: Igaku-Shoin Ltd; 1969.
23. Monllau JC, León A, Cugat R, Ballester J. Ring-shaped lateral meniscus. Arthroscopy. 1998;14(5):502-504. doi: 10.1016/S0749-8063(98)70079-9.
24. Jordan M, Duncan J, Bertrand S. Discoid lateral meniscus: a review. S Orthop J. 1993;2(4):239-253.
25. Klingele KE, Kocher MS, Hresko MT, Gerbino P, Micheli LJ. Discoid lateral meniscus: prevalence of peripheral rim instability. J Pediatr Orthop. 2004;24(1):79-82. doi: 10.1097/01241398-200401000-00015.
26. Choi SH, Ahn JH, Kim KI, et al. Do the radiographic findings of symptomatic discoid lateral meniscus in children differ from normal control subjects? Knee Surg Sports Traumatol Arthrosc. 2015;23(4):1128-1134. doi: 10.1007/s00167-014-2924-6.
27. Gans I, Bedoya MA, Ho-Fung V, Ganley TJ. Diagnostic performance of magnetic resonance imaging and pre-surgical evaluation in the assessment of traumatic intra-articular knee disorders in children and adolescents: what conditions still pose diagnostic challenges? Pediatr Radiol. 2015;45(2):194-202. doi: 10.1007/s00247-014-3127-5.
28. Samoto N, Kozuma M, Tokuhisa T, Kobayashi K. Diagnosis of discoid lateral meniscus of the knee on MR imaging. Magn Reson Imaging. 2002;20(1):59-64. doi: 10.1016/S0730-725X(02)00473-3.
29. Hayashi LK, Yamaga H, Ida K, Miura T. Arthroscopic meniscectomy for discoid lateral meniscus in children. J Bone Joint Surg, (Am.). 1988;70(10):1495-1500. doi: 10.2106/00004623-198870100-00009.
30. Kim S-J, Kim D-W, Min B-H. Discoid lateral meniscus associated with anomalous insertion of the medial meniscus. Clin Orthop Rel Res. 1995;315(315):234-237 doi: 10.1097/00003086-199506000-00026.
31. Adachi N, Ochi M, Uchio Y, Kuriwaka M, Shinomiya R. Torn discoid lateral meniscus treated using partial central meniscectomy and suture of the peripheral tear. Arthroscopy. 2004;20(5):536-542. doi: 10.1016/j.arthro.2004.01.028.
32. Kim J-M, Bin S-I. Meniscal allograft transplantation after total meniscectomy of torn discoid lateral meniscus. Arthroscopy. 2006;22(12):1344-1350.e1. doi: 10.1016/j.arthro.2006.07.048.
33. Ramme AJ, Strauss EJ, Jazrawi L, Gold HT. Cost effectiveness of meniscal allograft for torn discoid lateral meniscus in young women. Phys Sportsmed. 2016;44(3):278-282. doi: 10.1080/00913847.2016.1197762.
34. Baratz ME, Fu FH, Mengato R. Meniscal tears: the effect of meniscectomy and of repair on intraarticular contact areas and stress in the human knee: a preliminary report. Am J Sports Med. 1986;14(4):270-275. doi: 10.1177/036354658601400405.
35. Wroble RR, Henderson RC, Campion ER, el-Khoury GY, Albright JP. Meniscectomy in children and adolescents. A long-term follow-up study. Clin Orthop Relat Res. 1992;279(279):180-189.
36. Räber D, Friederich N, Hefti F. Discoid lateral meniscus in children. Long-term follow-up after total meniscectomy. J Bone Joint Surg, (Am.). 1998;80(11):1579-1586. doi: 10.2106/00004623-199811000-00003.
37. Stilli S, Reggiani LM, Muccioli GMM, Cappella M, Donzelli O. Arthroscopic treatment for symptomatic discoid lateral meniscus during childhood. Knee Surg Sports Traumatol Arthrosc. 2011;19(8):1337-1342. doi: 10.1007/s00167-011-1440-1.
38. Ahn JH, Kim KI, Wang JH, Jeon JW, Cho YC, Lee SH. Long-term results of arthroscopic reshaping for symptomatic discoid lateral meniscus in children. Arthroscopy. 2015;31(5):867-873. doi: 10.1016/j.arthro.2014.12.012.
39. Carter CW, Hoellwarth J, Weiss JM. Clinical outcomes as a function of meniscal stability in the discoid meniscus: a preliminary report. J Pediatr Orthop. 2012;32(1):9-14. doi: 10.1097/BPO.0b013e31823d8338.
40. Yoo WJ, Jang WY, Park MS, et al. Arthroscopic treatment for symptomatic discoid meniscus in children: midterm outcomes and prognostic factors. Arthroscopy. 2015;31(12):2327-2334. doi: 10.1016/j.arthro.2015.06.032.
41. Shieh A, Edmonds EW, Pennock AT. Revision meniscus surgery in children and adolescents: the effect of skeletal immaturity. Orthop J Sports Med. 2015;3(7):S00075:2325967115. doi: 10.1177/2325967115S00075.
42. Shieh AK, Edmonds EW, Pennock AT. Revision meniscal surgery in children and adolescents: risk factors and mechanisms for failure and subsequent management. Am J Sports Med. 2016;44(4):838-843. doi: 10.1177/0363546515623511.
ABSTRACT
Discoid meniscus is a rare anatomical variant with altered morphology and structure that can sometimes present symptomatically, typically in the pediatric population. The discoid meniscus is usually in the lateral compartment of the knee and is characterized by a partial or complete filling-in of central meniscal tissue, increased meniscal thickness, disorganization of longitudinal collagen fibers, and sometimes lack of peripheral attachments. These changes to both the macro- and micro-structure of the meniscus predispose affected patients to increased rates of both meniscal tears and mechanical symptoms. Surgical management of symptomatic discoid meniscus is directed toward symptom resolution while preserving sufficient functional meniscal tissue to delay or prevent the development of osteoarthritis. Modern surgical techniques consist of arthroscopic saucerization of the discoid meniscus with repair of associated meniscal tears and stabilization of peripheral attachments. Although long-term outcome data are lacking, short- and mid-term outcomes for patients treated with arthroscopic partial meniscectomy and meniscal repair and/or stabilization as needed are generally good.
Continue to: The discoid meniscus...
The discoid meniscus is an uncommon anatomical meniscal variant that may present with pain, snapping, motion loss, swelling, and locking of the affected knee, typically during childhood or adolescence.1 Although the etiology of discoid meniscus is not completely understood, it is considered a congenital anomaly with a possible genetic component.2, 3 Incidence is estimated at 0.4% to 5.2% in the Western European population but is reportedly much higher (roughly 17%) in Asian populations.4-6, Discoid menisci, when present, are almost always in the lateral compartment, although cases of medial discoid meniscus have also been reported. The overall incidence of medial discoid meniscus has been estimated to be between 0.06% and 0.3% while that of lateral discoid meniscus, as detailed above, is significantly higher.7-11
ANATOMY AND MORPHOLOGY
The menisci differentiate from mesenchymal tissue early during fetal development with a clear definition by 8 weeks and a mature anatomical shape by 14 weeks in utero.12 Interestingly, menisci never possess a discoid shape during the normal course of development.13,14 The meniscus is fully vascularized at birth, with the central one-third becoming avascular by 9 months as the vascular supply regresses.14 By 10 years, only the peripheral one-third maintains its vascular supply, and the menisci have adult-like characteristics with tissue composed mainly of circumferential collagen fibers.4,14-16
A normal meniscus is wedge-shaped in the coronal plane and crescent-shaped in the axial plane. The medial meniscus is shaped like the letter “C,” is connected firmly to the joint capsule, and covers approximately 50% of the medial tibial plateau. The normal lateral meniscus covers 70% of the lateral tibial plateau and has firm anterior and posterior attachments while the lateral portion is less securely tethered.17 A discoid meniscus is characterized by an atypical shape—the center being partially or completely filled in (or “disc-like”)—often associated with anomalous attachments to the tibia, femur, and surrounding joint capsule. In addition to an atypical morphology, the discoid meniscus may also be much thicker than normal, resulting in a “block-like” appearance. The increased thickness may be responsible for the pathognomonic snapping of the knee as it is brought passively into flexion or extension (hence, the term “snapping knee” was traditionally used to describe a symptomatic discoid lateral meniscus). While the discoid shape is relatively straightforward to address, the increased meniscal thickness may be quite difficult to correct surgically.4-6,18
In addition to the macromorphological differences characteristic of discoid menisci, histological differences have also been described, including differences in collagen density and disorganization of the circumferential collagen network.19-21 Taken together, these differences may compromise the ability of the discoid meniscus to withstand normal forces placed across the knee and predispose it to tear. This is important because the normal meniscus plays a critical role in facilitating load distribution across the knee joint, in addition to assisting with functions including shock absorption, proprioception, and stabilization of the knee. Torn and/or unstable discoid menisci are unable to perform these functions adequately, which may ultimately result in degenerative joint disease and progress to end-stage osteoarthritis (OA).
CLASSIFICATION
The traditional Watanabe classification of discoid meniscus consists of 3 variants: type I, a complete discoid shape; type II, an incomplete discoid shape; and type III, the Wrisberg variant.22 The Wrisberg variant is described as a more normal-appearing meniscus but lacks normal peripheral attachments.5, 6, 15 This classification system was expanded by Monllau and colleagues, 23 who described a ring-type meniscus variant with normal posterior tibial attachments. Although the Watanabe classification is commonly used, its utility in assisting with clinical decision-making may be limited.
Continue to: Several other classification schemes...
Several other classification schemes exist. Jordan and colleagues24 described a classification scheme defining a meniscal type as complete or incomplete, also noting the presence of symptoms, tearing, and peripheral rim instability. They grouped stable types together, regardless of morphology, and then further classified them based on the presence of symptoms and tears. Similarly, the unstable types were grouped together and then subclassified in the same manner.17,24 Klingele and colleagues25 also described a contemporary classification scheme of discoid meniscus evaluating peripheral stability patterns that may be more clinically and surgically relevant. This classification is based on the type of discoid morphology (complete vs incomplete), the presence or absence of peripheral rim stability (stable vs unstable), and the presence or absence of a meniscal tear (torn vs untorn).5,25
EVALUATION
A stable discoid meniscus is often an incidental finding, seen either on advanced diagnostic imaging performed for another reason or at the time of arthroscopy to address another problem. Younger children with discoid meniscus tend to present with symptoms such as popping and snapping related to instability and the abnormal morphology of the discoid meniscus. Older patients tend to present with symptoms related to acute tears through the abnormal meniscal tissue. Although discoid menisci can become acutely symptomatic in the presence of a tear, the onset of symptoms may occur in the absence of a discrete traumatic event.1 Alternatively, some patients will report a clear history of injury, often a noncontact, rotational injury mechanism related to an athletic activity. Patients with torn discoid menisci may report pain, catching, locking, and/or giving way of the knee, and on examination may have limited extension, snapping, effusion, quadriceps atrophy, and joint line tenderness. Eponymous meniscal compression tests including the McMurray, Apley, and Thessaly tests, may also be performed when meniscal tear is suspected, although this may be tricky for younger children.1
Considering the high association of meniscal tears with ligamentous injuries, examination of knee stability is important. Plain radiographs of the knee should be taken, although the results will often be negative for osseous injury in the case of an isolated meniscal tear. Radiographs of a discoid knee may show subtle differences compared with radiographs of a non-discoid knee. A recent comparison of children with symptomatic lateral discoid menisci with age-matched controls found statistically significant increased lateral joint space, elevated fibular head, increased height of the lateral tibial spine, and increased obliquity of the tibial plateau.26 They did not find statistically significant increased squaring of the lateral femoral condyle or cupping of the lateral tibial plateau. Radiographic signs can be subtle and may not all be present in a patient with a discoid meniscus.
Magnetic resonance imaging (MRI) is the assessment technique of choice for the diagnosis of discoid meniscus, although MRI may not reliably identify a Wrisberg variant or incomplete discoid menisci (Figure 1).
Gans and colleagues27 examined preoperative MRI and clinical examination compared with pathology found during arthroscopy. Although they found that MRI and clinical examination had excellent diagnostic accuracy of 92.7% and 95.3%, respectively, the most common missed pathology on MRI later found on diagnostic arthroscopy was the presence of a lateral discoid meniscus, which occurred in 26.7% of missed diagnoses. Adult diagnostic criteria of discoid meniscus include ≥3 contiguous 5-mm sagittal cuts showing continuity between the anterior and posterior horns of the meniscus. Other criteria include a minimal meniscal width >15 mm on the coronal view or a minimum meniscal width that is >20% of the width of the maximal tibial width.28 These criteria are often applied to children as well. Additionally, if >50% of the lateral joint space is covered by meniscal tissue, a diagnosis of discoid meniscus should be considered.6
Continue to: TREATMENT
TREATMENT
Management of symptomatic discoid meniscus is directed toward resolving symptoms while preserving meniscal tissue and preventing the development of OA. Incidentally found discoid menisci in asymptomatic patients should not be treated surgically and can have periodic follow-ups for detection of any functional deterioration or symptom development. Surgical treatment may be beneficial for patients with symptoms related to their discoid menisci such as pain, recurrent effusion, limited knee motion, mechanical symptoms (e.g., locking and catching), and activity restrictions.
Traditionally, surgical treatment of a symptomatic discoid meniscus consisted of subtotal (meaning nearly complete, or <3mm of peripheral rim remaining) or complete meniscectomy, often performed in an open fashion. Surgical techniques have evolved; current surgical approaches typically include diagnostic arthroscopy followed by arthroscopic saucerization (also called partial meniscectomy) of the central portion of the “disc,” leaving a peripheral rim of 6 mm to 8 mm to approximate that of the normal meniscus (Figure 2).29,30 Saucerization removes the redundant central meniscal tissue in an attempt to create a more “normal” C-shaped morphology, although it does not address the increased thickness characteristic of many discoid menisci. It may be particularly difficult to “debulk” an abnormally thick discoid meniscus in the coronal plane, and there is little in the outcomes literature to support this approach. Following partial meniscectomy, the remaining meniscal tissue should be inspected thoroughly both for the presence of instability and for residual tears. Meniscal tears should be repaired in standard fashion; commonly, this may be a combination of all-inside sutures placed into the posterior horn, inside-out sutures placed into the midbody, and outside-in sutures placed into the anterior horn. Peripheral rim instability—identified, for example, by the ability to translate the posterior horn of the meniscus fully onto the anterior tibial plateau—should be addressed by suturing the meniscus to the adjacent capsule (Figure 2).25, 31 Menisci that have residual complex tears that are not amenable to repair may be treated by subtotal meniscectomy. These patients may be candidates for subsequent meniscal allograft transplantation.32,33
TREATMENT OUTCOMES
Performing a partial meniscectomy has consistently been shown in the laboratory to increase contact stresses proportionally to the percentage of tissue removed.34 It follows logically that performing a subtotal or complete meniscectomy in a young patient would yield increased, abnormal contact stresses throughout the knee with resultant degenerative changes and progression to OA over time. While long-term outcome studies of subtotal or complete meniscectomy have shown somewhat variable results, the majority report the development of pain, instability, poor function, osteoarthritic changes and even the development of osteochondritis desiccans.5,35
In an early investigation of long-term outcomes associated with surgical resection of a discoid lateral meniscus, Räber and colleagues36 retrospectively examined 17 knees that had undergone total meniscectomy for the lateral discoid meniscus. At a mean follow-up of 19.8 years, these authors reported that patients accounting for 10 of 17 operative knees had developed symptoms of osteoarthrosis such as pain. In addition, two-thirds of the knees that had follow-up radiographs performed (10 of 15 knees) had visible osteoarthritic changes present.
In 2011, Stilli and colleagues37 conducted a mid-term follow-up study examining 104 knees over an average follow-up of 8.5 years, with an average age of 8 years at the time of surgery. Younger patients, 2 to 7 years, underwent subtotal meniscectomy whereas patients, 8 to 14 years, underwent arthroscopic partial meniscectomy. Patients with a Wrisberg variant underwent removal of the posterior horn. The authors of this study reported that younger patients who had undergone subtotal meniscectomy had the best outcomes (defined by self-reported questionnaire responses and clinical evaluation). However, these results should be interpreted with caution: at the time of follow-up, patients in this study would still have been in adolescence and may not yet have developed the symptomatic degenerative changes in the knee joint that are strongly associated with meniscectomy.
Continue to: There are a few long-term...
There are few long-term outcome studies for arthroscopic saucerization. In 2014, Ahn and colleagues38 examined patient-reported outcomes and radiographic changes for 48 knees in pediatric patients treated arthroscopically for a symptomatic discoid meniscus. Patients were treated with partial meniscectomy (22 knees), partial meniscectomy with meniscal repair (18 knees), or subtotal meniscectomy (8 knees). Out of 48 knees evaluated, 45 knees (94%) were rated as “excellent” or “good” at an average 10-year follow-up. However, a significant percentage of patients had evidence of degenerative changes on follow-up radiographs, specifically: 88% of the subtotal meniscectomy group, 39% of the partial meniscectomy with repair group, and 23% in the partial meniscectomy-alone group. This finding suggests that the early appearance of radiographic changes suggestive of OA does not necessarily correlate with the development of knee symptoms in this cohort, although longer-term follow-up of these patients into adulthood and middle age is clearly needed.
Recently, short-term outcomes have been reported for contemporary arthroscopic saucerization of discoid menisci with peripheral rim stabilization performed as deemed necessary at the time of surgery. In 2012, Carter and colleagues39 examined 57 knees that underwent saucerization alone or saucerization with peripheral rim stabilization. At an average follow-up of 15 months, both patient populations had equivalent self-reported outcomes and clinical examination findings. The authors concluded that peripheral stabilization does not negatively affect short-term outcomes if instability is recognized and treated.
Yoo and colleagues40 found equivalent results between patients undergoing saucerization, saucerization with rim stabilization, and subtotal meniscectomy for 100 pediatric knees with an average follow-up of 4.7 years. Subtotal meniscectomy was defined as <3 mm of peripheral rim remaining and was performed when there was severe degeneration or complex tearing of the remnant rim following arthroscopic saucerization. Taken together, these data seem to suggest that short- and mid-term outcomes for pediatric patients treated surgically are generally good in terms of reported clinical function and development of OA, although long-term follow-up studies will be essential for understanding the true impact of surgical interventions.
Shieh and colleagues41,42 investigated risk factors for failed meniscal surgery in 324 menisci from 2008 to 2012, including in 46 discoid saucerization procedures with or without stabilization. At a mean of 40 months, 15% of the discoid saucerization cohort required a revision procedure, with increased odds of revision surgeries in patients who had undergone meniscal tear repair. The most frequent indication of revision surgery was sustaining a meniscal tear during intense physical activity in the first year after the index procedure, and patients underwent either debridement or repair for their revision procedure.
SUMMARY
Discoid meniscus is a rare anatomical meniscal variant characterized by an abnormal “O-like” shape, increased meniscal thickness, disorganization of collagen fibers, and variable absence of peripheral attachments. These morphological changes predispose patients with discoid menisci to increased rates of symptomatic meniscal instability and tearing. An MRI remains the most sensitive imaging modality for the diagnosis of a discoid meniscus, with ≥3 contiguous 5-mm sagittal cuts showing continuity between the anterior and posterior horns indicative of a discoid variant. Surgical treatment for symptomatic discoid menisci has evolved from subtotal meniscectomy to contemporary arthroscopic saucerization techniques with repair of meniscal tears and peripheral stabilization performed as needed. Long-term outcome studies for patients who undergo subtotal or complete meniscectomy reveal an association with osteoarthritic changes of the knee. Short- and mid-term outcome studies for patients who undergo arthroscopic saucerization with or without repair and/or peripheral stabilization are generally good, although reoperation rates have been estimated at 15% in the first 3 to 4 years. Longer-term follow-up, with the inclusion of validated functional outcomes measures, will be essential for understanding the true impact of various surgical interventions over time.
ABSTRACT
Discoid meniscus is a rare anatomical variant with altered morphology and structure that can sometimes present symptomatically, typically in the pediatric population. The discoid meniscus is usually in the lateral compartment of the knee and is characterized by a partial or complete filling-in of central meniscal tissue, increased meniscal thickness, disorganization of longitudinal collagen fibers, and sometimes lack of peripheral attachments. These changes to both the macro- and micro-structure of the meniscus predispose affected patients to increased rates of both meniscal tears and mechanical symptoms. Surgical management of symptomatic discoid meniscus is directed toward symptom resolution while preserving sufficient functional meniscal tissue to delay or prevent the development of osteoarthritis. Modern surgical techniques consist of arthroscopic saucerization of the discoid meniscus with repair of associated meniscal tears and stabilization of peripheral attachments. Although long-term outcome data are lacking, short- and mid-term outcomes for patients treated with arthroscopic partial meniscectomy and meniscal repair and/or stabilization as needed are generally good.
Continue to: The discoid meniscus...
The discoid meniscus is an uncommon anatomical meniscal variant that may present with pain, snapping, motion loss, swelling, and locking of the affected knee, typically during childhood or adolescence.1 Although the etiology of discoid meniscus is not completely understood, it is considered a congenital anomaly with a possible genetic component.2, 3 Incidence is estimated at 0.4% to 5.2% in the Western European population but is reportedly much higher (roughly 17%) in Asian populations.4-6, Discoid menisci, when present, are almost always in the lateral compartment, although cases of medial discoid meniscus have also been reported. The overall incidence of medial discoid meniscus has been estimated to be between 0.06% and 0.3% while that of lateral discoid meniscus, as detailed above, is significantly higher.7-11
ANATOMY AND MORPHOLOGY
The menisci differentiate from mesenchymal tissue early during fetal development with a clear definition by 8 weeks and a mature anatomical shape by 14 weeks in utero.12 Interestingly, menisci never possess a discoid shape during the normal course of development.13,14 The meniscus is fully vascularized at birth, with the central one-third becoming avascular by 9 months as the vascular supply regresses.14 By 10 years, only the peripheral one-third maintains its vascular supply, and the menisci have adult-like characteristics with tissue composed mainly of circumferential collagen fibers.4,14-16
A normal meniscus is wedge-shaped in the coronal plane and crescent-shaped in the axial plane. The medial meniscus is shaped like the letter “C,” is connected firmly to the joint capsule, and covers approximately 50% of the medial tibial plateau. The normal lateral meniscus covers 70% of the lateral tibial plateau and has firm anterior and posterior attachments while the lateral portion is less securely tethered.17 A discoid meniscus is characterized by an atypical shape—the center being partially or completely filled in (or “disc-like”)—often associated with anomalous attachments to the tibia, femur, and surrounding joint capsule. In addition to an atypical morphology, the discoid meniscus may also be much thicker than normal, resulting in a “block-like” appearance. The increased thickness may be responsible for the pathognomonic snapping of the knee as it is brought passively into flexion or extension (hence, the term “snapping knee” was traditionally used to describe a symptomatic discoid lateral meniscus). While the discoid shape is relatively straightforward to address, the increased meniscal thickness may be quite difficult to correct surgically.4-6,18
In addition to the macromorphological differences characteristic of discoid menisci, histological differences have also been described, including differences in collagen density and disorganization of the circumferential collagen network.19-21 Taken together, these differences may compromise the ability of the discoid meniscus to withstand normal forces placed across the knee and predispose it to tear. This is important because the normal meniscus plays a critical role in facilitating load distribution across the knee joint, in addition to assisting with functions including shock absorption, proprioception, and stabilization of the knee. Torn and/or unstable discoid menisci are unable to perform these functions adequately, which may ultimately result in degenerative joint disease and progress to end-stage osteoarthritis (OA).
CLASSIFICATION
The traditional Watanabe classification of discoid meniscus consists of 3 variants: type I, a complete discoid shape; type II, an incomplete discoid shape; and type III, the Wrisberg variant.22 The Wrisberg variant is described as a more normal-appearing meniscus but lacks normal peripheral attachments.5, 6, 15 This classification system was expanded by Monllau and colleagues, 23 who described a ring-type meniscus variant with normal posterior tibial attachments. Although the Watanabe classification is commonly used, its utility in assisting with clinical decision-making may be limited.
Continue to: Several other classification schemes...
Several other classification schemes exist. Jordan and colleagues24 described a classification scheme defining a meniscal type as complete or incomplete, also noting the presence of symptoms, tearing, and peripheral rim instability. They grouped stable types together, regardless of morphology, and then further classified them based on the presence of symptoms and tears. Similarly, the unstable types were grouped together and then subclassified in the same manner.17,24 Klingele and colleagues25 also described a contemporary classification scheme of discoid meniscus evaluating peripheral stability patterns that may be more clinically and surgically relevant. This classification is based on the type of discoid morphology (complete vs incomplete), the presence or absence of peripheral rim stability (stable vs unstable), and the presence or absence of a meniscal tear (torn vs untorn).5,25
EVALUATION
A stable discoid meniscus is often an incidental finding, seen either on advanced diagnostic imaging performed for another reason or at the time of arthroscopy to address another problem. Younger children with discoid meniscus tend to present with symptoms such as popping and snapping related to instability and the abnormal morphology of the discoid meniscus. Older patients tend to present with symptoms related to acute tears through the abnormal meniscal tissue. Although discoid menisci can become acutely symptomatic in the presence of a tear, the onset of symptoms may occur in the absence of a discrete traumatic event.1 Alternatively, some patients will report a clear history of injury, often a noncontact, rotational injury mechanism related to an athletic activity. Patients with torn discoid menisci may report pain, catching, locking, and/or giving way of the knee, and on examination may have limited extension, snapping, effusion, quadriceps atrophy, and joint line tenderness. Eponymous meniscal compression tests including the McMurray, Apley, and Thessaly tests, may also be performed when meniscal tear is suspected, although this may be tricky for younger children.1
Considering the high association of meniscal tears with ligamentous injuries, examination of knee stability is important. Plain radiographs of the knee should be taken, although the results will often be negative for osseous injury in the case of an isolated meniscal tear. Radiographs of a discoid knee may show subtle differences compared with radiographs of a non-discoid knee. A recent comparison of children with symptomatic lateral discoid menisci with age-matched controls found statistically significant increased lateral joint space, elevated fibular head, increased height of the lateral tibial spine, and increased obliquity of the tibial plateau.26 They did not find statistically significant increased squaring of the lateral femoral condyle or cupping of the lateral tibial plateau. Radiographic signs can be subtle and may not all be present in a patient with a discoid meniscus.
Magnetic resonance imaging (MRI) is the assessment technique of choice for the diagnosis of discoid meniscus, although MRI may not reliably identify a Wrisberg variant or incomplete discoid menisci (Figure 1).
Gans and colleagues27 examined preoperative MRI and clinical examination compared with pathology found during arthroscopy. Although they found that MRI and clinical examination had excellent diagnostic accuracy of 92.7% and 95.3%, respectively, the most common missed pathology on MRI later found on diagnostic arthroscopy was the presence of a lateral discoid meniscus, which occurred in 26.7% of missed diagnoses. Adult diagnostic criteria of discoid meniscus include ≥3 contiguous 5-mm sagittal cuts showing continuity between the anterior and posterior horns of the meniscus. Other criteria include a minimal meniscal width >15 mm on the coronal view or a minimum meniscal width that is >20% of the width of the maximal tibial width.28 These criteria are often applied to children as well. Additionally, if >50% of the lateral joint space is covered by meniscal tissue, a diagnosis of discoid meniscus should be considered.6
Continue to: TREATMENT
TREATMENT
Management of symptomatic discoid meniscus is directed toward resolving symptoms while preserving meniscal tissue and preventing the development of OA. Incidentally found discoid menisci in asymptomatic patients should not be treated surgically and can have periodic follow-ups for detection of any functional deterioration or symptom development. Surgical treatment may be beneficial for patients with symptoms related to their discoid menisci such as pain, recurrent effusion, limited knee motion, mechanical symptoms (e.g., locking and catching), and activity restrictions.
Traditionally, surgical treatment of a symptomatic discoid meniscus consisted of subtotal (meaning nearly complete, or <3mm of peripheral rim remaining) or complete meniscectomy, often performed in an open fashion. Surgical techniques have evolved; current surgical approaches typically include diagnostic arthroscopy followed by arthroscopic saucerization (also called partial meniscectomy) of the central portion of the “disc,” leaving a peripheral rim of 6 mm to 8 mm to approximate that of the normal meniscus (Figure 2).29,30 Saucerization removes the redundant central meniscal tissue in an attempt to create a more “normal” C-shaped morphology, although it does not address the increased thickness characteristic of many discoid menisci. It may be particularly difficult to “debulk” an abnormally thick discoid meniscus in the coronal plane, and there is little in the outcomes literature to support this approach. Following partial meniscectomy, the remaining meniscal tissue should be inspected thoroughly both for the presence of instability and for residual tears. Meniscal tears should be repaired in standard fashion; commonly, this may be a combination of all-inside sutures placed into the posterior horn, inside-out sutures placed into the midbody, and outside-in sutures placed into the anterior horn. Peripheral rim instability—identified, for example, by the ability to translate the posterior horn of the meniscus fully onto the anterior tibial plateau—should be addressed by suturing the meniscus to the adjacent capsule (Figure 2).25, 31 Menisci that have residual complex tears that are not amenable to repair may be treated by subtotal meniscectomy. These patients may be candidates for subsequent meniscal allograft transplantation.32,33
TREATMENT OUTCOMES
Performing a partial meniscectomy has consistently been shown in the laboratory to increase contact stresses proportionally to the percentage of tissue removed.34 It follows logically that performing a subtotal or complete meniscectomy in a young patient would yield increased, abnormal contact stresses throughout the knee with resultant degenerative changes and progression to OA over time. While long-term outcome studies of subtotal or complete meniscectomy have shown somewhat variable results, the majority report the development of pain, instability, poor function, osteoarthritic changes and even the development of osteochondritis desiccans.5,35
In an early investigation of long-term outcomes associated with surgical resection of a discoid lateral meniscus, Räber and colleagues36 retrospectively examined 17 knees that had undergone total meniscectomy for the lateral discoid meniscus. At a mean follow-up of 19.8 years, these authors reported that patients accounting for 10 of 17 operative knees had developed symptoms of osteoarthrosis such as pain. In addition, two-thirds of the knees that had follow-up radiographs performed (10 of 15 knees) had visible osteoarthritic changes present.
In 2011, Stilli and colleagues37 conducted a mid-term follow-up study examining 104 knees over an average follow-up of 8.5 years, with an average age of 8 years at the time of surgery. Younger patients, 2 to 7 years, underwent subtotal meniscectomy whereas patients, 8 to 14 years, underwent arthroscopic partial meniscectomy. Patients with a Wrisberg variant underwent removal of the posterior horn. The authors of this study reported that younger patients who had undergone subtotal meniscectomy had the best outcomes (defined by self-reported questionnaire responses and clinical evaluation). However, these results should be interpreted with caution: at the time of follow-up, patients in this study would still have been in adolescence and may not yet have developed the symptomatic degenerative changes in the knee joint that are strongly associated with meniscectomy.
Continue to: There are a few long-term...
There are few long-term outcome studies for arthroscopic saucerization. In 2014, Ahn and colleagues38 examined patient-reported outcomes and radiographic changes for 48 knees in pediatric patients treated arthroscopically for a symptomatic discoid meniscus. Patients were treated with partial meniscectomy (22 knees), partial meniscectomy with meniscal repair (18 knees), or subtotal meniscectomy (8 knees). Out of 48 knees evaluated, 45 knees (94%) were rated as “excellent” or “good” at an average 10-year follow-up. However, a significant percentage of patients had evidence of degenerative changes on follow-up radiographs, specifically: 88% of the subtotal meniscectomy group, 39% of the partial meniscectomy with repair group, and 23% in the partial meniscectomy-alone group. This finding suggests that the early appearance of radiographic changes suggestive of OA does not necessarily correlate with the development of knee symptoms in this cohort, although longer-term follow-up of these patients into adulthood and middle age is clearly needed.
Recently, short-term outcomes have been reported for contemporary arthroscopic saucerization of discoid menisci with peripheral rim stabilization performed as deemed necessary at the time of surgery. In 2012, Carter and colleagues39 examined 57 knees that underwent saucerization alone or saucerization with peripheral rim stabilization. At an average follow-up of 15 months, both patient populations had equivalent self-reported outcomes and clinical examination findings. The authors concluded that peripheral stabilization does not negatively affect short-term outcomes if instability is recognized and treated.
Yoo and colleagues40 found equivalent results between patients undergoing saucerization, saucerization with rim stabilization, and subtotal meniscectomy for 100 pediatric knees with an average follow-up of 4.7 years. Subtotal meniscectomy was defined as <3 mm of peripheral rim remaining and was performed when there was severe degeneration or complex tearing of the remnant rim following arthroscopic saucerization. Taken together, these data seem to suggest that short- and mid-term outcomes for pediatric patients treated surgically are generally good in terms of reported clinical function and development of OA, although long-term follow-up studies will be essential for understanding the true impact of surgical interventions.
Shieh and colleagues41,42 investigated risk factors for failed meniscal surgery in 324 menisci from 2008 to 2012, including in 46 discoid saucerization procedures with or without stabilization. At a mean of 40 months, 15% of the discoid saucerization cohort required a revision procedure, with increased odds of revision surgeries in patients who had undergone meniscal tear repair. The most frequent indication of revision surgery was sustaining a meniscal tear during intense physical activity in the first year after the index procedure, and patients underwent either debridement or repair for their revision procedure.
SUMMARY
Discoid meniscus is a rare anatomical meniscal variant characterized by an abnormal “O-like” shape, increased meniscal thickness, disorganization of collagen fibers, and variable absence of peripheral attachments. These morphological changes predispose patients with discoid menisci to increased rates of symptomatic meniscal instability and tearing. An MRI remains the most sensitive imaging modality for the diagnosis of a discoid meniscus, with ≥3 contiguous 5-mm sagittal cuts showing continuity between the anterior and posterior horns indicative of a discoid variant. Surgical treatment for symptomatic discoid menisci has evolved from subtotal meniscectomy to contemporary arthroscopic saucerization techniques with repair of meniscal tears and peripheral stabilization performed as needed. Long-term outcome studies for patients who undergo subtotal or complete meniscectomy reveal an association with osteoarthritic changes of the knee. Short- and mid-term outcome studies for patients who undergo arthroscopic saucerization with or without repair and/or peripheral stabilization are generally good, although reoperation rates have been estimated at 15% in the first 3 to 4 years. Longer-term follow-up, with the inclusion of validated functional outcomes measures, will be essential for understanding the true impact of various surgical interventions over time.
1. Yaniv M, Blumberg N. The discoid meniscus. J Child Orthop. 2007;1(2):89-96. doi: 10.1007/s11832-007-0029-1.
2. Gebhardt M, Rosenthal R. Bilateral lateral discoid meniscus in identical twins. J Bone Joint Surg Am. 1979;61(7):1110-1111. doi: 10.2106/00004623-197961070-00027.
3. Dashefsky JH. Discoid lateral meniscus in three members of a family. J Bone Joint Surg, (Am.). 1971;53(6):1208-1210. doi: 10.2106/00004623-197153060-00018.
4. Francavilla ML, Restrepo R, Zamora KW, Sarode V, Swirsky SM, Mintz D. Meniscal pathology in children: differences and similarities with the adult meniscus. Pediatr Radiol. 2014;44(8):910-925. doi: 10.1007/s00247-014-3022-0.
5. Kushare I, Klingele K, Samora W. Discoid meniscus: diagnosis and management. Orthop Clin North Am. 2015;46(4):533-540. doi: 10.1016/j.ocl.2015.06.007.
6. McKay S, Chen C, Rosenfeld S. Orthopedic perspective on selected pediatric and adolescent knee conditions. Pediatr Radiol. 2013;43(1):99-106. doi: 10.1007/s00247-012-2587-8.
7. Greis PE, Bardana DD, Holmstrom MC, Burks RT. Meniscal injury: I. Basic science and evaluation. J Am Acad Orthop Surg. 2002;10(3):168-176. doi: 10.5435/00124635-200205000-00003.
8. Ikeuchi H. Arthroscopic treatment of the discoid lateral meniscus. Technique and long-term results. Clin Orthop Relat Res. 1982;167(167):19-28.
9. Nathan PA, Cole SC. 12 Discoid meniscus: a clinical and pathologic study. Clin Orthop Relat Res: SC: Cole. 1969;64:107-113.
10. Jeannopoulos CL. Observations on discoid menisci. J Bone Joint Surg, (Am.). 1950;32(3):649-652. doi: 10.2106/00004623-195032030-00019.
11. Dickason J, Del WP, Blazina ME, Fox JM, Friedman MJ, Snyder SJ. A series of ten discoid medial menisci. Clin Orthop Relat Res. 1982;168:75-79. doi: 10.1097/00003086-198208000-00014
12. Andrish JT. Meniscal injuries in children and adolescents: diagnosis and management. J Am Acad Orthop Surg. 1996;4(5):231-237. doi: 10.5435/00124635-199609000-00001.
13. Kaplan E. The embryology of the menisci of the knee joint. Bull Hosp Joint Dis. 1955;16(2):111-124.
14. Clark C, Ogden J. Development of the menisci of the human knee joint. Morphological changes and their potential role in childhood meniscal injury. J Bone Joint Surg, (Am.). 1983;65(4):538-547 doi: 10.2106/00004623-198365040-00018.
15. Carter CW, Kocher MS. Meniscus repair in children. Clin Sports Med. 2012;31(1):135-154. doi: 10.1016/j.csm.2011.09.002.
16. Shieh A, Bastrom T, Roocroft J, Edmonds EW, Pennock AT. Meniscus tear patterns in relation to skeletal immaturity: children versus adolescents. Am J Sports Med. 2013;41(12):2779-2783. doi: 10.1177/0363546513504286.
17. Jordan MR. Lateral meniscal variants: evaluation and treatment. J Am Acad Orthopsurg. 1996;4(4):191-200. doi: 10.5435/00124635-199607000-00003.
18. Flouzat-Lachaniette C, Pujol N, Boisrenoult P, Beaufils P. Discoid medial meniscus: report of four cases and literature review. Orthop Traumatol Surg Res. 2011;97(8):826-832. doi: 10.1016/j.otsr.2011.07.011.
19. Choi Y-H, Seo Y-J, Ha JM, Jung KH, Kim J, Song SY. Collagenous ultrastructure of the discoid meniscus: A Transmission Electron Microscopy Study. Am J Sports Med. 2017;45(3):598-603. doi: 10.1177/0363546516674181.
20. Atay OA, Pekmezci M, Doral MN, Sargon MF, Ayvaz M, Johnson DL. Discoid meniscus: an ultrastructural study with transmission electron microscopy. Am J Sports Med. 2007;35(3):475-478. doi: 10.1177/0363546506294678.
21. Papadopoulos A, Kirkos JM, Kapetanos GA. Histomorphologic study of discoid meniscus. Arthroscopy. 2009;25(3):262-268. doi: 10.1016/j.arthro.2008.10.006.
22. Watanabe M, Takeda S, Ikeuchi H. Atlas of Arthroscopy. Tokyo: Igaku-Shoin Ltd; 1969.
23. Monllau JC, León A, Cugat R, Ballester J. Ring-shaped lateral meniscus. Arthroscopy. 1998;14(5):502-504. doi: 10.1016/S0749-8063(98)70079-9.
24. Jordan M, Duncan J, Bertrand S. Discoid lateral meniscus: a review. S Orthop J. 1993;2(4):239-253.
25. Klingele KE, Kocher MS, Hresko MT, Gerbino P, Micheli LJ. Discoid lateral meniscus: prevalence of peripheral rim instability. J Pediatr Orthop. 2004;24(1):79-82. doi: 10.1097/01241398-200401000-00015.
26. Choi SH, Ahn JH, Kim KI, et al. Do the radiographic findings of symptomatic discoid lateral meniscus in children differ from normal control subjects? Knee Surg Sports Traumatol Arthrosc. 2015;23(4):1128-1134. doi: 10.1007/s00167-014-2924-6.
27. Gans I, Bedoya MA, Ho-Fung V, Ganley TJ. Diagnostic performance of magnetic resonance imaging and pre-surgical evaluation in the assessment of traumatic intra-articular knee disorders in children and adolescents: what conditions still pose diagnostic challenges? Pediatr Radiol. 2015;45(2):194-202. doi: 10.1007/s00247-014-3127-5.
28. Samoto N, Kozuma M, Tokuhisa T, Kobayashi K. Diagnosis of discoid lateral meniscus of the knee on MR imaging. Magn Reson Imaging. 2002;20(1):59-64. doi: 10.1016/S0730-725X(02)00473-3.
29. Hayashi LK, Yamaga H, Ida K, Miura T. Arthroscopic meniscectomy for discoid lateral meniscus in children. J Bone Joint Surg, (Am.). 1988;70(10):1495-1500. doi: 10.2106/00004623-198870100-00009.
30. Kim S-J, Kim D-W, Min B-H. Discoid lateral meniscus associated with anomalous insertion of the medial meniscus. Clin Orthop Rel Res. 1995;315(315):234-237 doi: 10.1097/00003086-199506000-00026.
31. Adachi N, Ochi M, Uchio Y, Kuriwaka M, Shinomiya R. Torn discoid lateral meniscus treated using partial central meniscectomy and suture of the peripheral tear. Arthroscopy. 2004;20(5):536-542. doi: 10.1016/j.arthro.2004.01.028.
32. Kim J-M, Bin S-I. Meniscal allograft transplantation after total meniscectomy of torn discoid lateral meniscus. Arthroscopy. 2006;22(12):1344-1350.e1. doi: 10.1016/j.arthro.2006.07.048.
33. Ramme AJ, Strauss EJ, Jazrawi L, Gold HT. Cost effectiveness of meniscal allograft for torn discoid lateral meniscus in young women. Phys Sportsmed. 2016;44(3):278-282. doi: 10.1080/00913847.2016.1197762.
34. Baratz ME, Fu FH, Mengato R. Meniscal tears: the effect of meniscectomy and of repair on intraarticular contact areas and stress in the human knee: a preliminary report. Am J Sports Med. 1986;14(4):270-275. doi: 10.1177/036354658601400405.
35. Wroble RR, Henderson RC, Campion ER, el-Khoury GY, Albright JP. Meniscectomy in children and adolescents. A long-term follow-up study. Clin Orthop Relat Res. 1992;279(279):180-189.
36. Räber D, Friederich N, Hefti F. Discoid lateral meniscus in children. Long-term follow-up after total meniscectomy. J Bone Joint Surg, (Am.). 1998;80(11):1579-1586. doi: 10.2106/00004623-199811000-00003.
37. Stilli S, Reggiani LM, Muccioli GMM, Cappella M, Donzelli O. Arthroscopic treatment for symptomatic discoid lateral meniscus during childhood. Knee Surg Sports Traumatol Arthrosc. 2011;19(8):1337-1342. doi: 10.1007/s00167-011-1440-1.
38. Ahn JH, Kim KI, Wang JH, Jeon JW, Cho YC, Lee SH. Long-term results of arthroscopic reshaping for symptomatic discoid lateral meniscus in children. Arthroscopy. 2015;31(5):867-873. doi: 10.1016/j.arthro.2014.12.012.
39. Carter CW, Hoellwarth J, Weiss JM. Clinical outcomes as a function of meniscal stability in the discoid meniscus: a preliminary report. J Pediatr Orthop. 2012;32(1):9-14. doi: 10.1097/BPO.0b013e31823d8338.
40. Yoo WJ, Jang WY, Park MS, et al. Arthroscopic treatment for symptomatic discoid meniscus in children: midterm outcomes and prognostic factors. Arthroscopy. 2015;31(12):2327-2334. doi: 10.1016/j.arthro.2015.06.032.
41. Shieh A, Edmonds EW, Pennock AT. Revision meniscus surgery in children and adolescents: the effect of skeletal immaturity. Orthop J Sports Med. 2015;3(7):S00075:2325967115. doi: 10.1177/2325967115S00075.
42. Shieh AK, Edmonds EW, Pennock AT. Revision meniscal surgery in children and adolescents: risk factors and mechanisms for failure and subsequent management. Am J Sports Med. 2016;44(4):838-843. doi: 10.1177/0363546515623511.
1. Yaniv M, Blumberg N. The discoid meniscus. J Child Orthop. 2007;1(2):89-96. doi: 10.1007/s11832-007-0029-1.
2. Gebhardt M, Rosenthal R. Bilateral lateral discoid meniscus in identical twins. J Bone Joint Surg Am. 1979;61(7):1110-1111. doi: 10.2106/00004623-197961070-00027.
3. Dashefsky JH. Discoid lateral meniscus in three members of a family. J Bone Joint Surg, (Am.). 1971;53(6):1208-1210. doi: 10.2106/00004623-197153060-00018.
4. Francavilla ML, Restrepo R, Zamora KW, Sarode V, Swirsky SM, Mintz D. Meniscal pathology in children: differences and similarities with the adult meniscus. Pediatr Radiol. 2014;44(8):910-925. doi: 10.1007/s00247-014-3022-0.
5. Kushare I, Klingele K, Samora W. Discoid meniscus: diagnosis and management. Orthop Clin North Am. 2015;46(4):533-540. doi: 10.1016/j.ocl.2015.06.007.
6. McKay S, Chen C, Rosenfeld S. Orthopedic perspective on selected pediatric and adolescent knee conditions. Pediatr Radiol. 2013;43(1):99-106. doi: 10.1007/s00247-012-2587-8.
7. Greis PE, Bardana DD, Holmstrom MC, Burks RT. Meniscal injury: I. Basic science and evaluation. J Am Acad Orthop Surg. 2002;10(3):168-176. doi: 10.5435/00124635-200205000-00003.
8. Ikeuchi H. Arthroscopic treatment of the discoid lateral meniscus. Technique and long-term results. Clin Orthop Relat Res. 1982;167(167):19-28.
9. Nathan PA, Cole SC. 12 Discoid meniscus: a clinical and pathologic study. Clin Orthop Relat Res: SC: Cole. 1969;64:107-113.
10. Jeannopoulos CL. Observations on discoid menisci. J Bone Joint Surg, (Am.). 1950;32(3):649-652. doi: 10.2106/00004623-195032030-00019.
11. Dickason J, Del WP, Blazina ME, Fox JM, Friedman MJ, Snyder SJ. A series of ten discoid medial menisci. Clin Orthop Relat Res. 1982;168:75-79. doi: 10.1097/00003086-198208000-00014
12. Andrish JT. Meniscal injuries in children and adolescents: diagnosis and management. J Am Acad Orthop Surg. 1996;4(5):231-237. doi: 10.5435/00124635-199609000-00001.
13. Kaplan E. The embryology of the menisci of the knee joint. Bull Hosp Joint Dis. 1955;16(2):111-124.
14. Clark C, Ogden J. Development of the menisci of the human knee joint. Morphological changes and their potential role in childhood meniscal injury. J Bone Joint Surg, (Am.). 1983;65(4):538-547 doi: 10.2106/00004623-198365040-00018.
15. Carter CW, Kocher MS. Meniscus repair in children. Clin Sports Med. 2012;31(1):135-154. doi: 10.1016/j.csm.2011.09.002.
16. Shieh A, Bastrom T, Roocroft J, Edmonds EW, Pennock AT. Meniscus tear patterns in relation to skeletal immaturity: children versus adolescents. Am J Sports Med. 2013;41(12):2779-2783. doi: 10.1177/0363546513504286.
17. Jordan MR. Lateral meniscal variants: evaluation and treatment. J Am Acad Orthopsurg. 1996;4(4):191-200. doi: 10.5435/00124635-199607000-00003.
18. Flouzat-Lachaniette C, Pujol N, Boisrenoult P, Beaufils P. Discoid medial meniscus: report of four cases and literature review. Orthop Traumatol Surg Res. 2011;97(8):826-832. doi: 10.1016/j.otsr.2011.07.011.
19. Choi Y-H, Seo Y-J, Ha JM, Jung KH, Kim J, Song SY. Collagenous ultrastructure of the discoid meniscus: A Transmission Electron Microscopy Study. Am J Sports Med. 2017;45(3):598-603. doi: 10.1177/0363546516674181.
20. Atay OA, Pekmezci M, Doral MN, Sargon MF, Ayvaz M, Johnson DL. Discoid meniscus: an ultrastructural study with transmission electron microscopy. Am J Sports Med. 2007;35(3):475-478. doi: 10.1177/0363546506294678.
21. Papadopoulos A, Kirkos JM, Kapetanos GA. Histomorphologic study of discoid meniscus. Arthroscopy. 2009;25(3):262-268. doi: 10.1016/j.arthro.2008.10.006.
22. Watanabe M, Takeda S, Ikeuchi H. Atlas of Arthroscopy. Tokyo: Igaku-Shoin Ltd; 1969.
23. Monllau JC, León A, Cugat R, Ballester J. Ring-shaped lateral meniscus. Arthroscopy. 1998;14(5):502-504. doi: 10.1016/S0749-8063(98)70079-9.
24. Jordan M, Duncan J, Bertrand S. Discoid lateral meniscus: a review. S Orthop J. 1993;2(4):239-253.
25. Klingele KE, Kocher MS, Hresko MT, Gerbino P, Micheli LJ. Discoid lateral meniscus: prevalence of peripheral rim instability. J Pediatr Orthop. 2004;24(1):79-82. doi: 10.1097/01241398-200401000-00015.
26. Choi SH, Ahn JH, Kim KI, et al. Do the radiographic findings of symptomatic discoid lateral meniscus in children differ from normal control subjects? Knee Surg Sports Traumatol Arthrosc. 2015;23(4):1128-1134. doi: 10.1007/s00167-014-2924-6.
27. Gans I, Bedoya MA, Ho-Fung V, Ganley TJ. Diagnostic performance of magnetic resonance imaging and pre-surgical evaluation in the assessment of traumatic intra-articular knee disorders in children and adolescents: what conditions still pose diagnostic challenges? Pediatr Radiol. 2015;45(2):194-202. doi: 10.1007/s00247-014-3127-5.
28. Samoto N, Kozuma M, Tokuhisa T, Kobayashi K. Diagnosis of discoid lateral meniscus of the knee on MR imaging. Magn Reson Imaging. 2002;20(1):59-64. doi: 10.1016/S0730-725X(02)00473-3.
29. Hayashi LK, Yamaga H, Ida K, Miura T. Arthroscopic meniscectomy for discoid lateral meniscus in children. J Bone Joint Surg, (Am.). 1988;70(10):1495-1500. doi: 10.2106/00004623-198870100-00009.
30. Kim S-J, Kim D-W, Min B-H. Discoid lateral meniscus associated with anomalous insertion of the medial meniscus. Clin Orthop Rel Res. 1995;315(315):234-237 doi: 10.1097/00003086-199506000-00026.
31. Adachi N, Ochi M, Uchio Y, Kuriwaka M, Shinomiya R. Torn discoid lateral meniscus treated using partial central meniscectomy and suture of the peripheral tear. Arthroscopy. 2004;20(5):536-542. doi: 10.1016/j.arthro.2004.01.028.
32. Kim J-M, Bin S-I. Meniscal allograft transplantation after total meniscectomy of torn discoid lateral meniscus. Arthroscopy. 2006;22(12):1344-1350.e1. doi: 10.1016/j.arthro.2006.07.048.
33. Ramme AJ, Strauss EJ, Jazrawi L, Gold HT. Cost effectiveness of meniscal allograft for torn discoid lateral meniscus in young women. Phys Sportsmed. 2016;44(3):278-282. doi: 10.1080/00913847.2016.1197762.
34. Baratz ME, Fu FH, Mengato R. Meniscal tears: the effect of meniscectomy and of repair on intraarticular contact areas and stress in the human knee: a preliminary report. Am J Sports Med. 1986;14(4):270-275. doi: 10.1177/036354658601400405.
35. Wroble RR, Henderson RC, Campion ER, el-Khoury GY, Albright JP. Meniscectomy in children and adolescents. A long-term follow-up study. Clin Orthop Relat Res. 1992;279(279):180-189.
36. Räber D, Friederich N, Hefti F. Discoid lateral meniscus in children. Long-term follow-up after total meniscectomy. J Bone Joint Surg, (Am.). 1998;80(11):1579-1586. doi: 10.2106/00004623-199811000-00003.
37. Stilli S, Reggiani LM, Muccioli GMM, Cappella M, Donzelli O. Arthroscopic treatment for symptomatic discoid lateral meniscus during childhood. Knee Surg Sports Traumatol Arthrosc. 2011;19(8):1337-1342. doi: 10.1007/s00167-011-1440-1.
38. Ahn JH, Kim KI, Wang JH, Jeon JW, Cho YC, Lee SH. Long-term results of arthroscopic reshaping for symptomatic discoid lateral meniscus in children. Arthroscopy. 2015;31(5):867-873. doi: 10.1016/j.arthro.2014.12.012.
39. Carter CW, Hoellwarth J, Weiss JM. Clinical outcomes as a function of meniscal stability in the discoid meniscus: a preliminary report. J Pediatr Orthop. 2012;32(1):9-14. doi: 10.1097/BPO.0b013e31823d8338.
40. Yoo WJ, Jang WY, Park MS, et al. Arthroscopic treatment for symptomatic discoid meniscus in children: midterm outcomes and prognostic factors. Arthroscopy. 2015;31(12):2327-2334. doi: 10.1016/j.arthro.2015.06.032.
41. Shieh A, Edmonds EW, Pennock AT. Revision meniscus surgery in children and adolescents: the effect of skeletal immaturity. Orthop J Sports Med. 2015;3(7):S00075:2325967115. doi: 10.1177/2325967115S00075.
42. Shieh AK, Edmonds EW, Pennock AT. Revision meniscal surgery in children and adolescents: risk factors and mechanisms for failure and subsequent management. Am J Sports Med. 2016;44(4):838-843. doi: 10.1177/0363546515623511.
TAKE-HOME POINTS
- The discoid meniscus is a congenital variant that is present from birth and may or not become symptomatic as a child matures.
- MRI may be used to make the diagnosis of discoid lateral meniscus, defined as 3 or more consecutive sagittal 5-mm cuts demonstrating contiguity of the anterior and posterior horns.
- A useful classification system for discoid meniscus describes the shape of the meniscus (complete or partial disc), whether it is torn (torn or intact), and whether it has peripheral instability (stable or unstable).
- Surgical treatment of symptomatic discoid lateral meniscus is aimed at restoring normal morphology and stability to the abnormal meniscus.
- Short- and mid-term outcomes following partial meniscectomy with repair and/or stabilization as needed are generally good; long-term outcomes following subtotal or complete meniscectomy are poor, demonstrating progression to early arthritis.
Return to Play After an Anterior Cruciate Ligament Injury: Prioritizing Neurological and Psychological Factors of the Decision-Making Algorithm
ABSTRACT
Soccer players recovering from anterior cruciate ligament (ACL) injuries have better options for treatment today than they did 25 years ago. Surgical techniques have improved, and rehabilitation protocols have evolved considerably. Although the rehabilitation community is doing a better job of treating this patient population, the evidence does demonstrate that both re-injury and return- to-play (RTP) rates are still suboptimal. Most protocols focus on normalizing strength and range of motion (ROM) and achieving limb symmetry with soccer-specific movements. While these factors are certainly prerequisites for returning to the field, their inclusion does not provide a complete picture of the athlete’s presentation. An additional factor that should be prioritized with this patient population is the central nervous system (CNS). Advanced imaging has shown that peripheral deafferentation does occur with musculoskeletal injuries; this ultimately results in cortical reorganization, which makes movement planning more difficult for the player, since simpler tasks must now be processed at higher levels in the CNS. The evidence also shows that the CNS demonstrates plasticity in these cases, so that through focused neuromotor rehabilitation techniques, it is possible to bring movement planning back down to a sub-cortical level. Cognitive issues may also be a factor in preventing the player from returning. Fear of re-injury and diminished confidence can influence the way the player moves on the field, and diminish ability to demonstrate protective kinematics with all soccer-specific tasks. We believe that an approach incorporating traditional musculoskeletal rehabilitation, CNS neuro-motor training, and consideration for cognitive factors, may define an improved paradigm for treating the soccer player and assessing readiness for RTP following ACL injury.
Continue to: Although anterior cruciate ligament (ACL)...
Although anterior cruciate ligament (ACL) rehabilitation has evolved considerably over the past 2 decades, the basic paradigm has remained consistent: normalize strength and range of motion, reduce swelling and pain, achieve limb symmetry with functional tasks, and return to sport-specific activities gradually over a 6 to 12-month period. There have been some slight additions to this basic premise, such as evaluating knee and hip mechanics in the frontal plane, but the requirements here are vaguely defined and are typically only evaluated within the context of controlled clinical testing.
It is interesting to note that the typical ACL injury pattern occurs during a normal sport-specific movement, yet most rehabilitation protocols fail to recognize the potential causes of the aberrant movement pattern and how to best modify it so that the risk of repeated stress to the ACL can be minimized. It should be understood that movement occurs through the interaction of 3 discrete factors: the individual, the task being performed, and the environment in which it is performed.1 All of these factors will play a role in how the final movement pattern is produced. For example, a soccer player (individual) may backpedal and pivot to the left 60° and accelerate to sprint after a player moving towards the touchline (task) while receiving instructions from teammates and monitoring the movements of opposing players (environment). A small variance in any 1 of these factors could significantly impact the movement pattern as the player completes the task.
In most rehabilitation programs, each of these factors may be treated in a singular, non-specific manner, but if these factors are not coordinated effectively throughout the program to produce the desired sport-specific movement, a faulty pattern may persist, leaving the player at risk for injury. Current rehabilitation programs seem to have a strong focus on creating stability, mobility, and strength, but these are trained in silos, with an internal focus of control, which only solves the biomechanical equation. Often, it is difficult for the player to coordinate good biomechanics into an efficient, protective movement pattern that is specific to the tasks performed on the field during the normal course of play. The missing link here is the central nervous system (CNS).
Limitations to the current ACL protocols may be that they rely heavily on musculoskeletal rehabilitation and that they have limited emphasis on neurological rehabilitation. As will be discussed later, the CNS has a large impact on the final movement selected by the player. In fact, cognition, perception, and action are the three factors that comprise the individual’s part of the movement paradigm,1 yet rarely are these factors addressed in most ACL rehabilitation programs. These elements are a large part of the movement equation, so it is easy to understand how failing to address these features can lead to poor movement quality and subsequent ACL re-injury.
In addition to central neurological factors, cognitive issues may play a role in the player’s ability to return to sport. Determining optimal readiness for return to play is a difficult task for the medical community, with many variables to consider. Previous research studies have assessed the variability in return to play for various sports, including football, rugby, soccer, skiing, running, and tennis, with return-to-play rates ranging from 18% to 100%.2,3,4-10 The risk of secondary injury may cast doubt and fear on athletes as they contemplate their successful return to play.8,11 Although robust functional testing has become commonplace for determining athlete readiness after injury,12-20 the assessment of psychological readiness, persistent fear, and loss of confidence are often neglected and not as commonly integrated into the return-to-play algorithm.21-24 The purpose of this paper is to assess the various cognitive and central neural factors affecting a soccer player’s ability to recover from an ACL injury and offer suggestions for integrating treatments into the protocols to address these issues.
Continue to: CENTRAL NERVOUS SYSTEM NEUROPLASTICITY...
CENTRAL NERVOUS SYSTEM NEUROPLASTICITY
Despite the vast amount of attention and research focused on the ACL, the re-injury rate still remains quite high. It has been reported that rehabilitation programs that employ traditional neuromotor training produce a re-injury rate as high as 30% after the athlete returns to sport.25-28 The overall rate of sustaining a second ACL injury is 15%11 in all patient populations. For the general population <25 years of age, the re-injury rate is 21%, and for athletes <25 years of age, the re-injury rate rises to 23%.11 With re-injury rates at this level, it is certainly fair to consider and be critical of the current rehabilitation methods being used with this population. One opportunity for improvement lies in the general approach used to rehabilitate ACL-injured patients. Therapy for this injury is protocol-driven, and the fact remains that most protocols prioritize restoration of peripheral systems, with minimal thought given to the cortical control necessary to manage those systems.29,30 When neural factors are considered, it is usually within the context of increasing strength, balance, power, and biomechanical control,31-34 which are certainly important but peripheral factors nonetheless. The missing element in many ACL protocols may be how to best manage the central neural components and cognitive factors associated with this injury.
If the CNS were to receive more consideration in ACL protocols, the opportunity for improved outcomes could be substantial because the CNS has been proven to be a very malleable system, as long as it receives the correct input. The CNS demonstrates neuroplasticity,35 which means that it is capable of reorganization, based on the stimuli that it receives, whether internal or external.36
This is an important consideration in ACL rehabilitation because the ACL graft, while restoring the biomechanical properties to the knee, is not fully capable of producing the same neurosensory properties of the original ACL.37-42This is an important concept to understand because an ACL tear does indeed cause deafferentation in the ascending pathways to the brain.37-40,42-46 This can lead to CNS reorganization and subsequent alterations in efferent output to the periphery.37-40,42-46 Therefore, if a protocol with traditional musculoskeletal principles was used, then the mechanical function of the knee may certainly be remediated, but the neurosensory function will remain in a maladaptive state,47-50 potentially leading to aberrant, non-protective movement strategies and a higher risk of re-injury.
The process of CNS reorganization may begin with the initial ACL injury. A peripheral musculoskeletal injury creates an inflammatory response that results in the arrival of chemical mediators such as histamine, substance P, calcitonin, and calcitonin gene-related peptide at the site of injury.51 As edema accumulates in the joint, tension is applied to the capsule, which may adversely affect proprioception from the receptors located within.45 The interruption of consistent input from the peripheral mechanoreceptors may lead to long-term differentiation of the ascending pathways.52 This information is synthesized at 3 different levels of the CNS (spinal cord, brain stem, and motor cortex) to produce motor output.53-56 Differentiation in the ascending circuitry can cause inhibition of motor neurons at the spinal cord.45Animal research has shown that this differentiation can cause a breakdown in the cuneate nucleus of the brainstem,57which provides sensory information from the upper body, while the gracile nucleus does the same for the lower body. These structures transfer proprioceptive input to the ventral posterior lateral nucleus in the thalamus, where it is then sent to the primary somatosensory cortex.57 In general, the somatosensory, visual, and vestibular systems interpret afferent inputs to control movement, balance, and stability.58,59 In a sport like soccer, where the movement tasks are dynamic and unpredictable, it is easy to see why even a slight deficit in somatosensory processing could disrupt a movement. Valeriani and colleagues42,46 showed that somatosensory-evoked potentials were indeed altered in a cohort of ACL reconstruction (ACLR) subjects, indicating reorganization within the CNS. Additionally, the deafferentation could not be changed by other afferent input coming from the knee or by the new ACL graft placed in the knee.42,46The primary motor cortex has been found to have a substantial network of connectivity with the primary somatosensory cortex, which supports the theory that the motor cortex has a very strong linkage with the peripheral receptors in the joint.60 The ligaments in the joint contain Ruffini, Pacinian, and Golgi receptors, all of which react to changes in the collagen fibers and send information regarding tension, length, speed, acceleration, position, and movement back to the CNS.61-64 Unfortunately, the ascending pathway deafferentation can cause reorganization within the CNS, which makes the feedback provided from the periphery less effective in motor planning.
Ward and colleagues65 have reported that reorganization within the motor cortex is the primary cause of chronic neuromuscular movement deficits in peripheral joint injuries. Researchers have used functional magnetic resonance imaging, transcranial magnetic stimulation, and electroencephalography in ACL patients to demonstrate changes in cortical activity and subsequent CNS reorganization.65 Kapreli and colleagues41 reported that subjects with an ACL injury demonstrated higher cortical activation in the pre-supplementary motor area (pre-SMA). This is a region that is responsible for more complex motor planning.66,67 This area becomes active before the primary motor cortex and is responsible for preparing the final movement pattern that the motor cortex executes.41 As the task becomes more complex, activity in the pre-SMA will increase.41 Additionally, they found that the posterior secondary somatosensory area and posterior inferior temporal gyrus showed increased cortical activity compared with controls.41 Visual planning is processed in the posterior inferior temporal gyrus, and so, it appears that the difficulty in processing somatosensory information due to ascending pathway deafferentation places an increased reliance on the visual system for movement planning.68-70 This was observed while ACL-injured subjects performed a simple knee flexion-extension movement encompassing 40°, indicating the need to incorporate higher central levels of planning for a very simple movement pattern.41 Baumeister and colleagues37,38 also showed that subjects with ACLR had higher levels of cortical activation in the areas of the brain that require attention and that process sensory input. They theorized that this occurred because of reduced efficiency of neural processing at lower levels in the CNS. Despite the higher levels of cortical activity observed, they found that subjects with an ACLR demonstrated proprioceptive testing that was deficient compared with that of controls. Heroux and Tremblay71 also demonstrated that subjects with an ACLR had increased resting motor cortex activity. They believed that this occurred as the motor cortex attempted to maintain neuromotor output to the periphery in the face of diminished afferent input.
Continue to: The reorganization that results in movement planning...
The reorganization that results in movement planning, transitioning from subcortical levels to cortical levels, is a phenomenon that researchers believe can lead to deficiencies even as the athlete has returned to sport. Grooms et al72revealed in a case report that a subject with an ACLR showed higher levels of activity in the crus region of the cerebellum. This area contains corticobulbar and corticospinal tracts that transmit neural input to maintain balance and coordination.73 These changes in the cerebellum, combined with increased motor cortex activity, are thought to be indicative of a global neural strategy that uses higher levels of the CNS, as opposed to subcortical processing.72
The current research makes a clear and compelling argument for the importance of CNS reorganization after an ACL injury, placing increased reliance on higher cortical levels of control, as well as the visual system to coordinate balance and movement. It is thought that this reorganized method of neural transmission can then become imprinted within the CNS, if not corrected.35,74 If this is the case, then traditional strength programs may not be sufficient to restore these connections to their pre-injury level. If the CNS has the ability to reorganize based on the aberrant input that it receives from the periphery, then it also certainly has the potential to adapt to more specific structured input via the ascending afferent pathways.41,45 The rehabilitation program, however, needs to be structured specifically to target the reorganized regions of the brain. There needs to be an emphasis on rehabilitating not only the peripheral neuromotor structures but also the CNS.75
CENTRAL NERVOUS SYSTEM REHABILITATION PRINCIPLES
For a neurological rehabilitation to be successful, the interventions need to be repetitive and task-specific, involve learning, employ whole and part practice, and transition from using an internal to an external focus of control.76Movements that are repetitive, but which lack structured learning and skill, have been shown to have no effect on inducing neuroplastic changes in the primary motor cortex.77,78 However, using neurological rehabilitation techniques that facilitate the acquisition of new motor skills by the CNS have been shown to cause neuroplastic adaptation in the motor cortex.79-85 This occurs because neuroplasticity is determined by experience and practice.78 The CNS operates on cues received in the ascending tracts by mechanoreceptors in the joint. If a new movement pattern is being learned by the athlete, then this new afferent input received from the periphery will start to initiate reorganization in the higher learning centers. If this occurs with optimal repetition and precision, then a positive reorganization can take place within the CNS that results in a higher percentage of motor planning and control being filtered down to a subcortical level. Essentially, the movements become instinctive, which is crucial in athletics, where attention in higher cortical areas is frequently diverted to external aspects of the competition and not solely used to focus on movement.
This is why shifting neurological rehabilitation from an internal focus of control to an external focus of control is paramount. While using an internal focus of control is required early in rehabilitation to enable the athlete to understand the specific tasks required in a composite movement, a gradual transition to an external focus of control is necessary as the athlete begins to perform tasks that are more soccer-specific. This autonomous stage of motor learning is crucial because it transfers the burden of motor planning from higher to lower levels of the CNS and frees up the pre-SMA and primary motor cortex to handle more complex patterns.58,86-88
Continue to: ANTERIOR CRUCIATE LIGAMENT RISK POTENTIAL IN SOCCER PLAYERS...
ANTERIOR CRUCIATE LIGAMENT RISK POTENTIAL IN SOCCER PLAYERS
If a comprehensive neuromotor rehabilitation program is to be used effectively with soccer athletes, then the first priority is to define how the players should move, so that they can demonstrate protective kinematics with all soccer-specific tasks and minimize stress to the ACL. As the ideal movement pattern becomes autonomous, then it should be trained within the context of a dynamic environment; remembering that environmental changes have a large impact on the final movement pattern selected by the individual. Brophy et al89 evaluated videos of non-contact ACL injuries in male and female soccer players and determined that 45% occurred while cutting, 25% while landing, and 16% during deceleration. These 3 patterns represent 86% of the ACL injuries observed and offer an opportunity for evaluation and treatment with specific central neuromotor rehabilitation techniques.
The foundational movement patterns for the soccer player should focus on producing leverage that minimizes stress to the ACL during the 3 primary tasks outlined above. To achieve this, it is necessary to reduce posterior ground reaction forces at the hip and knee joint during these movements. There is a high correlation between the magnitude of the posterior ground reaction force, and anterior tibial shear, and subsequent displacement.90,91 This stress can be reduced by increasing the hip and knee flexion angles during soccer-specific movements that involve pivoting, decelerating, and landing from a jump in a unipedal stance.92
This phenomenon can be explained by observing changes in the ACL elevation angle, hamstring insertion angle, and patella tendon-tibial tuberosity insertion angle. As the knee moves into flexion, the ACL takes on a more parallel orientation to the tibia, and its fibers are better able to resist elastic deformation accompanied by a posterior ground reaction force.93,94 The quadriceps will produce less anterior translation on the tibia because the patella tendon insertion angle is reduced relative to the longitudinal axis of the tibia, and the mechanical advantage of the quadriceps is decreased.95 Lastly, the hamstrings will be able to provide better leverage posteriorly because the resultant force trends toward a more parallel orientation to the tibial plateau, which enables the player to counter, more effectively, the posterior ground reaction force and the anterior pull directed by the quadriceps.95
This theory is supported by the work of Li and colleagues,96 who showed that there is an inverse relationship between knee flexion angle and ACL loading. In their study, they applied a constant quadriceps force of 200 N at 15°, 30°, and 60° angles. The anterior shear force was obviously the highest at 15° and reduced by 20% at 30° and 60% at 60°. When hamstrings co-contraction was added, there was an additional 30% reduction in anterior shear at 15° and 50% at 30° and 60°. From a more flexed position, the hamstrings can increase joint compression and reduce the anterior translation by allowing the concave medial tibial plateau to limit the anterior drawer effect and absorb the forces that occur with excessive anterior shear, internal rotation, and valgus loads.97 As the knee flexion angle approximates 60°, the hamstring leverage is increased, and the quadriceps leverage is diminished to the point where its ability to produce anterior tibial translation is neutralized.98 Daniel and colleagues98 referred to this as the quadriceps neutral angle.
For soccer-specific movements that are potentially injurious to the ACL, it may then be beneficial to create a default movement pattern at the knee that approximates this value. In keeping with the information presented in this paper, it will be important to have the player reproduce this angle consistently during activities that involve pivoting, decelerating, and landing from a jump within the context of match play. This will certainly require that segments located both proximal and distal to the knee are able to function within specific parameters so that a cohesive protective synergy is produced throughout the lower quarter which minimizes posterior ground reaction forces and is protective of the ACL. This is where structured neuromotor training that is able to modulate networks within the CNS may be beneficial.
Continue to: CENTRAL NERVOUS SYSTEM TREATMENT TECHNIQUES FOR THE SOCCER PLAYER...
CENTRAL NERVOUS SYSTEM TREATMENT TECHNIQUES FOR THE SOCCER PLAYER
The ultimate goal is to create a foundational movement pattern that optimizes leverage and is protective of the ACL during decelerating, pivoting, and landing in a unipedal stance from a jump. The composite segments that are necessary to achieve this include local core stability to create lumbopelvic stiffness, and global core activation to enhance posterior chain stability. This should enable the player to feel more balanced when placing the pelvis in a more posterior and inferior position while still maintaining the trunk in a position that is parallel with the tibia, as the knee is flexed to an approximate 60° angle (Figure 1). From a frontal plane perspective, the acetabulum should bisect the malleoli of the stance leg, with a neutral tibiofemoral joint alignment (Figure 1).
The neuromotor training for the composite segments of this movement can begin in the “acute postoperative phase” (Table 1). Because the surgical repair will limit the player’s capabilities in this stage, this is a good time to break down the foundational movement pattern into its component parts and ensure that the CNS receives a high number of quality repetitions of parts. In this phase, the player may begin an isolated training for the transversus abdominis, multifidus, and pubococcygeus. This can start in a supine position using biofeedback with an isometric contraction, progress to a standing position, and incorporate deep core activation with stance-phase gait training, mini squats, and lunge variations. This phase will require an abundance of visual and verbal feedback with an internal focus of control as the player gets used to activating the deep core and quad/hip synergy during functional lower extremity movements. Even in this early phase, the player should look to minimize anterior and/or posterior pelvic tilting and maintain a stiff thoracolumbar segment that remains parallel with the tibia during all functional movements.
Table 1. Adjunct CNS Treatment Principles for ACL Reconstruction in Soccer Athletes
Phase | Goal | CNS Rehabilitation Techniques |
Acute postoperative | Local core activation with weightbearing exercise. Produce trunk stiffness with lower extremity movements. | High repetitions. Verbal or tactile cues. Internal focus of control. Partial practice. |
Subacute postoperative | Lumbopelvic, foot or ankle, and posterior chain segments learn to participate in movement effectively. | Requires higher levels of cortical planning. Internal focus of control. CIMT. |
Static stability | Able to adopt foundational movement pattern consistently with vision eliminated. | Somatosensory vs visual processing. Partial-to-whole practice. Use internal and/or external focus of control. |
Dynamic stability | Able to perform plyometrics in a single-leg position using foundational movement pattern with subcortical processing. | Increase velocity with movement challenges. Occlude or eliminate vision. Heavy reliance on an external focus of control. Prioritize movement quality. |
Dynamic agility | Pivoting, decelerating, and landing are performed with hip flexion/knee flexion synergy, trunk stiffness, and posterior chain activation. | Unanticipated movement challenges. Whole practice. Ball reaction drills with vision obstructed or occluded. Contact drills with vision obstructed or occluded. Use external focus of control to include soccer-specific tasks. |
Abbreviations: ACL, anterior cruciate ligament; CNS, central nervous system; CIMT, constraint-induced movement therapy.
As the player moves into the “subacute postoperative phase” (Table 1). He or she will continue to use an internal focus of control to activate the local/global core synergy with functional movements progressing from double-leg, to single-leg positions. Partial practice, instead of whole practice, is still the predominant theme of the neural training process. In this phase, the knee and hip flexion angles can increase, and the player’s trunk and pelvic position should be critiqued in a single-leg position so that the trunk remains parallel with the tibia in the sagittal plane with a slight forward hip hinge. The pelvis should remain level throughout single-leg stance to ensure adequate activation of the lateral hip stabilizers. This is the stage where the player can learn to isolate closed kinetic chain hip rotation for pivoting, and so, single-leg hip internal and external rotation drills are useful, both with and without resistance. Skill acquisition is crucial in this phase because the patterns that the CNS adopts will form the foundation for more dynamic patterns that will occur in the later soccer-specific stages. Higher levels of cortical planning are still needed in this phase. For this reason, it is important that poor quality repetitions are recognized by the player and clinician so that he or she can learn to perform them correctly, albeit still with an internal focus of control. This is also a good time to begin to employ constraint-induced movement therapy as the player is able to replicate the desired pattern with more precision. For example, by eliminating the use of the upper extremities as a source of balance, the CNS is forced to program alternate synergies such as the lumbopelvic, and foot and ankle segments to maintain the desired alignment.
The “lower quarter static stability phase” (Table 1) marks a point where it may be useful to use direct strategies that have the capability to change CNS efferent owutput from a primary reliance on the visual processing areas in the posterior-inferior temporal gyrus back to the somatosensory area. It is critical that the player is able to make this transition in cortical reorganization and control, because ACL-injured subjects have been shown to have balance scores similar to healthy controls when they are able to use their vision, but this is reduced when vision is taken away.99-105 Their balance will diminish even further if vision is modulated during more complex landing and pivoting maneuvers.99-105 This may certainly explain why defending is a riskier task for ACL-injured players89 as their visual system is focused more on tracking a player than attending to precision with movement planning.
To enhance this cortical reorganization within the context of soccer-specific movements, it is useful to start from a foundational single-leg position, with the knee approximating 60° of flexion and the trunk parallel to the tibia. In the frontal plane, the pelvis should be level, the trunk vertical, and the acetabulum bisecting the malleoli of the stance leg (Figure 1). The player may initially work on getting into this foundational position with vision either partially obstructed using stroboscopic eyewear or completely obstructed if this equipment is unavailable.106-108 The pattern can be progressed by constraining the upper extremities to force reliance on the lumbopelvic and foot/ankle strategies for balance. Head and/or trunk turns can be added to simulate the external focus of control that is required with movement in soccer. These should progress from slow to fast and anticipated to unanticipated as the player demonstrates competence in maintaining stability at each segment within the foundational stance position. Once this is in play, a ball should be introduced into the drills. As the player maintains the foundational position with vision diminished and upper extremities constrained, they should attempt to reach for or trap a ball from this position. If vision is completely obstructed, then the player can be instructed to open his/her eyes just as the ball arrives to induce a reactive response. Again, quality repetitions are essential for learning to occur, and subsequent skill acquisition to take place in the CNS; thus, close scrutiny should be paid to the qualitative essence of the movement patterns to ensure pristine biomechanics during this phase.
The “lower quarter dynamic stability phase” (Table 1) should continue with the same neuromotor training principles employed in the previous phase, except that the drills will now involve plyometrics. The player should ultimately progress from double-leg, to single-leg jumps and then linear to diagonal. Vision should still be obstructed and upper extremities constrained to channel the lumbopelvic region for force production and balance. Movement quality in the foundational position remains paramount with these drills to ensure that skill acquisition is occurring and injury risk is being mitigated. An external focus of control can be introduced by applying an unanticipated perturbation during a jump. Additional learning opportunities should include unanticipated head and trunk turns while landing in a unipedal stance from a jump. The task can be made more specific by having the player trap a pass while doing linear or diagonal single-leg hop progressions. In this manner, the player’s CNS can become reorganized to program the requisite synergies to maintain a protective foundational position on the stance leg, as the contralateral limb is required to perform work that is far outside the player’s base of support.
Continue to: The final segment of the CNS neuromotor rehabilitation program...
The final segment of the CNS neuromotor rehabilitation program is the “lower quarter dynamic agility phase” (Table 1), when the player will learn to perform an unanticipated directional change in a foundational position for the pivot leg. The player can begin this phase by initiating sprint-deceleration-pivot efforts, progressing at 45°, 90°, 120°, and 180° turns. This should be trained in both a forward and backpedal position. Close attention should be paid to the deceleration phase of the sprint-pivot effort, as this will set the player up to demonstrate protective kinematics during the pivot phase of the task. In this phase, the center of mass should become lower and move posteriorly, so that a deeper knee and hip flexion angle, supported by posterior chain synergies, can occur at the pivot point. This is an important skill for the player to acquire, as Cortes and colleagues92 have reported that female collegiate soccer players tend to perform a pivoting task with a more erect trunk position. In the same cohort, they also measured the mean knee flexion angle at initial contact during pivoting to be 24°.92 Movement patterns that reflect an elevated center of mass, with arms abducted away from the trunk, should be discouraged here. The drills can be progressed to have the player react to a command and perform unanticipated pivots within a 5 × 5-meter box to simulate defending. This should be progressed from eyes open and arms unconstrained, to vision disrupted and arms constrained. From here, an external focus of control can be added by playing a ball to the athlete. Vision should be withheld until the instant that the ball arrives at the player, when he/she is required to play the ball to an unanticipated spot. As is the case in all other phases of the neuromotor training, the quality of movement is the most important parameter to critique with each drill. From a qualitative standpoint, the player should demonstrate stiffness throughout the thoracolumbar region and power and control through the pelvis with each directional change. In addition, he or she should maintain a low and posteriorly oriented center of mass to optimize leverage in the hamstrings/gluteals compared with the quadriceps and reduce posterior ground reaction forces.
PSYCHOLOGICAL READINESS FOR RETURN TO PLAY
After an injury is sustained, an athlete is often subjected to a range of psychological responses in addition to the functional impairment, including stress, hesitancy, alterations in self-esteem, depression, fear of re-injury, and anxiety.43,109-111 The aforementioned responses are often at their height in the time immediately following an injury and generally subside over time during the rehabilitation process.110 The rates at which athletes experience psychological distress following an injury range between 5% and 19%; the levels are comparable with patients receiving treatment for mental health illness.43 However, these elements may persist, or even increase, in the later stages of the rehabilitation process as the topic of return to play is deliberated.112,113 If these fears are left unresolved, then a significant delay can be incurred during the rehabilitation process, which might ultimately jeopardize the successful return to play.114,115
When athletes have been cleared to return to sport, fear tends to be the most common reason for their decision to not return to play.21,116 The persistence of fear has clinical implications and warrants close monitoring to ensure that the athlete feels adequately supported in the decision to return to sport.117,118 Building the athlete’s confidence by addressing hesitation, lack of confidence, heightened awareness of risk or re-injury, and safe reintegration into athletic participation are important themes identified to encourage a safe return to play.43 A variety of validated tools can be integrated into an existing return-to-play decision-making algorithm (Table 2).118-120
Abbreviations: ACL, anterior cruciate ligament; CNS, central nervous system; CIMT, constraint-induced movement therapy.
Table 2. Self-Report Measurement Tools to Integrate into Return-to-Play Decision-Making Algorithm
Self-report Measurement Tools |
13-item Tampa Scale for Kinesiophobia |
Anterior Cruciate Ligament Return to Sport after Injury Scale (ACL-RSI) |
Global Rating Scale (GRS) |
International Knee Documentation Committee (IKDC) Subjective Knee Evaluation Form |
Knee Injury and Osteoarthritis Outcome Score (KOOS) |
Lysholm Knee Scoring Scale |
Short Form-36 Health Survey (SF-36) |
Subjective Patient Outcome for Return to Sport (SPORTS) |
Patient Health Questionnaire-9 |
By integrating the necessary screening of patients for kinesiophobia and assessing patient expectations after enduring an ACL injury, clinicians may be able to identify patients who are at risk for poorer functional outcomes. A consideration of psychosocial elements such as activity avoidance, fear of movement and re-injury, loss of confidence and expectations/assumptions during the continuum of the rehabilitation process, and the decision to return to play may favorably impact the individual’s ability to safely return to sport. It is critical to address both the physical and psychosocial factors during the rehabilitation process to more optimally transition individuals back to their prior level of athleticism.
CONCLUSION
Psychosocial factors may play a role in determining a player’s readiness to return to sport, as well as a potential for re-injury. A number of tests are available for use with this patient population to identify mental deficits that may impact player performance upon return. Additionally, the CNS should be considered as a source of impairment in players with ACL injuries. Current protocols may not fully appreciate the CNS’ impact on the player’s functional outcome. Therefore, an approach that includes CNS neuromotor training with traditional musculoskeletal rehabilitation, which also incorporates cognitive and psychosocial factors, may define an improved paradigm for treating soccer athletes following an ACL injury and assessing return-to-play capability.
- Shumway-Cook A, Woollacott MH Motor control: translating research into clinical practice. 4th ed: Lippincott, Williams and Wilkins, 2012.
- Ardern CL, Webster KE, Taylor NF, Feller JA. Return to the preinjury level of competitive sport after anterior cruciate ligament reconstruction surgery: two-thirds of patients have not returned by 12 months after surgery. Am J Sports Med. 2011;39(3):538-543. doi:10.1177/0363546510384798.
- Bauer M, Feeley BT, Wawrzyniak JR, Pinkowsky G, Gallo RA. Factors affecting return to play after anterior cruciate ligament reconstruction: a review of the current literature. Phys Sportsmed. 2014;42(4):71-79. doi:10.3810/psm.2014.11.2093.
- Eisenstein ED, Rawicki NL, Rensing NJ, Kusnezov NA, Lanzi JT. Variables afftecting return to play after anterior cruciate ligament injury in the national football league. Orthop J Sports Med. 2016;4(10):2325967116670117.
- Ellman MB, Sherman SL, Forsythe B, LaPrade RF, Cole BJ, Bach BR. Return to play following anterior cruciate ligament reconstruction. J Am Acad Orthop Surg. 2015;23(5):283-296. doi:10.5435/JAAOS-D-13-00183.
- Fabricant PD, Chin CS, Conte S, Conte S, Coleman SH, Pearle AD, Dines JS. Return to play after anterior cruciate ligament reconstruction in major league baseball athletes. Arthroscopy. 2015;31(5):896-900. doi:10.1016/j.arthro.2014.12.008.
- Morris RC, Hulstyn MJ, Fleming BC, Owens BD, Fadale PD. Return to play following anterior cruciate ligament reconstruction. Clin Sports Med. 2016;35:(4):655-668. doi:10.1016/j.csm.2016.05.009.
- Paterno MV, Rauh MJ, Schmitt LC, Ford KR, Hewett TE. Incidence of second ACL injuries 2 years after primary ACL reconstruction and return to sport. Am J Sports Med. 2014;42(7):1567-1573. doi:10.1177/0363546514530088.
- Sclafani MP, Davis CC. Return to play progression for rugby following injury to the lower extremity. A clinical commentary and review of the literature. Int J Sports Phys Ther. 2016;11(2):302-320.
- Walden M, Hagglund M, Magnusson H, Ekstrand J. ACL injuries in men’s professional football: a 15-year prospective study on time trends and return-to-play rates reveals only 65% of players still play at the top level 3 years after ACL rupture. Br J Sports Med. 2016;50(12):744-750. doi:10.1136/bjsports-2015-095952.
- Wiggins AJ, Grandhi RK, Schneider DK, Stanfield D, Webster KE, Myer GD. Risk of secondary injury in younger athletes after anterior cruciate ligament reconstruction: a systematic review and meta-analysis. Am J Sports Med. 2016;44(7):1861-1876. doi:10.1177/0363546515621554.
- Arundale AJ, Cummer K, Capin JJ, Zarzycki R, Snyder-Mackler L. Report of the clinical and functional primary outcomes in men of the ACL-SPORTS trial: similar outcomes in men receiving secondary prevention with and without perturbation training 1 and 2 years after ACL reconstruction. Clin Orthop Relat Res. 2017;475(10):2523-2534. doi:10.1007/s11999-017-5280-2.
- Brumitt J, HB, Manske RC, Niemuth PE, Rauh MJ. Lower extremity functional tests and risk of injury in division III collegiate athletes. Int J Sports Phys Ther. 2013;8:216-227.
- Cacolice PA, Carcia CR, Scibek JS, Phelps AL. The use of functional tests to predict sagittal plane knee kinematics in ncaa-d1 female athlets. Int J Sports Phys Ther. 2015;10(4):493-504.
- Goodstadt NM, Hunter-Giordano A, Axe MJ, Snyder-Mackler L. Functonal testing to determine readiness to discontinue brace use one year after acl reconstruction. Int J Sports Phys Ther. 2013;8(2):91-96.
- Herbst E, Hoser C, Hildebrandt C, Raschner C, Hepperger C, Pointner H, Fink C. Functional assessments for decision-making regarding return to sports following ACL reconstruction. Part ll: clinical application of a new test battery. Knee Surg Sports Traumatol Arthrosc. 2015;23(5):1283-1291. doi:10.1007/s00167-015-3546-3.
- Hoog P, Warren M, Smith CA, Chimera NJ. Functional hop tests and tuck jump assessment scores between female division l collegiate athletes participating in high versus low acl injury prone sports: a cross sectional analysis. Int J Sports Phys Ther. 2016;11(6):945-953.
- Mohammadi F, Salavati M, Akhbari B, Mazaheri M, Mohsen Mir S, Etemadi Y. Comparison of functional outcome measures after ACL reconstruction in competitive soccer players: a randomized trial. J Bone Joint Surg, (Am.). 2013;95(14):1271-1277. doi:10.2106/JBJS.L.00724.
- Rambaud A, Samozino P, Edouard P. Functional tests can they help in the decision to return to sports after anterior cruciate ligament? Example with hop tests. Ann Phys Rehabil Med. 2016;59s:e19-ee20. doi:10.1016/j.rehab.2016.07.047.
- Xergia SA, Pappas E, Zampeli F, Georgiou S, Georgoulis AD. Asymmetries in functional hop tests, lower extremity kinematics, and isokinetic strength persist 6 to 9 months following anterior cruciate ligament reconstruction. J Orthop Sports Phys Ther. 2013;43(3):154-162. doi:10.2519/jospt.2013.3967.
- Ardern CL, Taylor NF, Feller JA, Webster KE. A systematic review of the psychological factors associated with returning to sport following injury. Br J Sports Med. 2013;47(17):1120-1126. doi:10.1136/bjsports-2012-091203.
- Ardern CL, Taylor NF, Feller JA, Whitehead TS, Webster KE. Psychological responses matter in returning to preinjury level of sport after anterior cruciate ligament reconstruction surgery. Am J Sports Med. 2013;41(7):1549-1558. doi:10.1177/0363546513489284.
- Christino MA, Fantry AJ, Vopat BG. Psychological aspects of recovery following anterior cruciate ligament reconstruction. J Am Acad Orthop Surg. 2015;23(8):501-509. doi:10.5435/JAAOS-D-14-00173.
- Naghdi S, Nakhostin Ansari N, Farhadi Y, Ebadi S, Entezary E, Glazer D. Cross-cultural adaptation and validation of the Injury-Psychological Readiness to Return to Sport scale to Persian language. Physiother Theory Pract. 2016;32(7):528-535. doi:10.1080/09593985.2016.1221486.
- Hui C, Salmon LJ, Kok A, Maeno S, Linklater J, Pinczewski LA. Fifteen-year outcome of endoscopic anterior cruciate ligament reconstruction with patellar tendon autograft for “isolated” anterior cruciate ligament tear. Am J Sports Med. 2011;39:89-98. doi:10.1177/0363546510379975.
- Paterno MV, Rauh MJ, Schmitt LC, Ford KR, Hewett TE. Incidence of contralateral and ipsilateral anterior cruciate ligament (ACL) injury after primary ACL reconstruction and return to sport. Clin J Sport Med. 2012;22:116-121. doi:10.1097/JSM.0b013e318246ef9e.
- Paterno MV, Schmitt LC, Ford KR, Rauh MJ, Myer GD, Huang B, Hewett TE. Biomechanical measures during landing and postural stability predict second anterior cruciate ligament injury after anterior cruciate ligament reconstruction and return to sport. Am J Sports Med. 2010;38:1968-1978. doi:10.1177/0363546510376053.
- Wright RW, Dunn WR, Amendola A, Andrish JT, Bergfeld J, Kaeding CC, Marx RG, McCarty EC, Parker RD, Wolcott M, Wolf BR, Spindler KP. Risk of tearing the intact anterior cruciate ligament in the contralateral knee and rupturing the anterior cruciate ligament graft during the first 2 years after anterior cruciate ligament reconstruction: a prospective MOON cohort study. Am J Sports Med. 2007;35:1131-1134. doi:10.1177/0363546507301318.
- Bystrom MG, Rasmussen-Barr E, Grooten WJ. Motor control exercises reduces pain and disability in chronic and recurrent low back pain: a meta-analysis. Spine. 2013;38(6):E350-E358. doi:10.1097/BRS.0b013e31828435fb.
- Macedo LG, Maher CG, Latimer J, McAuley JH. Motor control exercise for persistent nonspecific low back pain: a systematic review. Phys Ther. 2009;89(1):9-25. doi:10.2522/ptj.20080103.
- Grindstaff TL, Hammill RR, Tuzson AE, Hertel J. Neuromuscular control training programs and noncontact anterior cruciate ligament injur rates in female athletes: a numbers-needed-to-treat analysis. J Athl Train. 2006;41:450-456.
- Myer GD, Ford KR, Brent JL, Hewett TE. An integrated approach to change the outcome part II: Targeted neuromuscular training techniques to reduce identified ACL injury risk factors. J Strength Cond Res. 2012;26:2272-2292. doi:10.1519/JSC.0b013e31825c2c7d.
- Myer GD, Paterno MV, Ford KR, Hewett TE. Neuromuscular training techniques to target deficits before return to sport after anterior cruciate ligament reconstruction. J Strength Cond Res. 2008;22:987-1014. doi:10.1519/JSC.0b013e31816a86cd.
- Yoo JH, Lim BO, Ha M, Lee SW, Oh SJ, Lee YS, Kim JG. A meta-analysis of the effect of neuromuscular training on the prevention of the anterior cruciate ligament injury in female athletes. Knee Surg Sports Traumatol Arthrosc. 2010;18:824-830. doi:10.1007/s00167-009-0901-2.
- Moseley GL, Flor H. Targeting cortical representations in the treatment of chronic pain; a review. Neurorehabil Neural Repair. 2012;26(6):646-652. doi:10.1177/1545968311433209.
- Cramer SC. Brain repair after stroke. New Engl J Med 2010;362(19):1827-1829. doi:10.1056/NEJMe1003399.
- Baumeister J, Reinecke K, Weiss M. Changed cortical activity after anterior cruciate ligament reconstruction in a joint position paradigm: an EEG study. Scand J Med Sci Sports. 2008;18:473-484. doi:10.1111/j.1600-0838.2007.00702.x.
- Baumeister J, Reinecke K, Schubert M, Weiss M. Altered electrocortical brain activity after ACL reconstruction during force control. J Orthop Res. 2011;29:1383-1389. doi:10.1002/jor.21380.
- Courtney C, Rine RM. Central somatosensory changes associated with improved dynamic balance in subjects with anterior cruciate ligament deficiency. Gait Posture. 2006;24:190-195. doi:10.1016/j.gaitpost.2005.08.006.
- Courtney C, Rine RM, Kroll P. Central somatosensory changes and altered muscle synergies in subjects with anterior cruciate ligament deficiency. Gait Posture. 2005;22:69-74. doi:10.1016/j.gaitpost.2004.07.002.
- Kapreli E, Athanasopoulos S, Gliatis J, Papathanasiou M, Peeters R, Strimpakos N, Van Hecke P, Gouliamos A, Sunaert S. Anterior cruciate ligament deficiency causes brain plasticity: a functional MRI study. Am J Sports Med. 2009;37:2419-2426. doi:10.1177/0363546509343201.
- Valeriani M, Restuccia D, Di Lazaro V, Franceschi F, Fabbriciani C, Tonali P. Clinical and neurophysiological abnormalities before and after reconstruction of the anterior cruciate ligament of the knee. Acta Neurol Scand. 1999;99:303-307. doi:10.1111/j.1600-0404.1999.tb00680.x.
- Burland JP, Toonstra J, Werner JL, Mattacola CG, Howell DM, Howard JS. Decision to return to sport after anterior cruciate ligament reconstruction, Part 1: A qualitative investigation of psychosocial factors. J Athl Train. 2018;53(5):452-463. doi:10.4085/1062-6050-313-16
- Johansson H, Sjolander P, Sojka P. A sensory role for the cruciate ligaments. Clin Orthop Relat Res. 1991;268:161-178.
- Kaprelli E, Athanasopoulos S. The anterior cruciate ligament deficiency as a model of brain plasticity. Med Hypo. 2006;67:645-650. doi:10.1016/j.mehy.2006.01.063.
- Valeriani M, Restuccia D, Di Lazzaro V, Franceschi F, Fabbriciani C, Tonali P. Central nervous system modifications in patients with lesion of the anterior cruciate ligament of the knee. Brain. 1996;119(Pt 5):1751-1762. doi:10.1093/brain/119.5.1751.
- Nyland J, Fisher B, Brad E, Krupp R, Caborn DN. Osseous deficits after anterior cruciate ligament injury and reconstruction: a systematic review with suggestions to improve osseous homeostasis. Arthroscopy. 2010;26:1248-1257. doi:10.1016/j.arthro.2010.03.017.
- Nyland J, Klein S, Caborn DN. Lower extremity compensatory neuromuscular and biomechanical adaptations 2 to 11 years after anterior cruciate ligament reconstruction. Arthroscopy. 2010;26:1212-1225. doi:10.1016/j.arthro.2010.01.003.
- Paterno MV, Ford KR, Myer GD, Heyl R, Hewett TE. Limb asymmetries in landing and jumping 2 years following anterior cruciate ligament reconstruction. Clin. J Sports Med. 2007;17:258-262. doi:10.1097/JSM.0b013e31804c77ea.
- Wojtys EM, Huston LJ. Longitudinal effects of anterior cruciate ligament injury and patellar tendon autograft reconstruction on neuromuscular performance. Am J Sports Med. 2000;28:336-344. doi:10.1177/03635465000280030901.
- Levine JD, Dardick SJ, Basbaum AI, Scipio E. Reflex neurogenic inflammation. I. Contribution of the peripheral nervous system to spatially remote inflammatory responses that follow injury. J Neurosci. 1985;5:1380-1386. doi:10.1523/JNEUROSCI.05-05-01380.1985.
- McNair PJ, Marshall RN, Maguire K, Brown C. Knee joint effusion and proprioception. Arch Phys Med Rehabil. 1995;76:566-568. doi:10.1016/S0003-9993(95)80512-5.
- Jerosch J, Prymka M. Knee joint proprioception in normal volunteers and patients with anterior cruciate ligament tears, taking special account of the effect of a knee bandage. Arch Orthop Trauma Surg. 1996;115:162-166. doi:10.1007/BF00434546.
- Lattanzio PJ, Petrella RJ. Knee proprioception: a review of mechanisms, measurements, and implications of muscular fatigue. Orthopedics. 1998;21:463-470.
- Lephart SM, Pincivero DM, Giraido JL, Fu FH. The role of proprioception in the management and rehabilitation of athletic injuries. Am J Sports Med. 1997;25:130-137. doi:10.1177/036354659702500126.
- Lephart SM, Riemann BL, Fu FH. Introduction to the sensorimotor system. In: Lephart SM, Fu FH, editors. Proprioception and neuromuscular control in joint stability: Champaign (IL): Human Kinetics Publishers, 2000.
- Pelletier R, Higgins J, Bourbonnais D. Is neuroplasticity in the central nervous system the missing link to our understanding of chronic musculoskeletal disorders? BMC Musculoskelet Disord. 2015;16:25. doi:10.1186/s12891-015-0480-y.
- Magill R Motor learning and control: concepts and applications. Boston, MA: WCB/McGraw-hill. 8th ed, 2007.
- Winter DA Biomechanics and motor control of human movement. 4th ed: Hoboken, NJ:Wiley, 2009.
- Hoffman M, Koceja D. Hoffmann reflex profiles and strength rations in postoperative anterior cruciate ligament reconstruction patients. Int J Neurosci. 2000;104:17-27. doi:10.3109/00207450009035006.
- Duthon VB, Barea C, Abrassart S, Fasel JH, Fritschy D, Ménétrey J. Anatomy of the anterior cruciate ligament. Knee Surg Sports Traumatol Arthrosc. 2006;14:204-213. doi:10.1007/s00167-005-0679-9.
- Zimny ML. Mechanoreceptors in articular tissues. Am J Anat. 1988;182:16-32. doi:10.1002/aja.1001820103.
- Zimny ML, Schutte M, Dabezies E. Mechanoreceptors in the human anterior cruciate ligament. Anat Rec. 1986;214:204-209. doi:10.1002/ar.1092140216.
- Zimny ML, Wink CS. Neuroreceptors in the tissues of the knee joint. J Electromyogr Kinesiol. 1991;1:148-157. doi:10.1016/1050-6411(91)90031-Y.
- Ward S, Pearce AJ, Pietrosimone B, Bennell K, Clark R, Bryant AL. Neuromuscular deficits after peripheral joint injury: a neurophysiological hypothesis. Muscle Nerve. 2015;51(3):327-332. doi:10.1002/mus.24463.
- Ball T, Schreiber A, Feige B, Wagner M, Lücking CH, Kristeva-Feige R. The role of higher-order motor areas in voluntary movement as revealed by high resolution EEG and fMRI. Neuroimage. 1999;10:682-694. doi:10.1006/nimg.1999.0507.
- Nachev P, Wydell H, O’Neill K, Husain M, Kennard C. The role of the pre-supplementary motor area in the control of action. Neuroimage. 2007;36(suppl. 2):T155-TT163. doi:10.1016/j.neuroimage.2007.03.034.
- Binder JR, Desai RH. The neurobiology of semantic memory. Trends Cogn Sci. 2011;15:527-536. doi:10.1016/j.tics.2011.10.001.
- Bonner MF, Price AR. Where is the anterior temporal lobe and what does it do? J Neurosci. 2013;33:4213-4215. doi:10.1523/JNEUROSCI.0041-13.2013.
- Peuskens H, Vanrie J, Verfaillie K, Orban GA. Specificity of regions processing biological motion. Eur J Neurosci. 2005;21:2864-2875. doi:10.1111/j.1460-9568.2005.04106.x.
- Heroux ME, Tremblay F. Corticomotor excitability associated with unilateral knee dysfunction secondary to anterior cruciate ligament injury. Knee Surg Sports Traumatol Arthrosc. 2006;14:823-833. doi:10.1007/s00167-006-0063-4.
- Grooms D, Page S, Onate J. Brain activation for knee movement measured days before second anterior cruciate ligament injury: neuroimaging in musculoskeletal medicine. J Athl Train.2015;50(10):1005-1010. doi:10.4085/1062-6050-50.10.02.
- Stoodley CJ, Schmahmann JD. Functional topography in the human cerebellum: a meta-analysis of neuroimaging studies. Neuroimage. 2009;44(2):489-501. doi:10.1016/j.neuroimage.2008.08.039.
- Mansour A, Farmer M, Baliki M, Apkarian AV. Chronic pain: the role of learning and brain plasticity. Restor Neurol Neurosci. 2014;32:129-139. doi:10.3233/RNN-139003.
- Nyland J, Wera J, Klein S, Caborn DN. Lower extremity neuromuscular compensations during instrumented single leg hop testing 2-10 years following ACL reconstruction. Knee. 2014;21:1191-1197. doi:10.1016/j.knee.2014.07.017.
- Van Vliet PM, Heneghan N. Motor control and the management of musculoskeletal dysfunction. Man Ther. 2006;11(3):208-213. doi:10.1016/j.math.2006.03.009.
- Bayona NA, Bitensky J, Teasell R. Plasticity and reorganization of the uninjured brain. Top Stroke Rehabil. 2005;12:1-10. doi:10.1310/A422-G91U-Q4HB-86XC.
- Remple M, Bruneau R, VandenBerg P, Goertzen C, Kleim JA. Sensitivity of cortical movement representations to motor experience evidence that skilled learning but not strength training induces cortical reorganization. Behav Brain Res. 2001;123:133-141. doi:10.1016/S0166-4328(01)00199-1.
- Jull GA, Falla D, Vicenzino B, Hodges PW. The effect of therapeutic exercise on activation of the deep cervical flexor muscles in people with chronic neck pain. Man Ther. 2009;14:696-701. doi:10.1016/j.math.2009.05.004.
- Karni A, Meyer G, Jezzard P, Adams MM, Turner R, Ungerleider LG. Functional MRI evidence for adult motor cortex plasticity during motor skill learning. Nature. 1995;377:155-158. doi:10.1038/377155a0.
- Koeneke S, Lutz K, Herwig U, Ziemann U, Jäncke L. Extensive training of elementary finger tapping movements changes the pattern of motor cortex excitability. Exp Brain Res. 2006;174:199-209. doi:10.1007/s00221-006-0440-8.
- O’Leary S, Falla D, Elliott JM, Jull G. Muscle dysfunction in cervical spine pain: implications for assessment and management. J Orthop Sports Phys Ther. 2009;39:324-333. doi:10.2519/jospt.2009.2872.
- Pascual-Leone A, Nguyet D, Cohen LG, Brasil-Neto JP, Cammarota A, Hallett M. Modulation of muscle responses evoked by transcranial magnetic stimulation during the acquisition of new fine motor skills. J Neurophysiol. 1995;74:1037-1045. doi:10.1152/jn.1995.74.3.1037.
- Svensson P, Romaniello A, Wang K, Arendt-Nielsen L, Sessle BJ. One hour of tongue-task training is associated with plasticity in corticomotor control of the human tongue musculature. Exp Brain Res. 2006;173:165-173. doi:10.1007/s00221-006-0380-3.
- Tsao H, Druitt TR, Schollum TM, Hodges PW. Motor training of the lumbar paraspinal muscles induces immediate changes in motor coordination in patients with recurrent low back pain. J Pain. 2010;11:1120-1128. doi:10.1016/j.jpain.2010.02.004.
- Gokeler A, Banjaminse A, Hewett TE, Paterno MV, Ford KR, Otten E, Myer GD. Feedback techniques to target functional deficits following anterior cruciate ligament reconstruction: implications for motor control and reduction of second injury risk. Sports. Med. 2013;43:1065-1074. doi:10.1007/s40279-013-0095-0.
- Powers CM, Fisher B. Mechanisms underlying ACL injury-prevention training: the brain-behavior relationship. J Athl Train. 2010;45:513-515. doi:10.4085/1062-6050-45.5.513.
- Seidler RD, Noll DC. Neuroanatomical correlates of motor acquisition and motor transfer. J Neurophysiol. 2008;99:1836-1845. doi:10.1152/jn.01187.2007.
- Brophy RH, Stepan JG, Silvers HL, Mandelbaum BR. Defending puts the anterior cruciate ligament at risk during soccer: a gender-based analysis. Sports Health. 2015;7:244-249. doi:10.1177/1941738114535184.
- Sell TC, Ferris CM, Abt JP, Tsai YS, Myers JB, Fu FH, Lephart SM. Predictors of proximal tibia anterior shear force during a vertical stop-jump. J Orthop Sports Res. 2007;25:1589-1597. doi:10.1002/jor.20459.
- Yu B, Lin CF, Garrett WE. Lower extremity biomechanics during the landing of a stop-jump task. Clin Bio. 2006;21:297-305. doi:10.1016/j.clinbiomech.2005.11.003.
- Cortes N, Onate J, Van Lunen B. Pivot task increases knee frontal plane loading compared with sidestep and drop-jump. J Sports Sci. 2011;29:83-92. doi:10.1080/02640414.2010.523087.
- Blackburn JT, Padua DA. Influence of trunk flexion on hip and knee joint kinematics during a controlled drop landing. Clin Biomech. 2008;23:313-319. doi:10.1016/j.clinbiomech.2007.10.003.
- Li G, Papannagari R, DeFrate LE, Yoo JD, Park SE, Gill TJ. Comparison of the ACL and ACL graft forces before and after ACL reconstruction: an in-vitro robotic investigation. Acta Orthop. 2006;77:267-274. doi:10.1080/17453670610046019.
- Zheng N, Fleisig GS, Escamilla RF, Barrentine SW. An analytical model of the knee for estimation of internal forces during exercise. J Biomech. 1998;31:963-967. doi:10.1016/S0021-9290(98)00056-6.
- Li G, Rudy TW, Sakan M, Kanamori A, Ma CB, Woo SL. The importance of quadriceps and hamstring muscle loading on knee kinematics and in situ forces in the ACL. J Biomech. 1999;32:395-400. doi:10.1016/S0021-9290(98)00181-X.
- Hewett TE, Myer GD, Ford KR. Anterior cruciate ligament injuries in female athletes, Part l: Mechanisms and risk factors. Am J Sports Med.2006;34:299-311. doi:10.1177/0363546505284183.
- Daniel DM, Stone ML, Barnett P. Use of the quadriceps active test to diagnose to diagnose posterior cruciate ligament disruption and measure posterior laxity of the knee. J Bone Jt SurA, (Am.).1988;70:386-391.
- Hoffman M, Schrader J, Koceja D. An investigation of postural control in postoperative anterior cruciate ligament reconstruction patients. J Athl Train. 1999;34:130-136.
- Mattacola CG, Perrin DH, Gansneder BM, Gieck JH, Saliba EN, McCue FC. Strength, functional outcome, and postural stability after anterior cruciate ligament reconstruction. J Athl Train.2002;37:262-268.
- McLean SG, Lipfert SW, van den Boget AJ. Effect of gender and defensive opponent on the biomechanics of sidestep cutting. Med Sci Sports Exerc. 2004;36:1008-1016. doi:10.1249/01.MSS.0000128180.51443.83.
- McLean SG, Neal RJ, Myers PT, Walters MR. Knee joint kinematics during the sidestep cutting maneuver: potential for injury in women. Med Sci Sports Exerc. 1999;31:959-968. doi:10.1097/00005768-199907000-00007.
- O’Connell M, George K, Stock D. Postural sway and balane testing: a comparison of normal and anterior cruciate ligament deficient knees. Gait Posture. 1998;8:136-142. doi:10.1016/S0966-6362(98)00023-X.
- Okuda K, Abe N, Katayama Y, Senda M, Kuroda T, Inoue H. Effect of vision on postural sway in anterior cruciate ligament injured knees. J Orthop Sci. 2005;10:277-283. doi:10.1007/s00776-005-0893-9.
- Swanik CB, Lephart SM, Giraldo JL, Demont RG, Fu FH. Reactive muscle firing of anterior cruciate ligament-injured females during functional activities. J Athl Train. 1999;34:121-129.
- Bennett S, Ashford D, Rioja N, Elliott D. Intermittent vision and one-handed catching: the effect of general and specific task experience. J Mot Behav. 2004;36:442-449. doi:10.3200/JMBR.36.4.442-449.
- Bennett SJ, Elliott D, Weeks DJ, Keil D. The effects of intermittent vision on prehension under binocular and monocular viewing. Mot Contr. 2003;7:46-56. doi:10.1123/mcj.7.1.46.
- Grooms D, Appelbaum G, Onate J. Neuroplasticity following anterior cruciate ligament injury: a framework for visual-motor training approaches in rehabilitation. J Orthop Sports Phys Ther.2015;45(5):381-393. doi:10.2519/jospt.2015.5549.
- Ardern CL. Anterior cruciate ligament reconstruction-not exactly a one-way ticket back to the preinjury level: a review of contextual factors affecting return to sport after surgery. Sports Health.2015;7(3):224-230. doi:10.1177/1941738115578131.
- Hsu CJ, Meierbachtol A, George SZ, Chmielewski TL. Fear of reinjury in athletes. Sports Health.2017;9(2):162-167. doi:10.1177/1941738116666813.
- Medvecky MJ, Nelson S. Kinesiophobia and return to sports after anterior cruciate ligament reconstruction. Conn Med. 2015;79(3):155-157.
- Chmielewski TL, ZG, Lentz TA, Tillman SM, Moser MW, Indelicato PA, George SZ. Longitudinal changes in psychosocial factors and their association with knee pain and function after anterior cruciate ligament reconstruction. Phys Ther. 2011;91:1355-1366. doi:10.2522/ptj.20100277.
- Clement D, Arvinen-Barrow M, Fetty T. A-BM, Fetty T. Psychosocial responses during different phases of sport-injury rehabilitation: a qualitative study. J Athl Train. 2014;50:95-104. doi:10.4085/1062-6050-49.3.52.
- Te Wierike SC, van der Sluis A, van den Akker-Scheek I, Elferink-Gemser MT, Visscher C. Psychosocial factors influencing the recovery of athletes with anterior cruciate ligament injury: a systematic review. Scand J Med Sci Sports. 2013;23(5):527-540. doi:10.1111/sms.12010.
- Wiese-Bjornstal DM. Psychology and socioculture affect injury risk, response, and recovery in high-intensity athletes: a consensus statement. Scand J Med Sci Sports. 2010;20(Suppl. 2):103-111. doi:10.1111/j.1600-0838.2010.01195.x.
- Crossman J, GL, Jamieson J. The emotional responses of injured athletes. NZ J Sports Med. 1995;23:1-2.
- Hambly K. The use of the tegner activity scale for articular cartilage repair of the knee: a systematic review. Knee Surg Sports Traumatol Arthrosc. 2011;19(4):604-614. doi:10.1007/s00167-010-1301-3.
- Hambly K, Griva K. IKDC or KOOS: which one captures symptoms and disabilities most important to patients who have undergone initial anterior cruciate ligament reconstruction? Am J Sports Med. 2010;38(7):1395-1404. doi:10.1177/0363546509359678.
- Archer K, Reinke E, Huston LJ, Bird M, Scaramuzza E, Coronado R, Haug C, Vanston S, Spindler KP. Impact of preoperative expectations and fear of movement on return to sport and KOOS scores at 6 months following ACL reconstruction. Orthop J Sports Med. 2015;3(7 suppl.2):2325967115S2325900113. doi:10.1177/2325967115S00113.
- Zarzycki R, Failla M, Arundale AJH, Capin JJ, Snyder-Mackler L. Athletes with a positive psychological response to return to sport training have better outcomes one and two years after ACL reconstruction. Orthop J Sports Med. 2017;5(7 suppl.6):2325967117S2325900324. doi:10.1177/2325967117
ABSTRACT
Soccer players recovering from anterior cruciate ligament (ACL) injuries have better options for treatment today than they did 25 years ago. Surgical techniques have improved, and rehabilitation protocols have evolved considerably. Although the rehabilitation community is doing a better job of treating this patient population, the evidence does demonstrate that both re-injury and return- to-play (RTP) rates are still suboptimal. Most protocols focus on normalizing strength and range of motion (ROM) and achieving limb symmetry with soccer-specific movements. While these factors are certainly prerequisites for returning to the field, their inclusion does not provide a complete picture of the athlete’s presentation. An additional factor that should be prioritized with this patient population is the central nervous system (CNS). Advanced imaging has shown that peripheral deafferentation does occur with musculoskeletal injuries; this ultimately results in cortical reorganization, which makes movement planning more difficult for the player, since simpler tasks must now be processed at higher levels in the CNS. The evidence also shows that the CNS demonstrates plasticity in these cases, so that through focused neuromotor rehabilitation techniques, it is possible to bring movement planning back down to a sub-cortical level. Cognitive issues may also be a factor in preventing the player from returning. Fear of re-injury and diminished confidence can influence the way the player moves on the field, and diminish ability to demonstrate protective kinematics with all soccer-specific tasks. We believe that an approach incorporating traditional musculoskeletal rehabilitation, CNS neuro-motor training, and consideration for cognitive factors, may define an improved paradigm for treating the soccer player and assessing readiness for RTP following ACL injury.
Continue to: Although anterior cruciate ligament (ACL)...
Although anterior cruciate ligament (ACL) rehabilitation has evolved considerably over the past 2 decades, the basic paradigm has remained consistent: normalize strength and range of motion, reduce swelling and pain, achieve limb symmetry with functional tasks, and return to sport-specific activities gradually over a 6 to 12-month period. There have been some slight additions to this basic premise, such as evaluating knee and hip mechanics in the frontal plane, but the requirements here are vaguely defined and are typically only evaluated within the context of controlled clinical testing.
It is interesting to note that the typical ACL injury pattern occurs during a normal sport-specific movement, yet most rehabilitation protocols fail to recognize the potential causes of the aberrant movement pattern and how to best modify it so that the risk of repeated stress to the ACL can be minimized. It should be understood that movement occurs through the interaction of 3 discrete factors: the individual, the task being performed, and the environment in which it is performed.1 All of these factors will play a role in how the final movement pattern is produced. For example, a soccer player (individual) may backpedal and pivot to the left 60° and accelerate to sprint after a player moving towards the touchline (task) while receiving instructions from teammates and monitoring the movements of opposing players (environment). A small variance in any 1 of these factors could significantly impact the movement pattern as the player completes the task.
In most rehabilitation programs, each of these factors may be treated in a singular, non-specific manner, but if these factors are not coordinated effectively throughout the program to produce the desired sport-specific movement, a faulty pattern may persist, leaving the player at risk for injury. Current rehabilitation programs seem to have a strong focus on creating stability, mobility, and strength, but these are trained in silos, with an internal focus of control, which only solves the biomechanical equation. Often, it is difficult for the player to coordinate good biomechanics into an efficient, protective movement pattern that is specific to the tasks performed on the field during the normal course of play. The missing link here is the central nervous system (CNS).
Limitations to the current ACL protocols may be that they rely heavily on musculoskeletal rehabilitation and that they have limited emphasis on neurological rehabilitation. As will be discussed later, the CNS has a large impact on the final movement selected by the player. In fact, cognition, perception, and action are the three factors that comprise the individual’s part of the movement paradigm,1 yet rarely are these factors addressed in most ACL rehabilitation programs. These elements are a large part of the movement equation, so it is easy to understand how failing to address these features can lead to poor movement quality and subsequent ACL re-injury.
In addition to central neurological factors, cognitive issues may play a role in the player’s ability to return to sport. Determining optimal readiness for return to play is a difficult task for the medical community, with many variables to consider. Previous research studies have assessed the variability in return to play for various sports, including football, rugby, soccer, skiing, running, and tennis, with return-to-play rates ranging from 18% to 100%.2,3,4-10 The risk of secondary injury may cast doubt and fear on athletes as they contemplate their successful return to play.8,11 Although robust functional testing has become commonplace for determining athlete readiness after injury,12-20 the assessment of psychological readiness, persistent fear, and loss of confidence are often neglected and not as commonly integrated into the return-to-play algorithm.21-24 The purpose of this paper is to assess the various cognitive and central neural factors affecting a soccer player’s ability to recover from an ACL injury and offer suggestions for integrating treatments into the protocols to address these issues.
Continue to: CENTRAL NERVOUS SYSTEM NEUROPLASTICITY...
CENTRAL NERVOUS SYSTEM NEUROPLASTICITY
Despite the vast amount of attention and research focused on the ACL, the re-injury rate still remains quite high. It has been reported that rehabilitation programs that employ traditional neuromotor training produce a re-injury rate as high as 30% after the athlete returns to sport.25-28 The overall rate of sustaining a second ACL injury is 15%11 in all patient populations. For the general population <25 years of age, the re-injury rate is 21%, and for athletes <25 years of age, the re-injury rate rises to 23%.11 With re-injury rates at this level, it is certainly fair to consider and be critical of the current rehabilitation methods being used with this population. One opportunity for improvement lies in the general approach used to rehabilitate ACL-injured patients. Therapy for this injury is protocol-driven, and the fact remains that most protocols prioritize restoration of peripheral systems, with minimal thought given to the cortical control necessary to manage those systems.29,30 When neural factors are considered, it is usually within the context of increasing strength, balance, power, and biomechanical control,31-34 which are certainly important but peripheral factors nonetheless. The missing element in many ACL protocols may be how to best manage the central neural components and cognitive factors associated with this injury.
If the CNS were to receive more consideration in ACL protocols, the opportunity for improved outcomes could be substantial because the CNS has been proven to be a very malleable system, as long as it receives the correct input. The CNS demonstrates neuroplasticity,35 which means that it is capable of reorganization, based on the stimuli that it receives, whether internal or external.36
This is an important consideration in ACL rehabilitation because the ACL graft, while restoring the biomechanical properties to the knee, is not fully capable of producing the same neurosensory properties of the original ACL.37-42This is an important concept to understand because an ACL tear does indeed cause deafferentation in the ascending pathways to the brain.37-40,42-46 This can lead to CNS reorganization and subsequent alterations in efferent output to the periphery.37-40,42-46 Therefore, if a protocol with traditional musculoskeletal principles was used, then the mechanical function of the knee may certainly be remediated, but the neurosensory function will remain in a maladaptive state,47-50 potentially leading to aberrant, non-protective movement strategies and a higher risk of re-injury.
The process of CNS reorganization may begin with the initial ACL injury. A peripheral musculoskeletal injury creates an inflammatory response that results in the arrival of chemical mediators such as histamine, substance P, calcitonin, and calcitonin gene-related peptide at the site of injury.51 As edema accumulates in the joint, tension is applied to the capsule, which may adversely affect proprioception from the receptors located within.45 The interruption of consistent input from the peripheral mechanoreceptors may lead to long-term differentiation of the ascending pathways.52 This information is synthesized at 3 different levels of the CNS (spinal cord, brain stem, and motor cortex) to produce motor output.53-56 Differentiation in the ascending circuitry can cause inhibition of motor neurons at the spinal cord.45Animal research has shown that this differentiation can cause a breakdown in the cuneate nucleus of the brainstem,57which provides sensory information from the upper body, while the gracile nucleus does the same for the lower body. These structures transfer proprioceptive input to the ventral posterior lateral nucleus in the thalamus, where it is then sent to the primary somatosensory cortex.57 In general, the somatosensory, visual, and vestibular systems interpret afferent inputs to control movement, balance, and stability.58,59 In a sport like soccer, where the movement tasks are dynamic and unpredictable, it is easy to see why even a slight deficit in somatosensory processing could disrupt a movement. Valeriani and colleagues42,46 showed that somatosensory-evoked potentials were indeed altered in a cohort of ACL reconstruction (ACLR) subjects, indicating reorganization within the CNS. Additionally, the deafferentation could not be changed by other afferent input coming from the knee or by the new ACL graft placed in the knee.42,46The primary motor cortex has been found to have a substantial network of connectivity with the primary somatosensory cortex, which supports the theory that the motor cortex has a very strong linkage with the peripheral receptors in the joint.60 The ligaments in the joint contain Ruffini, Pacinian, and Golgi receptors, all of which react to changes in the collagen fibers and send information regarding tension, length, speed, acceleration, position, and movement back to the CNS.61-64 Unfortunately, the ascending pathway deafferentation can cause reorganization within the CNS, which makes the feedback provided from the periphery less effective in motor planning.
Ward and colleagues65 have reported that reorganization within the motor cortex is the primary cause of chronic neuromuscular movement deficits in peripheral joint injuries. Researchers have used functional magnetic resonance imaging, transcranial magnetic stimulation, and electroencephalography in ACL patients to demonstrate changes in cortical activity and subsequent CNS reorganization.65 Kapreli and colleagues41 reported that subjects with an ACL injury demonstrated higher cortical activation in the pre-supplementary motor area (pre-SMA). This is a region that is responsible for more complex motor planning.66,67 This area becomes active before the primary motor cortex and is responsible for preparing the final movement pattern that the motor cortex executes.41 As the task becomes more complex, activity in the pre-SMA will increase.41 Additionally, they found that the posterior secondary somatosensory area and posterior inferior temporal gyrus showed increased cortical activity compared with controls.41 Visual planning is processed in the posterior inferior temporal gyrus, and so, it appears that the difficulty in processing somatosensory information due to ascending pathway deafferentation places an increased reliance on the visual system for movement planning.68-70 This was observed while ACL-injured subjects performed a simple knee flexion-extension movement encompassing 40°, indicating the need to incorporate higher central levels of planning for a very simple movement pattern.41 Baumeister and colleagues37,38 also showed that subjects with ACLR had higher levels of cortical activation in the areas of the brain that require attention and that process sensory input. They theorized that this occurred because of reduced efficiency of neural processing at lower levels in the CNS. Despite the higher levels of cortical activity observed, they found that subjects with an ACLR demonstrated proprioceptive testing that was deficient compared with that of controls. Heroux and Tremblay71 also demonstrated that subjects with an ACLR had increased resting motor cortex activity. They believed that this occurred as the motor cortex attempted to maintain neuromotor output to the periphery in the face of diminished afferent input.
Continue to: The reorganization that results in movement planning...
The reorganization that results in movement planning, transitioning from subcortical levels to cortical levels, is a phenomenon that researchers believe can lead to deficiencies even as the athlete has returned to sport. Grooms et al72revealed in a case report that a subject with an ACLR showed higher levels of activity in the crus region of the cerebellum. This area contains corticobulbar and corticospinal tracts that transmit neural input to maintain balance and coordination.73 These changes in the cerebellum, combined with increased motor cortex activity, are thought to be indicative of a global neural strategy that uses higher levels of the CNS, as opposed to subcortical processing.72
The current research makes a clear and compelling argument for the importance of CNS reorganization after an ACL injury, placing increased reliance on higher cortical levels of control, as well as the visual system to coordinate balance and movement. It is thought that this reorganized method of neural transmission can then become imprinted within the CNS, if not corrected.35,74 If this is the case, then traditional strength programs may not be sufficient to restore these connections to their pre-injury level. If the CNS has the ability to reorganize based on the aberrant input that it receives from the periphery, then it also certainly has the potential to adapt to more specific structured input via the ascending afferent pathways.41,45 The rehabilitation program, however, needs to be structured specifically to target the reorganized regions of the brain. There needs to be an emphasis on rehabilitating not only the peripheral neuromotor structures but also the CNS.75
CENTRAL NERVOUS SYSTEM REHABILITATION PRINCIPLES
For a neurological rehabilitation to be successful, the interventions need to be repetitive and task-specific, involve learning, employ whole and part practice, and transition from using an internal to an external focus of control.76Movements that are repetitive, but which lack structured learning and skill, have been shown to have no effect on inducing neuroplastic changes in the primary motor cortex.77,78 However, using neurological rehabilitation techniques that facilitate the acquisition of new motor skills by the CNS have been shown to cause neuroplastic adaptation in the motor cortex.79-85 This occurs because neuroplasticity is determined by experience and practice.78 The CNS operates on cues received in the ascending tracts by mechanoreceptors in the joint. If a new movement pattern is being learned by the athlete, then this new afferent input received from the periphery will start to initiate reorganization in the higher learning centers. If this occurs with optimal repetition and precision, then a positive reorganization can take place within the CNS that results in a higher percentage of motor planning and control being filtered down to a subcortical level. Essentially, the movements become instinctive, which is crucial in athletics, where attention in higher cortical areas is frequently diverted to external aspects of the competition and not solely used to focus on movement.
This is why shifting neurological rehabilitation from an internal focus of control to an external focus of control is paramount. While using an internal focus of control is required early in rehabilitation to enable the athlete to understand the specific tasks required in a composite movement, a gradual transition to an external focus of control is necessary as the athlete begins to perform tasks that are more soccer-specific. This autonomous stage of motor learning is crucial because it transfers the burden of motor planning from higher to lower levels of the CNS and frees up the pre-SMA and primary motor cortex to handle more complex patterns.58,86-88
Continue to: ANTERIOR CRUCIATE LIGAMENT RISK POTENTIAL IN SOCCER PLAYERS...
ANTERIOR CRUCIATE LIGAMENT RISK POTENTIAL IN SOCCER PLAYERS
If a comprehensive neuromotor rehabilitation program is to be used effectively with soccer athletes, then the first priority is to define how the players should move, so that they can demonstrate protective kinematics with all soccer-specific tasks and minimize stress to the ACL. As the ideal movement pattern becomes autonomous, then it should be trained within the context of a dynamic environment; remembering that environmental changes have a large impact on the final movement pattern selected by the individual. Brophy et al89 evaluated videos of non-contact ACL injuries in male and female soccer players and determined that 45% occurred while cutting, 25% while landing, and 16% during deceleration. These 3 patterns represent 86% of the ACL injuries observed and offer an opportunity for evaluation and treatment with specific central neuromotor rehabilitation techniques.
The foundational movement patterns for the soccer player should focus on producing leverage that minimizes stress to the ACL during the 3 primary tasks outlined above. To achieve this, it is necessary to reduce posterior ground reaction forces at the hip and knee joint during these movements. There is a high correlation between the magnitude of the posterior ground reaction force, and anterior tibial shear, and subsequent displacement.90,91 This stress can be reduced by increasing the hip and knee flexion angles during soccer-specific movements that involve pivoting, decelerating, and landing from a jump in a unipedal stance.92
This phenomenon can be explained by observing changes in the ACL elevation angle, hamstring insertion angle, and patella tendon-tibial tuberosity insertion angle. As the knee moves into flexion, the ACL takes on a more parallel orientation to the tibia, and its fibers are better able to resist elastic deformation accompanied by a posterior ground reaction force.93,94 The quadriceps will produce less anterior translation on the tibia because the patella tendon insertion angle is reduced relative to the longitudinal axis of the tibia, and the mechanical advantage of the quadriceps is decreased.95 Lastly, the hamstrings will be able to provide better leverage posteriorly because the resultant force trends toward a more parallel orientation to the tibial plateau, which enables the player to counter, more effectively, the posterior ground reaction force and the anterior pull directed by the quadriceps.95
This theory is supported by the work of Li and colleagues,96 who showed that there is an inverse relationship between knee flexion angle and ACL loading. In their study, they applied a constant quadriceps force of 200 N at 15°, 30°, and 60° angles. The anterior shear force was obviously the highest at 15° and reduced by 20% at 30° and 60% at 60°. When hamstrings co-contraction was added, there was an additional 30% reduction in anterior shear at 15° and 50% at 30° and 60°. From a more flexed position, the hamstrings can increase joint compression and reduce the anterior translation by allowing the concave medial tibial plateau to limit the anterior drawer effect and absorb the forces that occur with excessive anterior shear, internal rotation, and valgus loads.97 As the knee flexion angle approximates 60°, the hamstring leverage is increased, and the quadriceps leverage is diminished to the point where its ability to produce anterior tibial translation is neutralized.98 Daniel and colleagues98 referred to this as the quadriceps neutral angle.
For soccer-specific movements that are potentially injurious to the ACL, it may then be beneficial to create a default movement pattern at the knee that approximates this value. In keeping with the information presented in this paper, it will be important to have the player reproduce this angle consistently during activities that involve pivoting, decelerating, and landing from a jump within the context of match play. This will certainly require that segments located both proximal and distal to the knee are able to function within specific parameters so that a cohesive protective synergy is produced throughout the lower quarter which minimizes posterior ground reaction forces and is protective of the ACL. This is where structured neuromotor training that is able to modulate networks within the CNS may be beneficial.
Continue to: CENTRAL NERVOUS SYSTEM TREATMENT TECHNIQUES FOR THE SOCCER PLAYER...
CENTRAL NERVOUS SYSTEM TREATMENT TECHNIQUES FOR THE SOCCER PLAYER
The ultimate goal is to create a foundational movement pattern that optimizes leverage and is protective of the ACL during decelerating, pivoting, and landing in a unipedal stance from a jump. The composite segments that are necessary to achieve this include local core stability to create lumbopelvic stiffness, and global core activation to enhance posterior chain stability. This should enable the player to feel more balanced when placing the pelvis in a more posterior and inferior position while still maintaining the trunk in a position that is parallel with the tibia, as the knee is flexed to an approximate 60° angle (Figure 1). From a frontal plane perspective, the acetabulum should bisect the malleoli of the stance leg, with a neutral tibiofemoral joint alignment (Figure 1).
The neuromotor training for the composite segments of this movement can begin in the “acute postoperative phase” (Table 1). Because the surgical repair will limit the player’s capabilities in this stage, this is a good time to break down the foundational movement pattern into its component parts and ensure that the CNS receives a high number of quality repetitions of parts. In this phase, the player may begin an isolated training for the transversus abdominis, multifidus, and pubococcygeus. This can start in a supine position using biofeedback with an isometric contraction, progress to a standing position, and incorporate deep core activation with stance-phase gait training, mini squats, and lunge variations. This phase will require an abundance of visual and verbal feedback with an internal focus of control as the player gets used to activating the deep core and quad/hip synergy during functional lower extremity movements. Even in this early phase, the player should look to minimize anterior and/or posterior pelvic tilting and maintain a stiff thoracolumbar segment that remains parallel with the tibia during all functional movements.
Table 1. Adjunct CNS Treatment Principles for ACL Reconstruction in Soccer Athletes
Phase | Goal | CNS Rehabilitation Techniques |
Acute postoperative | Local core activation with weightbearing exercise. Produce trunk stiffness with lower extremity movements. | High repetitions. Verbal or tactile cues. Internal focus of control. Partial practice. |
Subacute postoperative | Lumbopelvic, foot or ankle, and posterior chain segments learn to participate in movement effectively. | Requires higher levels of cortical planning. Internal focus of control. CIMT. |
Static stability | Able to adopt foundational movement pattern consistently with vision eliminated. | Somatosensory vs visual processing. Partial-to-whole practice. Use internal and/or external focus of control. |
Dynamic stability | Able to perform plyometrics in a single-leg position using foundational movement pattern with subcortical processing. | Increase velocity with movement challenges. Occlude or eliminate vision. Heavy reliance on an external focus of control. Prioritize movement quality. |
Dynamic agility | Pivoting, decelerating, and landing are performed with hip flexion/knee flexion synergy, trunk stiffness, and posterior chain activation. | Unanticipated movement challenges. Whole practice. Ball reaction drills with vision obstructed or occluded. Contact drills with vision obstructed or occluded. Use external focus of control to include soccer-specific tasks. |
Abbreviations: ACL, anterior cruciate ligament; CNS, central nervous system; CIMT, constraint-induced movement therapy.
As the player moves into the “subacute postoperative phase” (Table 1). He or she will continue to use an internal focus of control to activate the local/global core synergy with functional movements progressing from double-leg, to single-leg positions. Partial practice, instead of whole practice, is still the predominant theme of the neural training process. In this phase, the knee and hip flexion angles can increase, and the player’s trunk and pelvic position should be critiqued in a single-leg position so that the trunk remains parallel with the tibia in the sagittal plane with a slight forward hip hinge. The pelvis should remain level throughout single-leg stance to ensure adequate activation of the lateral hip stabilizers. This is the stage where the player can learn to isolate closed kinetic chain hip rotation for pivoting, and so, single-leg hip internal and external rotation drills are useful, both with and without resistance. Skill acquisition is crucial in this phase because the patterns that the CNS adopts will form the foundation for more dynamic patterns that will occur in the later soccer-specific stages. Higher levels of cortical planning are still needed in this phase. For this reason, it is important that poor quality repetitions are recognized by the player and clinician so that he or she can learn to perform them correctly, albeit still with an internal focus of control. This is also a good time to begin to employ constraint-induced movement therapy as the player is able to replicate the desired pattern with more precision. For example, by eliminating the use of the upper extremities as a source of balance, the CNS is forced to program alternate synergies such as the lumbopelvic, and foot and ankle segments to maintain the desired alignment.
The “lower quarter static stability phase” (Table 1) marks a point where it may be useful to use direct strategies that have the capability to change CNS efferent owutput from a primary reliance on the visual processing areas in the posterior-inferior temporal gyrus back to the somatosensory area. It is critical that the player is able to make this transition in cortical reorganization and control, because ACL-injured subjects have been shown to have balance scores similar to healthy controls when they are able to use their vision, but this is reduced when vision is taken away.99-105 Their balance will diminish even further if vision is modulated during more complex landing and pivoting maneuvers.99-105 This may certainly explain why defending is a riskier task for ACL-injured players89 as their visual system is focused more on tracking a player than attending to precision with movement planning.
To enhance this cortical reorganization within the context of soccer-specific movements, it is useful to start from a foundational single-leg position, with the knee approximating 60° of flexion and the trunk parallel to the tibia. In the frontal plane, the pelvis should be level, the trunk vertical, and the acetabulum bisecting the malleoli of the stance leg (Figure 1). The player may initially work on getting into this foundational position with vision either partially obstructed using stroboscopic eyewear or completely obstructed if this equipment is unavailable.106-108 The pattern can be progressed by constraining the upper extremities to force reliance on the lumbopelvic and foot/ankle strategies for balance. Head and/or trunk turns can be added to simulate the external focus of control that is required with movement in soccer. These should progress from slow to fast and anticipated to unanticipated as the player demonstrates competence in maintaining stability at each segment within the foundational stance position. Once this is in play, a ball should be introduced into the drills. As the player maintains the foundational position with vision diminished and upper extremities constrained, they should attempt to reach for or trap a ball from this position. If vision is completely obstructed, then the player can be instructed to open his/her eyes just as the ball arrives to induce a reactive response. Again, quality repetitions are essential for learning to occur, and subsequent skill acquisition to take place in the CNS; thus, close scrutiny should be paid to the qualitative essence of the movement patterns to ensure pristine biomechanics during this phase.
The “lower quarter dynamic stability phase” (Table 1) should continue with the same neuromotor training principles employed in the previous phase, except that the drills will now involve plyometrics. The player should ultimately progress from double-leg, to single-leg jumps and then linear to diagonal. Vision should still be obstructed and upper extremities constrained to channel the lumbopelvic region for force production and balance. Movement quality in the foundational position remains paramount with these drills to ensure that skill acquisition is occurring and injury risk is being mitigated. An external focus of control can be introduced by applying an unanticipated perturbation during a jump. Additional learning opportunities should include unanticipated head and trunk turns while landing in a unipedal stance from a jump. The task can be made more specific by having the player trap a pass while doing linear or diagonal single-leg hop progressions. In this manner, the player’s CNS can become reorganized to program the requisite synergies to maintain a protective foundational position on the stance leg, as the contralateral limb is required to perform work that is far outside the player’s base of support.
Continue to: The final segment of the CNS neuromotor rehabilitation program...
The final segment of the CNS neuromotor rehabilitation program is the “lower quarter dynamic agility phase” (Table 1), when the player will learn to perform an unanticipated directional change in a foundational position for the pivot leg. The player can begin this phase by initiating sprint-deceleration-pivot efforts, progressing at 45°, 90°, 120°, and 180° turns. This should be trained in both a forward and backpedal position. Close attention should be paid to the deceleration phase of the sprint-pivot effort, as this will set the player up to demonstrate protective kinematics during the pivot phase of the task. In this phase, the center of mass should become lower and move posteriorly, so that a deeper knee and hip flexion angle, supported by posterior chain synergies, can occur at the pivot point. This is an important skill for the player to acquire, as Cortes and colleagues92 have reported that female collegiate soccer players tend to perform a pivoting task with a more erect trunk position. In the same cohort, they also measured the mean knee flexion angle at initial contact during pivoting to be 24°.92 Movement patterns that reflect an elevated center of mass, with arms abducted away from the trunk, should be discouraged here. The drills can be progressed to have the player react to a command and perform unanticipated pivots within a 5 × 5-meter box to simulate defending. This should be progressed from eyes open and arms unconstrained, to vision disrupted and arms constrained. From here, an external focus of control can be added by playing a ball to the athlete. Vision should be withheld until the instant that the ball arrives at the player, when he/she is required to play the ball to an unanticipated spot. As is the case in all other phases of the neuromotor training, the quality of movement is the most important parameter to critique with each drill. From a qualitative standpoint, the player should demonstrate stiffness throughout the thoracolumbar region and power and control through the pelvis with each directional change. In addition, he or she should maintain a low and posteriorly oriented center of mass to optimize leverage in the hamstrings/gluteals compared with the quadriceps and reduce posterior ground reaction forces.
PSYCHOLOGICAL READINESS FOR RETURN TO PLAY
After an injury is sustained, an athlete is often subjected to a range of psychological responses in addition to the functional impairment, including stress, hesitancy, alterations in self-esteem, depression, fear of re-injury, and anxiety.43,109-111 The aforementioned responses are often at their height in the time immediately following an injury and generally subside over time during the rehabilitation process.110 The rates at which athletes experience psychological distress following an injury range between 5% and 19%; the levels are comparable with patients receiving treatment for mental health illness.43 However, these elements may persist, or even increase, in the later stages of the rehabilitation process as the topic of return to play is deliberated.112,113 If these fears are left unresolved, then a significant delay can be incurred during the rehabilitation process, which might ultimately jeopardize the successful return to play.114,115
When athletes have been cleared to return to sport, fear tends to be the most common reason for their decision to not return to play.21,116 The persistence of fear has clinical implications and warrants close monitoring to ensure that the athlete feels adequately supported in the decision to return to sport.117,118 Building the athlete’s confidence by addressing hesitation, lack of confidence, heightened awareness of risk or re-injury, and safe reintegration into athletic participation are important themes identified to encourage a safe return to play.43 A variety of validated tools can be integrated into an existing return-to-play decision-making algorithm (Table 2).118-120
Abbreviations: ACL, anterior cruciate ligament; CNS, central nervous system; CIMT, constraint-induced movement therapy.
Table 2. Self-Report Measurement Tools to Integrate into Return-to-Play Decision-Making Algorithm
Self-report Measurement Tools |
13-item Tampa Scale for Kinesiophobia |
Anterior Cruciate Ligament Return to Sport after Injury Scale (ACL-RSI) |
Global Rating Scale (GRS) |
International Knee Documentation Committee (IKDC) Subjective Knee Evaluation Form |
Knee Injury and Osteoarthritis Outcome Score (KOOS) |
Lysholm Knee Scoring Scale |
Short Form-36 Health Survey (SF-36) |
Subjective Patient Outcome for Return to Sport (SPORTS) |
Patient Health Questionnaire-9 |
By integrating the necessary screening of patients for kinesiophobia and assessing patient expectations after enduring an ACL injury, clinicians may be able to identify patients who are at risk for poorer functional outcomes. A consideration of psychosocial elements such as activity avoidance, fear of movement and re-injury, loss of confidence and expectations/assumptions during the continuum of the rehabilitation process, and the decision to return to play may favorably impact the individual’s ability to safely return to sport. It is critical to address both the physical and psychosocial factors during the rehabilitation process to more optimally transition individuals back to their prior level of athleticism.
CONCLUSION
Psychosocial factors may play a role in determining a player’s readiness to return to sport, as well as a potential for re-injury. A number of tests are available for use with this patient population to identify mental deficits that may impact player performance upon return. Additionally, the CNS should be considered as a source of impairment in players with ACL injuries. Current protocols may not fully appreciate the CNS’ impact on the player’s functional outcome. Therefore, an approach that includes CNS neuromotor training with traditional musculoskeletal rehabilitation, which also incorporates cognitive and psychosocial factors, may define an improved paradigm for treating soccer athletes following an ACL injury and assessing return-to-play capability.
ABSTRACT
Soccer players recovering from anterior cruciate ligament (ACL) injuries have better options for treatment today than they did 25 years ago. Surgical techniques have improved, and rehabilitation protocols have evolved considerably. Although the rehabilitation community is doing a better job of treating this patient population, the evidence does demonstrate that both re-injury and return- to-play (RTP) rates are still suboptimal. Most protocols focus on normalizing strength and range of motion (ROM) and achieving limb symmetry with soccer-specific movements. While these factors are certainly prerequisites for returning to the field, their inclusion does not provide a complete picture of the athlete’s presentation. An additional factor that should be prioritized with this patient population is the central nervous system (CNS). Advanced imaging has shown that peripheral deafferentation does occur with musculoskeletal injuries; this ultimately results in cortical reorganization, which makes movement planning more difficult for the player, since simpler tasks must now be processed at higher levels in the CNS. The evidence also shows that the CNS demonstrates plasticity in these cases, so that through focused neuromotor rehabilitation techniques, it is possible to bring movement planning back down to a sub-cortical level. Cognitive issues may also be a factor in preventing the player from returning. Fear of re-injury and diminished confidence can influence the way the player moves on the field, and diminish ability to demonstrate protective kinematics with all soccer-specific tasks. We believe that an approach incorporating traditional musculoskeletal rehabilitation, CNS neuro-motor training, and consideration for cognitive factors, may define an improved paradigm for treating the soccer player and assessing readiness for RTP following ACL injury.
Continue to: Although anterior cruciate ligament (ACL)...
Although anterior cruciate ligament (ACL) rehabilitation has evolved considerably over the past 2 decades, the basic paradigm has remained consistent: normalize strength and range of motion, reduce swelling and pain, achieve limb symmetry with functional tasks, and return to sport-specific activities gradually over a 6 to 12-month period. There have been some slight additions to this basic premise, such as evaluating knee and hip mechanics in the frontal plane, but the requirements here are vaguely defined and are typically only evaluated within the context of controlled clinical testing.
It is interesting to note that the typical ACL injury pattern occurs during a normal sport-specific movement, yet most rehabilitation protocols fail to recognize the potential causes of the aberrant movement pattern and how to best modify it so that the risk of repeated stress to the ACL can be minimized. It should be understood that movement occurs through the interaction of 3 discrete factors: the individual, the task being performed, and the environment in which it is performed.1 All of these factors will play a role in how the final movement pattern is produced. For example, a soccer player (individual) may backpedal and pivot to the left 60° and accelerate to sprint after a player moving towards the touchline (task) while receiving instructions from teammates and monitoring the movements of opposing players (environment). A small variance in any 1 of these factors could significantly impact the movement pattern as the player completes the task.
In most rehabilitation programs, each of these factors may be treated in a singular, non-specific manner, but if these factors are not coordinated effectively throughout the program to produce the desired sport-specific movement, a faulty pattern may persist, leaving the player at risk for injury. Current rehabilitation programs seem to have a strong focus on creating stability, mobility, and strength, but these are trained in silos, with an internal focus of control, which only solves the biomechanical equation. Often, it is difficult for the player to coordinate good biomechanics into an efficient, protective movement pattern that is specific to the tasks performed on the field during the normal course of play. The missing link here is the central nervous system (CNS).
Limitations to the current ACL protocols may be that they rely heavily on musculoskeletal rehabilitation and that they have limited emphasis on neurological rehabilitation. As will be discussed later, the CNS has a large impact on the final movement selected by the player. In fact, cognition, perception, and action are the three factors that comprise the individual’s part of the movement paradigm,1 yet rarely are these factors addressed in most ACL rehabilitation programs. These elements are a large part of the movement equation, so it is easy to understand how failing to address these features can lead to poor movement quality and subsequent ACL re-injury.
In addition to central neurological factors, cognitive issues may play a role in the player’s ability to return to sport. Determining optimal readiness for return to play is a difficult task for the medical community, with many variables to consider. Previous research studies have assessed the variability in return to play for various sports, including football, rugby, soccer, skiing, running, and tennis, with return-to-play rates ranging from 18% to 100%.2,3,4-10 The risk of secondary injury may cast doubt and fear on athletes as they contemplate their successful return to play.8,11 Although robust functional testing has become commonplace for determining athlete readiness after injury,12-20 the assessment of psychological readiness, persistent fear, and loss of confidence are often neglected and not as commonly integrated into the return-to-play algorithm.21-24 The purpose of this paper is to assess the various cognitive and central neural factors affecting a soccer player’s ability to recover from an ACL injury and offer suggestions for integrating treatments into the protocols to address these issues.
Continue to: CENTRAL NERVOUS SYSTEM NEUROPLASTICITY...
CENTRAL NERVOUS SYSTEM NEUROPLASTICITY
Despite the vast amount of attention and research focused on the ACL, the re-injury rate still remains quite high. It has been reported that rehabilitation programs that employ traditional neuromotor training produce a re-injury rate as high as 30% after the athlete returns to sport.25-28 The overall rate of sustaining a second ACL injury is 15%11 in all patient populations. For the general population <25 years of age, the re-injury rate is 21%, and for athletes <25 years of age, the re-injury rate rises to 23%.11 With re-injury rates at this level, it is certainly fair to consider and be critical of the current rehabilitation methods being used with this population. One opportunity for improvement lies in the general approach used to rehabilitate ACL-injured patients. Therapy for this injury is protocol-driven, and the fact remains that most protocols prioritize restoration of peripheral systems, with minimal thought given to the cortical control necessary to manage those systems.29,30 When neural factors are considered, it is usually within the context of increasing strength, balance, power, and biomechanical control,31-34 which are certainly important but peripheral factors nonetheless. The missing element in many ACL protocols may be how to best manage the central neural components and cognitive factors associated with this injury.
If the CNS were to receive more consideration in ACL protocols, the opportunity for improved outcomes could be substantial because the CNS has been proven to be a very malleable system, as long as it receives the correct input. The CNS demonstrates neuroplasticity,35 which means that it is capable of reorganization, based on the stimuli that it receives, whether internal or external.36
This is an important consideration in ACL rehabilitation because the ACL graft, while restoring the biomechanical properties to the knee, is not fully capable of producing the same neurosensory properties of the original ACL.37-42This is an important concept to understand because an ACL tear does indeed cause deafferentation in the ascending pathways to the brain.37-40,42-46 This can lead to CNS reorganization and subsequent alterations in efferent output to the periphery.37-40,42-46 Therefore, if a protocol with traditional musculoskeletal principles was used, then the mechanical function of the knee may certainly be remediated, but the neurosensory function will remain in a maladaptive state,47-50 potentially leading to aberrant, non-protective movement strategies and a higher risk of re-injury.
The process of CNS reorganization may begin with the initial ACL injury. A peripheral musculoskeletal injury creates an inflammatory response that results in the arrival of chemical mediators such as histamine, substance P, calcitonin, and calcitonin gene-related peptide at the site of injury.51 As edema accumulates in the joint, tension is applied to the capsule, which may adversely affect proprioception from the receptors located within.45 The interruption of consistent input from the peripheral mechanoreceptors may lead to long-term differentiation of the ascending pathways.52 This information is synthesized at 3 different levels of the CNS (spinal cord, brain stem, and motor cortex) to produce motor output.53-56 Differentiation in the ascending circuitry can cause inhibition of motor neurons at the spinal cord.45Animal research has shown that this differentiation can cause a breakdown in the cuneate nucleus of the brainstem,57which provides sensory information from the upper body, while the gracile nucleus does the same for the lower body. These structures transfer proprioceptive input to the ventral posterior lateral nucleus in the thalamus, where it is then sent to the primary somatosensory cortex.57 In general, the somatosensory, visual, and vestibular systems interpret afferent inputs to control movement, balance, and stability.58,59 In a sport like soccer, where the movement tasks are dynamic and unpredictable, it is easy to see why even a slight deficit in somatosensory processing could disrupt a movement. Valeriani and colleagues42,46 showed that somatosensory-evoked potentials were indeed altered in a cohort of ACL reconstruction (ACLR) subjects, indicating reorganization within the CNS. Additionally, the deafferentation could not be changed by other afferent input coming from the knee or by the new ACL graft placed in the knee.42,46The primary motor cortex has been found to have a substantial network of connectivity with the primary somatosensory cortex, which supports the theory that the motor cortex has a very strong linkage with the peripheral receptors in the joint.60 The ligaments in the joint contain Ruffini, Pacinian, and Golgi receptors, all of which react to changes in the collagen fibers and send information regarding tension, length, speed, acceleration, position, and movement back to the CNS.61-64 Unfortunately, the ascending pathway deafferentation can cause reorganization within the CNS, which makes the feedback provided from the periphery less effective in motor planning.
Ward and colleagues65 have reported that reorganization within the motor cortex is the primary cause of chronic neuromuscular movement deficits in peripheral joint injuries. Researchers have used functional magnetic resonance imaging, transcranial magnetic stimulation, and electroencephalography in ACL patients to demonstrate changes in cortical activity and subsequent CNS reorganization.65 Kapreli and colleagues41 reported that subjects with an ACL injury demonstrated higher cortical activation in the pre-supplementary motor area (pre-SMA). This is a region that is responsible for more complex motor planning.66,67 This area becomes active before the primary motor cortex and is responsible for preparing the final movement pattern that the motor cortex executes.41 As the task becomes more complex, activity in the pre-SMA will increase.41 Additionally, they found that the posterior secondary somatosensory area and posterior inferior temporal gyrus showed increased cortical activity compared with controls.41 Visual planning is processed in the posterior inferior temporal gyrus, and so, it appears that the difficulty in processing somatosensory information due to ascending pathway deafferentation places an increased reliance on the visual system for movement planning.68-70 This was observed while ACL-injured subjects performed a simple knee flexion-extension movement encompassing 40°, indicating the need to incorporate higher central levels of planning for a very simple movement pattern.41 Baumeister and colleagues37,38 also showed that subjects with ACLR had higher levels of cortical activation in the areas of the brain that require attention and that process sensory input. They theorized that this occurred because of reduced efficiency of neural processing at lower levels in the CNS. Despite the higher levels of cortical activity observed, they found that subjects with an ACLR demonstrated proprioceptive testing that was deficient compared with that of controls. Heroux and Tremblay71 also demonstrated that subjects with an ACLR had increased resting motor cortex activity. They believed that this occurred as the motor cortex attempted to maintain neuromotor output to the periphery in the face of diminished afferent input.
Continue to: The reorganization that results in movement planning...
The reorganization that results in movement planning, transitioning from subcortical levels to cortical levels, is a phenomenon that researchers believe can lead to deficiencies even as the athlete has returned to sport. Grooms et al72revealed in a case report that a subject with an ACLR showed higher levels of activity in the crus region of the cerebellum. This area contains corticobulbar and corticospinal tracts that transmit neural input to maintain balance and coordination.73 These changes in the cerebellum, combined with increased motor cortex activity, are thought to be indicative of a global neural strategy that uses higher levels of the CNS, as opposed to subcortical processing.72
The current research makes a clear and compelling argument for the importance of CNS reorganization after an ACL injury, placing increased reliance on higher cortical levels of control, as well as the visual system to coordinate balance and movement. It is thought that this reorganized method of neural transmission can then become imprinted within the CNS, if not corrected.35,74 If this is the case, then traditional strength programs may not be sufficient to restore these connections to their pre-injury level. If the CNS has the ability to reorganize based on the aberrant input that it receives from the periphery, then it also certainly has the potential to adapt to more specific structured input via the ascending afferent pathways.41,45 The rehabilitation program, however, needs to be structured specifically to target the reorganized regions of the brain. There needs to be an emphasis on rehabilitating not only the peripheral neuromotor structures but also the CNS.75
CENTRAL NERVOUS SYSTEM REHABILITATION PRINCIPLES
For a neurological rehabilitation to be successful, the interventions need to be repetitive and task-specific, involve learning, employ whole and part practice, and transition from using an internal to an external focus of control.76Movements that are repetitive, but which lack structured learning and skill, have been shown to have no effect on inducing neuroplastic changes in the primary motor cortex.77,78 However, using neurological rehabilitation techniques that facilitate the acquisition of new motor skills by the CNS have been shown to cause neuroplastic adaptation in the motor cortex.79-85 This occurs because neuroplasticity is determined by experience and practice.78 The CNS operates on cues received in the ascending tracts by mechanoreceptors in the joint. If a new movement pattern is being learned by the athlete, then this new afferent input received from the periphery will start to initiate reorganization in the higher learning centers. If this occurs with optimal repetition and precision, then a positive reorganization can take place within the CNS that results in a higher percentage of motor planning and control being filtered down to a subcortical level. Essentially, the movements become instinctive, which is crucial in athletics, where attention in higher cortical areas is frequently diverted to external aspects of the competition and not solely used to focus on movement.
This is why shifting neurological rehabilitation from an internal focus of control to an external focus of control is paramount. While using an internal focus of control is required early in rehabilitation to enable the athlete to understand the specific tasks required in a composite movement, a gradual transition to an external focus of control is necessary as the athlete begins to perform tasks that are more soccer-specific. This autonomous stage of motor learning is crucial because it transfers the burden of motor planning from higher to lower levels of the CNS and frees up the pre-SMA and primary motor cortex to handle more complex patterns.58,86-88
Continue to: ANTERIOR CRUCIATE LIGAMENT RISK POTENTIAL IN SOCCER PLAYERS...
ANTERIOR CRUCIATE LIGAMENT RISK POTENTIAL IN SOCCER PLAYERS
If a comprehensive neuromotor rehabilitation program is to be used effectively with soccer athletes, then the first priority is to define how the players should move, so that they can demonstrate protective kinematics with all soccer-specific tasks and minimize stress to the ACL. As the ideal movement pattern becomes autonomous, then it should be trained within the context of a dynamic environment; remembering that environmental changes have a large impact on the final movement pattern selected by the individual. Brophy et al89 evaluated videos of non-contact ACL injuries in male and female soccer players and determined that 45% occurred while cutting, 25% while landing, and 16% during deceleration. These 3 patterns represent 86% of the ACL injuries observed and offer an opportunity for evaluation and treatment with specific central neuromotor rehabilitation techniques.
The foundational movement patterns for the soccer player should focus on producing leverage that minimizes stress to the ACL during the 3 primary tasks outlined above. To achieve this, it is necessary to reduce posterior ground reaction forces at the hip and knee joint during these movements. There is a high correlation between the magnitude of the posterior ground reaction force, and anterior tibial shear, and subsequent displacement.90,91 This stress can be reduced by increasing the hip and knee flexion angles during soccer-specific movements that involve pivoting, decelerating, and landing from a jump in a unipedal stance.92
This phenomenon can be explained by observing changes in the ACL elevation angle, hamstring insertion angle, and patella tendon-tibial tuberosity insertion angle. As the knee moves into flexion, the ACL takes on a more parallel orientation to the tibia, and its fibers are better able to resist elastic deformation accompanied by a posterior ground reaction force.93,94 The quadriceps will produce less anterior translation on the tibia because the patella tendon insertion angle is reduced relative to the longitudinal axis of the tibia, and the mechanical advantage of the quadriceps is decreased.95 Lastly, the hamstrings will be able to provide better leverage posteriorly because the resultant force trends toward a more parallel orientation to the tibial plateau, which enables the player to counter, more effectively, the posterior ground reaction force and the anterior pull directed by the quadriceps.95
This theory is supported by the work of Li and colleagues,96 who showed that there is an inverse relationship between knee flexion angle and ACL loading. In their study, they applied a constant quadriceps force of 200 N at 15°, 30°, and 60° angles. The anterior shear force was obviously the highest at 15° and reduced by 20% at 30° and 60% at 60°. When hamstrings co-contraction was added, there was an additional 30% reduction in anterior shear at 15° and 50% at 30° and 60°. From a more flexed position, the hamstrings can increase joint compression and reduce the anterior translation by allowing the concave medial tibial plateau to limit the anterior drawer effect and absorb the forces that occur with excessive anterior shear, internal rotation, and valgus loads.97 As the knee flexion angle approximates 60°, the hamstring leverage is increased, and the quadriceps leverage is diminished to the point where its ability to produce anterior tibial translation is neutralized.98 Daniel and colleagues98 referred to this as the quadriceps neutral angle.
For soccer-specific movements that are potentially injurious to the ACL, it may then be beneficial to create a default movement pattern at the knee that approximates this value. In keeping with the information presented in this paper, it will be important to have the player reproduce this angle consistently during activities that involve pivoting, decelerating, and landing from a jump within the context of match play. This will certainly require that segments located both proximal and distal to the knee are able to function within specific parameters so that a cohesive protective synergy is produced throughout the lower quarter which minimizes posterior ground reaction forces and is protective of the ACL. This is where structured neuromotor training that is able to modulate networks within the CNS may be beneficial.
Continue to: CENTRAL NERVOUS SYSTEM TREATMENT TECHNIQUES FOR THE SOCCER PLAYER...
CENTRAL NERVOUS SYSTEM TREATMENT TECHNIQUES FOR THE SOCCER PLAYER
The ultimate goal is to create a foundational movement pattern that optimizes leverage and is protective of the ACL during decelerating, pivoting, and landing in a unipedal stance from a jump. The composite segments that are necessary to achieve this include local core stability to create lumbopelvic stiffness, and global core activation to enhance posterior chain stability. This should enable the player to feel more balanced when placing the pelvis in a more posterior and inferior position while still maintaining the trunk in a position that is parallel with the tibia, as the knee is flexed to an approximate 60° angle (Figure 1). From a frontal plane perspective, the acetabulum should bisect the malleoli of the stance leg, with a neutral tibiofemoral joint alignment (Figure 1).
The neuromotor training for the composite segments of this movement can begin in the “acute postoperative phase” (Table 1). Because the surgical repair will limit the player’s capabilities in this stage, this is a good time to break down the foundational movement pattern into its component parts and ensure that the CNS receives a high number of quality repetitions of parts. In this phase, the player may begin an isolated training for the transversus abdominis, multifidus, and pubococcygeus. This can start in a supine position using biofeedback with an isometric contraction, progress to a standing position, and incorporate deep core activation with stance-phase gait training, mini squats, and lunge variations. This phase will require an abundance of visual and verbal feedback with an internal focus of control as the player gets used to activating the deep core and quad/hip synergy during functional lower extremity movements. Even in this early phase, the player should look to minimize anterior and/or posterior pelvic tilting and maintain a stiff thoracolumbar segment that remains parallel with the tibia during all functional movements.
Table 1. Adjunct CNS Treatment Principles for ACL Reconstruction in Soccer Athletes
Phase | Goal | CNS Rehabilitation Techniques |
Acute postoperative | Local core activation with weightbearing exercise. Produce trunk stiffness with lower extremity movements. | High repetitions. Verbal or tactile cues. Internal focus of control. Partial practice. |
Subacute postoperative | Lumbopelvic, foot or ankle, and posterior chain segments learn to participate in movement effectively. | Requires higher levels of cortical planning. Internal focus of control. CIMT. |
Static stability | Able to adopt foundational movement pattern consistently with vision eliminated. | Somatosensory vs visual processing. Partial-to-whole practice. Use internal and/or external focus of control. |
Dynamic stability | Able to perform plyometrics in a single-leg position using foundational movement pattern with subcortical processing. | Increase velocity with movement challenges. Occlude or eliminate vision. Heavy reliance on an external focus of control. Prioritize movement quality. |
Dynamic agility | Pivoting, decelerating, and landing are performed with hip flexion/knee flexion synergy, trunk stiffness, and posterior chain activation. | Unanticipated movement challenges. Whole practice. Ball reaction drills with vision obstructed or occluded. Contact drills with vision obstructed or occluded. Use external focus of control to include soccer-specific tasks. |
Abbreviations: ACL, anterior cruciate ligament; CNS, central nervous system; CIMT, constraint-induced movement therapy.
As the player moves into the “subacute postoperative phase” (Table 1). He or she will continue to use an internal focus of control to activate the local/global core synergy with functional movements progressing from double-leg, to single-leg positions. Partial practice, instead of whole practice, is still the predominant theme of the neural training process. In this phase, the knee and hip flexion angles can increase, and the player’s trunk and pelvic position should be critiqued in a single-leg position so that the trunk remains parallel with the tibia in the sagittal plane with a slight forward hip hinge. The pelvis should remain level throughout single-leg stance to ensure adequate activation of the lateral hip stabilizers. This is the stage where the player can learn to isolate closed kinetic chain hip rotation for pivoting, and so, single-leg hip internal and external rotation drills are useful, both with and without resistance. Skill acquisition is crucial in this phase because the patterns that the CNS adopts will form the foundation for more dynamic patterns that will occur in the later soccer-specific stages. Higher levels of cortical planning are still needed in this phase. For this reason, it is important that poor quality repetitions are recognized by the player and clinician so that he or she can learn to perform them correctly, albeit still with an internal focus of control. This is also a good time to begin to employ constraint-induced movement therapy as the player is able to replicate the desired pattern with more precision. For example, by eliminating the use of the upper extremities as a source of balance, the CNS is forced to program alternate synergies such as the lumbopelvic, and foot and ankle segments to maintain the desired alignment.
The “lower quarter static stability phase” (Table 1) marks a point where it may be useful to use direct strategies that have the capability to change CNS efferent owutput from a primary reliance on the visual processing areas in the posterior-inferior temporal gyrus back to the somatosensory area. It is critical that the player is able to make this transition in cortical reorganization and control, because ACL-injured subjects have been shown to have balance scores similar to healthy controls when they are able to use their vision, but this is reduced when vision is taken away.99-105 Their balance will diminish even further if vision is modulated during more complex landing and pivoting maneuvers.99-105 This may certainly explain why defending is a riskier task for ACL-injured players89 as their visual system is focused more on tracking a player than attending to precision with movement planning.
To enhance this cortical reorganization within the context of soccer-specific movements, it is useful to start from a foundational single-leg position, with the knee approximating 60° of flexion and the trunk parallel to the tibia. In the frontal plane, the pelvis should be level, the trunk vertical, and the acetabulum bisecting the malleoli of the stance leg (Figure 1). The player may initially work on getting into this foundational position with vision either partially obstructed using stroboscopic eyewear or completely obstructed if this equipment is unavailable.106-108 The pattern can be progressed by constraining the upper extremities to force reliance on the lumbopelvic and foot/ankle strategies for balance. Head and/or trunk turns can be added to simulate the external focus of control that is required with movement in soccer. These should progress from slow to fast and anticipated to unanticipated as the player demonstrates competence in maintaining stability at each segment within the foundational stance position. Once this is in play, a ball should be introduced into the drills. As the player maintains the foundational position with vision diminished and upper extremities constrained, they should attempt to reach for or trap a ball from this position. If vision is completely obstructed, then the player can be instructed to open his/her eyes just as the ball arrives to induce a reactive response. Again, quality repetitions are essential for learning to occur, and subsequent skill acquisition to take place in the CNS; thus, close scrutiny should be paid to the qualitative essence of the movement patterns to ensure pristine biomechanics during this phase.
The “lower quarter dynamic stability phase” (Table 1) should continue with the same neuromotor training principles employed in the previous phase, except that the drills will now involve plyometrics. The player should ultimately progress from double-leg, to single-leg jumps and then linear to diagonal. Vision should still be obstructed and upper extremities constrained to channel the lumbopelvic region for force production and balance. Movement quality in the foundational position remains paramount with these drills to ensure that skill acquisition is occurring and injury risk is being mitigated. An external focus of control can be introduced by applying an unanticipated perturbation during a jump. Additional learning opportunities should include unanticipated head and trunk turns while landing in a unipedal stance from a jump. The task can be made more specific by having the player trap a pass while doing linear or diagonal single-leg hop progressions. In this manner, the player’s CNS can become reorganized to program the requisite synergies to maintain a protective foundational position on the stance leg, as the contralateral limb is required to perform work that is far outside the player’s base of support.
Continue to: The final segment of the CNS neuromotor rehabilitation program...
The final segment of the CNS neuromotor rehabilitation program is the “lower quarter dynamic agility phase” (Table 1), when the player will learn to perform an unanticipated directional change in a foundational position for the pivot leg. The player can begin this phase by initiating sprint-deceleration-pivot efforts, progressing at 45°, 90°, 120°, and 180° turns. This should be trained in both a forward and backpedal position. Close attention should be paid to the deceleration phase of the sprint-pivot effort, as this will set the player up to demonstrate protective kinematics during the pivot phase of the task. In this phase, the center of mass should become lower and move posteriorly, so that a deeper knee and hip flexion angle, supported by posterior chain synergies, can occur at the pivot point. This is an important skill for the player to acquire, as Cortes and colleagues92 have reported that female collegiate soccer players tend to perform a pivoting task with a more erect trunk position. In the same cohort, they also measured the mean knee flexion angle at initial contact during pivoting to be 24°.92 Movement patterns that reflect an elevated center of mass, with arms abducted away from the trunk, should be discouraged here. The drills can be progressed to have the player react to a command and perform unanticipated pivots within a 5 × 5-meter box to simulate defending. This should be progressed from eyes open and arms unconstrained, to vision disrupted and arms constrained. From here, an external focus of control can be added by playing a ball to the athlete. Vision should be withheld until the instant that the ball arrives at the player, when he/she is required to play the ball to an unanticipated spot. As is the case in all other phases of the neuromotor training, the quality of movement is the most important parameter to critique with each drill. From a qualitative standpoint, the player should demonstrate stiffness throughout the thoracolumbar region and power and control through the pelvis with each directional change. In addition, he or she should maintain a low and posteriorly oriented center of mass to optimize leverage in the hamstrings/gluteals compared with the quadriceps and reduce posterior ground reaction forces.
PSYCHOLOGICAL READINESS FOR RETURN TO PLAY
After an injury is sustained, an athlete is often subjected to a range of psychological responses in addition to the functional impairment, including stress, hesitancy, alterations in self-esteem, depression, fear of re-injury, and anxiety.43,109-111 The aforementioned responses are often at their height in the time immediately following an injury and generally subside over time during the rehabilitation process.110 The rates at which athletes experience psychological distress following an injury range between 5% and 19%; the levels are comparable with patients receiving treatment for mental health illness.43 However, these elements may persist, or even increase, in the later stages of the rehabilitation process as the topic of return to play is deliberated.112,113 If these fears are left unresolved, then a significant delay can be incurred during the rehabilitation process, which might ultimately jeopardize the successful return to play.114,115
When athletes have been cleared to return to sport, fear tends to be the most common reason for their decision to not return to play.21,116 The persistence of fear has clinical implications and warrants close monitoring to ensure that the athlete feels adequately supported in the decision to return to sport.117,118 Building the athlete’s confidence by addressing hesitation, lack of confidence, heightened awareness of risk or re-injury, and safe reintegration into athletic participation are important themes identified to encourage a safe return to play.43 A variety of validated tools can be integrated into an existing return-to-play decision-making algorithm (Table 2).118-120
Abbreviations: ACL, anterior cruciate ligament; CNS, central nervous system; CIMT, constraint-induced movement therapy.
Table 2. Self-Report Measurement Tools to Integrate into Return-to-Play Decision-Making Algorithm
Self-report Measurement Tools |
13-item Tampa Scale for Kinesiophobia |
Anterior Cruciate Ligament Return to Sport after Injury Scale (ACL-RSI) |
Global Rating Scale (GRS) |
International Knee Documentation Committee (IKDC) Subjective Knee Evaluation Form |
Knee Injury and Osteoarthritis Outcome Score (KOOS) |
Lysholm Knee Scoring Scale |
Short Form-36 Health Survey (SF-36) |
Subjective Patient Outcome for Return to Sport (SPORTS) |
Patient Health Questionnaire-9 |
By integrating the necessary screening of patients for kinesiophobia and assessing patient expectations after enduring an ACL injury, clinicians may be able to identify patients who are at risk for poorer functional outcomes. A consideration of psychosocial elements such as activity avoidance, fear of movement and re-injury, loss of confidence and expectations/assumptions during the continuum of the rehabilitation process, and the decision to return to play may favorably impact the individual’s ability to safely return to sport. It is critical to address both the physical and psychosocial factors during the rehabilitation process to more optimally transition individuals back to their prior level of athleticism.
CONCLUSION
Psychosocial factors may play a role in determining a player’s readiness to return to sport, as well as a potential for re-injury. A number of tests are available for use with this patient population to identify mental deficits that may impact player performance upon return. Additionally, the CNS should be considered as a source of impairment in players with ACL injuries. Current protocols may not fully appreciate the CNS’ impact on the player’s functional outcome. Therefore, an approach that includes CNS neuromotor training with traditional musculoskeletal rehabilitation, which also incorporates cognitive and psychosocial factors, may define an improved paradigm for treating soccer athletes following an ACL injury and assessing return-to-play capability.
- Shumway-Cook A, Woollacott MH Motor control: translating research into clinical practice. 4th ed: Lippincott, Williams and Wilkins, 2012.
- Ardern CL, Webster KE, Taylor NF, Feller JA. Return to the preinjury level of competitive sport after anterior cruciate ligament reconstruction surgery: two-thirds of patients have not returned by 12 months after surgery. Am J Sports Med. 2011;39(3):538-543. doi:10.1177/0363546510384798.
- Bauer M, Feeley BT, Wawrzyniak JR, Pinkowsky G, Gallo RA. Factors affecting return to play after anterior cruciate ligament reconstruction: a review of the current literature. Phys Sportsmed. 2014;42(4):71-79. doi:10.3810/psm.2014.11.2093.
- Eisenstein ED, Rawicki NL, Rensing NJ, Kusnezov NA, Lanzi JT. Variables afftecting return to play after anterior cruciate ligament injury in the national football league. Orthop J Sports Med. 2016;4(10):2325967116670117.
- Ellman MB, Sherman SL, Forsythe B, LaPrade RF, Cole BJ, Bach BR. Return to play following anterior cruciate ligament reconstruction. J Am Acad Orthop Surg. 2015;23(5):283-296. doi:10.5435/JAAOS-D-13-00183.
- Fabricant PD, Chin CS, Conte S, Conte S, Coleman SH, Pearle AD, Dines JS. Return to play after anterior cruciate ligament reconstruction in major league baseball athletes. Arthroscopy. 2015;31(5):896-900. doi:10.1016/j.arthro.2014.12.008.
- Morris RC, Hulstyn MJ, Fleming BC, Owens BD, Fadale PD. Return to play following anterior cruciate ligament reconstruction. Clin Sports Med. 2016;35:(4):655-668. doi:10.1016/j.csm.2016.05.009.
- Paterno MV, Rauh MJ, Schmitt LC, Ford KR, Hewett TE. Incidence of second ACL injuries 2 years after primary ACL reconstruction and return to sport. Am J Sports Med. 2014;42(7):1567-1573. doi:10.1177/0363546514530088.
- Sclafani MP, Davis CC. Return to play progression for rugby following injury to the lower extremity. A clinical commentary and review of the literature. Int J Sports Phys Ther. 2016;11(2):302-320.
- Walden M, Hagglund M, Magnusson H, Ekstrand J. ACL injuries in men’s professional football: a 15-year prospective study on time trends and return-to-play rates reveals only 65% of players still play at the top level 3 years after ACL rupture. Br J Sports Med. 2016;50(12):744-750. doi:10.1136/bjsports-2015-095952.
- Wiggins AJ, Grandhi RK, Schneider DK, Stanfield D, Webster KE, Myer GD. Risk of secondary injury in younger athletes after anterior cruciate ligament reconstruction: a systematic review and meta-analysis. Am J Sports Med. 2016;44(7):1861-1876. doi:10.1177/0363546515621554.
- Arundale AJ, Cummer K, Capin JJ, Zarzycki R, Snyder-Mackler L. Report of the clinical and functional primary outcomes in men of the ACL-SPORTS trial: similar outcomes in men receiving secondary prevention with and without perturbation training 1 and 2 years after ACL reconstruction. Clin Orthop Relat Res. 2017;475(10):2523-2534. doi:10.1007/s11999-017-5280-2.
- Brumitt J, HB, Manske RC, Niemuth PE, Rauh MJ. Lower extremity functional tests and risk of injury in division III collegiate athletes. Int J Sports Phys Ther. 2013;8:216-227.
- Cacolice PA, Carcia CR, Scibek JS, Phelps AL. The use of functional tests to predict sagittal plane knee kinematics in ncaa-d1 female athlets. Int J Sports Phys Ther. 2015;10(4):493-504.
- Goodstadt NM, Hunter-Giordano A, Axe MJ, Snyder-Mackler L. Functonal testing to determine readiness to discontinue brace use one year after acl reconstruction. Int J Sports Phys Ther. 2013;8(2):91-96.
- Herbst E, Hoser C, Hildebrandt C, Raschner C, Hepperger C, Pointner H, Fink C. Functional assessments for decision-making regarding return to sports following ACL reconstruction. Part ll: clinical application of a new test battery. Knee Surg Sports Traumatol Arthrosc. 2015;23(5):1283-1291. doi:10.1007/s00167-015-3546-3.
- Hoog P, Warren M, Smith CA, Chimera NJ. Functional hop tests and tuck jump assessment scores between female division l collegiate athletes participating in high versus low acl injury prone sports: a cross sectional analysis. Int J Sports Phys Ther. 2016;11(6):945-953.
- Mohammadi F, Salavati M, Akhbari B, Mazaheri M, Mohsen Mir S, Etemadi Y. Comparison of functional outcome measures after ACL reconstruction in competitive soccer players: a randomized trial. J Bone Joint Surg, (Am.). 2013;95(14):1271-1277. doi:10.2106/JBJS.L.00724.
- Rambaud A, Samozino P, Edouard P. Functional tests can they help in the decision to return to sports after anterior cruciate ligament? Example with hop tests. Ann Phys Rehabil Med. 2016;59s:e19-ee20. doi:10.1016/j.rehab.2016.07.047.
- Xergia SA, Pappas E, Zampeli F, Georgiou S, Georgoulis AD. Asymmetries in functional hop tests, lower extremity kinematics, and isokinetic strength persist 6 to 9 months following anterior cruciate ligament reconstruction. J Orthop Sports Phys Ther. 2013;43(3):154-162. doi:10.2519/jospt.2013.3967.
- Ardern CL, Taylor NF, Feller JA, Webster KE. A systematic review of the psychological factors associated with returning to sport following injury. Br J Sports Med. 2013;47(17):1120-1126. doi:10.1136/bjsports-2012-091203.
- Ardern CL, Taylor NF, Feller JA, Whitehead TS, Webster KE. Psychological responses matter in returning to preinjury level of sport after anterior cruciate ligament reconstruction surgery. Am J Sports Med. 2013;41(7):1549-1558. doi:10.1177/0363546513489284.
- Christino MA, Fantry AJ, Vopat BG. Psychological aspects of recovery following anterior cruciate ligament reconstruction. J Am Acad Orthop Surg. 2015;23(8):501-509. doi:10.5435/JAAOS-D-14-00173.
- Naghdi S, Nakhostin Ansari N, Farhadi Y, Ebadi S, Entezary E, Glazer D. Cross-cultural adaptation and validation of the Injury-Psychological Readiness to Return to Sport scale to Persian language. Physiother Theory Pract. 2016;32(7):528-535. doi:10.1080/09593985.2016.1221486.
- Hui C, Salmon LJ, Kok A, Maeno S, Linklater J, Pinczewski LA. Fifteen-year outcome of endoscopic anterior cruciate ligament reconstruction with patellar tendon autograft for “isolated” anterior cruciate ligament tear. Am J Sports Med. 2011;39:89-98. doi:10.1177/0363546510379975.
- Paterno MV, Rauh MJ, Schmitt LC, Ford KR, Hewett TE. Incidence of contralateral and ipsilateral anterior cruciate ligament (ACL) injury after primary ACL reconstruction and return to sport. Clin J Sport Med. 2012;22:116-121. doi:10.1097/JSM.0b013e318246ef9e.
- Paterno MV, Schmitt LC, Ford KR, Rauh MJ, Myer GD, Huang B, Hewett TE. Biomechanical measures during landing and postural stability predict second anterior cruciate ligament injury after anterior cruciate ligament reconstruction and return to sport. Am J Sports Med. 2010;38:1968-1978. doi:10.1177/0363546510376053.
- Wright RW, Dunn WR, Amendola A, Andrish JT, Bergfeld J, Kaeding CC, Marx RG, McCarty EC, Parker RD, Wolcott M, Wolf BR, Spindler KP. Risk of tearing the intact anterior cruciate ligament in the contralateral knee and rupturing the anterior cruciate ligament graft during the first 2 years after anterior cruciate ligament reconstruction: a prospective MOON cohort study. Am J Sports Med. 2007;35:1131-1134. doi:10.1177/0363546507301318.
- Bystrom MG, Rasmussen-Barr E, Grooten WJ. Motor control exercises reduces pain and disability in chronic and recurrent low back pain: a meta-analysis. Spine. 2013;38(6):E350-E358. doi:10.1097/BRS.0b013e31828435fb.
- Macedo LG, Maher CG, Latimer J, McAuley JH. Motor control exercise for persistent nonspecific low back pain: a systematic review. Phys Ther. 2009;89(1):9-25. doi:10.2522/ptj.20080103.
- Grindstaff TL, Hammill RR, Tuzson AE, Hertel J. Neuromuscular control training programs and noncontact anterior cruciate ligament injur rates in female athletes: a numbers-needed-to-treat analysis. J Athl Train. 2006;41:450-456.
- Myer GD, Ford KR, Brent JL, Hewett TE. An integrated approach to change the outcome part II: Targeted neuromuscular training techniques to reduce identified ACL injury risk factors. J Strength Cond Res. 2012;26:2272-2292. doi:10.1519/JSC.0b013e31825c2c7d.
- Myer GD, Paterno MV, Ford KR, Hewett TE. Neuromuscular training techniques to target deficits before return to sport after anterior cruciate ligament reconstruction. J Strength Cond Res. 2008;22:987-1014. doi:10.1519/JSC.0b013e31816a86cd.
- Yoo JH, Lim BO, Ha M, Lee SW, Oh SJ, Lee YS, Kim JG. A meta-analysis of the effect of neuromuscular training on the prevention of the anterior cruciate ligament injury in female athletes. Knee Surg Sports Traumatol Arthrosc. 2010;18:824-830. doi:10.1007/s00167-009-0901-2.
- Moseley GL, Flor H. Targeting cortical representations in the treatment of chronic pain; a review. Neurorehabil Neural Repair. 2012;26(6):646-652. doi:10.1177/1545968311433209.
- Cramer SC. Brain repair after stroke. New Engl J Med 2010;362(19):1827-1829. doi:10.1056/NEJMe1003399.
- Baumeister J, Reinecke K, Weiss M. Changed cortical activity after anterior cruciate ligament reconstruction in a joint position paradigm: an EEG study. Scand J Med Sci Sports. 2008;18:473-484. doi:10.1111/j.1600-0838.2007.00702.x.
- Baumeister J, Reinecke K, Schubert M, Weiss M. Altered electrocortical brain activity after ACL reconstruction during force control. J Orthop Res. 2011;29:1383-1389. doi:10.1002/jor.21380.
- Courtney C, Rine RM. Central somatosensory changes associated with improved dynamic balance in subjects with anterior cruciate ligament deficiency. Gait Posture. 2006;24:190-195. doi:10.1016/j.gaitpost.2005.08.006.
- Courtney C, Rine RM, Kroll P. Central somatosensory changes and altered muscle synergies in subjects with anterior cruciate ligament deficiency. Gait Posture. 2005;22:69-74. doi:10.1016/j.gaitpost.2004.07.002.
- Kapreli E, Athanasopoulos S, Gliatis J, Papathanasiou M, Peeters R, Strimpakos N, Van Hecke P, Gouliamos A, Sunaert S. Anterior cruciate ligament deficiency causes brain plasticity: a functional MRI study. Am J Sports Med. 2009;37:2419-2426. doi:10.1177/0363546509343201.
- Valeriani M, Restuccia D, Di Lazaro V, Franceschi F, Fabbriciani C, Tonali P. Clinical and neurophysiological abnormalities before and after reconstruction of the anterior cruciate ligament of the knee. Acta Neurol Scand. 1999;99:303-307. doi:10.1111/j.1600-0404.1999.tb00680.x.
- Burland JP, Toonstra J, Werner JL, Mattacola CG, Howell DM, Howard JS. Decision to return to sport after anterior cruciate ligament reconstruction, Part 1: A qualitative investigation of psychosocial factors. J Athl Train. 2018;53(5):452-463. doi:10.4085/1062-6050-313-16
- Johansson H, Sjolander P, Sojka P. A sensory role for the cruciate ligaments. Clin Orthop Relat Res. 1991;268:161-178.
- Kaprelli E, Athanasopoulos S. The anterior cruciate ligament deficiency as a model of brain plasticity. Med Hypo. 2006;67:645-650. doi:10.1016/j.mehy.2006.01.063.
- Valeriani M, Restuccia D, Di Lazzaro V, Franceschi F, Fabbriciani C, Tonali P. Central nervous system modifications in patients with lesion of the anterior cruciate ligament of the knee. Brain. 1996;119(Pt 5):1751-1762. doi:10.1093/brain/119.5.1751.
- Nyland J, Fisher B, Brad E, Krupp R, Caborn DN. Osseous deficits after anterior cruciate ligament injury and reconstruction: a systematic review with suggestions to improve osseous homeostasis. Arthroscopy. 2010;26:1248-1257. doi:10.1016/j.arthro.2010.03.017.
- Nyland J, Klein S, Caborn DN. Lower extremity compensatory neuromuscular and biomechanical adaptations 2 to 11 years after anterior cruciate ligament reconstruction. Arthroscopy. 2010;26:1212-1225. doi:10.1016/j.arthro.2010.01.003.
- Paterno MV, Ford KR, Myer GD, Heyl R, Hewett TE. Limb asymmetries in landing and jumping 2 years following anterior cruciate ligament reconstruction. Clin. J Sports Med. 2007;17:258-262. doi:10.1097/JSM.0b013e31804c77ea.
- Wojtys EM, Huston LJ. Longitudinal effects of anterior cruciate ligament injury and patellar tendon autograft reconstruction on neuromuscular performance. Am J Sports Med. 2000;28:336-344. doi:10.1177/03635465000280030901.
- Levine JD, Dardick SJ, Basbaum AI, Scipio E. Reflex neurogenic inflammation. I. Contribution of the peripheral nervous system to spatially remote inflammatory responses that follow injury. J Neurosci. 1985;5:1380-1386. doi:10.1523/JNEUROSCI.05-05-01380.1985.
- McNair PJ, Marshall RN, Maguire K, Brown C. Knee joint effusion and proprioception. Arch Phys Med Rehabil. 1995;76:566-568. doi:10.1016/S0003-9993(95)80512-5.
- Jerosch J, Prymka M. Knee joint proprioception in normal volunteers and patients with anterior cruciate ligament tears, taking special account of the effect of a knee bandage. Arch Orthop Trauma Surg. 1996;115:162-166. doi:10.1007/BF00434546.
- Lattanzio PJ, Petrella RJ. Knee proprioception: a review of mechanisms, measurements, and implications of muscular fatigue. Orthopedics. 1998;21:463-470.
- Lephart SM, Pincivero DM, Giraido JL, Fu FH. The role of proprioception in the management and rehabilitation of athletic injuries. Am J Sports Med. 1997;25:130-137. doi:10.1177/036354659702500126.
- Lephart SM, Riemann BL, Fu FH. Introduction to the sensorimotor system. In: Lephart SM, Fu FH, editors. Proprioception and neuromuscular control in joint stability: Champaign (IL): Human Kinetics Publishers, 2000.
- Pelletier R, Higgins J, Bourbonnais D. Is neuroplasticity in the central nervous system the missing link to our understanding of chronic musculoskeletal disorders? BMC Musculoskelet Disord. 2015;16:25. doi:10.1186/s12891-015-0480-y.
- Magill R Motor learning and control: concepts and applications. Boston, MA: WCB/McGraw-hill. 8th ed, 2007.
- Winter DA Biomechanics and motor control of human movement. 4th ed: Hoboken, NJ:Wiley, 2009.
- Hoffman M, Koceja D. Hoffmann reflex profiles and strength rations in postoperative anterior cruciate ligament reconstruction patients. Int J Neurosci. 2000;104:17-27. doi:10.3109/00207450009035006.
- Duthon VB, Barea C, Abrassart S, Fasel JH, Fritschy D, Ménétrey J. Anatomy of the anterior cruciate ligament. Knee Surg Sports Traumatol Arthrosc. 2006;14:204-213. doi:10.1007/s00167-005-0679-9.
- Zimny ML. Mechanoreceptors in articular tissues. Am J Anat. 1988;182:16-32. doi:10.1002/aja.1001820103.
- Zimny ML, Schutte M, Dabezies E. Mechanoreceptors in the human anterior cruciate ligament. Anat Rec. 1986;214:204-209. doi:10.1002/ar.1092140216.
- Zimny ML, Wink CS. Neuroreceptors in the tissues of the knee joint. J Electromyogr Kinesiol. 1991;1:148-157. doi:10.1016/1050-6411(91)90031-Y.
- Ward S, Pearce AJ, Pietrosimone B, Bennell K, Clark R, Bryant AL. Neuromuscular deficits after peripheral joint injury: a neurophysiological hypothesis. Muscle Nerve. 2015;51(3):327-332. doi:10.1002/mus.24463.
- Ball T, Schreiber A, Feige B, Wagner M, Lücking CH, Kristeva-Feige R. The role of higher-order motor areas in voluntary movement as revealed by high resolution EEG and fMRI. Neuroimage. 1999;10:682-694. doi:10.1006/nimg.1999.0507.
- Nachev P, Wydell H, O’Neill K, Husain M, Kennard C. The role of the pre-supplementary motor area in the control of action. Neuroimage. 2007;36(suppl. 2):T155-TT163. doi:10.1016/j.neuroimage.2007.03.034.
- Binder JR, Desai RH. The neurobiology of semantic memory. Trends Cogn Sci. 2011;15:527-536. doi:10.1016/j.tics.2011.10.001.
- Bonner MF, Price AR. Where is the anterior temporal lobe and what does it do? J Neurosci. 2013;33:4213-4215. doi:10.1523/JNEUROSCI.0041-13.2013.
- Peuskens H, Vanrie J, Verfaillie K, Orban GA. Specificity of regions processing biological motion. Eur J Neurosci. 2005;21:2864-2875. doi:10.1111/j.1460-9568.2005.04106.x.
- Heroux ME, Tremblay F. Corticomotor excitability associated with unilateral knee dysfunction secondary to anterior cruciate ligament injury. Knee Surg Sports Traumatol Arthrosc. 2006;14:823-833. doi:10.1007/s00167-006-0063-4.
- Grooms D, Page S, Onate J. Brain activation for knee movement measured days before second anterior cruciate ligament injury: neuroimaging in musculoskeletal medicine. J Athl Train.2015;50(10):1005-1010. doi:10.4085/1062-6050-50.10.02.
- Stoodley CJ, Schmahmann JD. Functional topography in the human cerebellum: a meta-analysis of neuroimaging studies. Neuroimage. 2009;44(2):489-501. doi:10.1016/j.neuroimage.2008.08.039.
- Mansour A, Farmer M, Baliki M, Apkarian AV. Chronic pain: the role of learning and brain plasticity. Restor Neurol Neurosci. 2014;32:129-139. doi:10.3233/RNN-139003.
- Nyland J, Wera J, Klein S, Caborn DN. Lower extremity neuromuscular compensations during instrumented single leg hop testing 2-10 years following ACL reconstruction. Knee. 2014;21:1191-1197. doi:10.1016/j.knee.2014.07.017.
- Van Vliet PM, Heneghan N. Motor control and the management of musculoskeletal dysfunction. Man Ther. 2006;11(3):208-213. doi:10.1016/j.math.2006.03.009.
- Bayona NA, Bitensky J, Teasell R. Plasticity and reorganization of the uninjured brain. Top Stroke Rehabil. 2005;12:1-10. doi:10.1310/A422-G91U-Q4HB-86XC.
- Remple M, Bruneau R, VandenBerg P, Goertzen C, Kleim JA. Sensitivity of cortical movement representations to motor experience evidence that skilled learning but not strength training induces cortical reorganization. Behav Brain Res. 2001;123:133-141. doi:10.1016/S0166-4328(01)00199-1.
- Jull GA, Falla D, Vicenzino B, Hodges PW. The effect of therapeutic exercise on activation of the deep cervical flexor muscles in people with chronic neck pain. Man Ther. 2009;14:696-701. doi:10.1016/j.math.2009.05.004.
- Karni A, Meyer G, Jezzard P, Adams MM, Turner R, Ungerleider LG. Functional MRI evidence for adult motor cortex plasticity during motor skill learning. Nature. 1995;377:155-158. doi:10.1038/377155a0.
- Koeneke S, Lutz K, Herwig U, Ziemann U, Jäncke L. Extensive training of elementary finger tapping movements changes the pattern of motor cortex excitability. Exp Brain Res. 2006;174:199-209. doi:10.1007/s00221-006-0440-8.
- O’Leary S, Falla D, Elliott JM, Jull G. Muscle dysfunction in cervical spine pain: implications for assessment and management. J Orthop Sports Phys Ther. 2009;39:324-333. doi:10.2519/jospt.2009.2872.
- Pascual-Leone A, Nguyet D, Cohen LG, Brasil-Neto JP, Cammarota A, Hallett M. Modulation of muscle responses evoked by transcranial magnetic stimulation during the acquisition of new fine motor skills. J Neurophysiol. 1995;74:1037-1045. doi:10.1152/jn.1995.74.3.1037.
- Svensson P, Romaniello A, Wang K, Arendt-Nielsen L, Sessle BJ. One hour of tongue-task training is associated with plasticity in corticomotor control of the human tongue musculature. Exp Brain Res. 2006;173:165-173. doi:10.1007/s00221-006-0380-3.
- Tsao H, Druitt TR, Schollum TM, Hodges PW. Motor training of the lumbar paraspinal muscles induces immediate changes in motor coordination in patients with recurrent low back pain. J Pain. 2010;11:1120-1128. doi:10.1016/j.jpain.2010.02.004.
- Gokeler A, Banjaminse A, Hewett TE, Paterno MV, Ford KR, Otten E, Myer GD. Feedback techniques to target functional deficits following anterior cruciate ligament reconstruction: implications for motor control and reduction of second injury risk. Sports. Med. 2013;43:1065-1074. doi:10.1007/s40279-013-0095-0.
- Powers CM, Fisher B. Mechanisms underlying ACL injury-prevention training: the brain-behavior relationship. J Athl Train. 2010;45:513-515. doi:10.4085/1062-6050-45.5.513.
- Seidler RD, Noll DC. Neuroanatomical correlates of motor acquisition and motor transfer. J Neurophysiol. 2008;99:1836-1845. doi:10.1152/jn.01187.2007.
- Brophy RH, Stepan JG, Silvers HL, Mandelbaum BR. Defending puts the anterior cruciate ligament at risk during soccer: a gender-based analysis. Sports Health. 2015;7:244-249. doi:10.1177/1941738114535184.
- Sell TC, Ferris CM, Abt JP, Tsai YS, Myers JB, Fu FH, Lephart SM. Predictors of proximal tibia anterior shear force during a vertical stop-jump. J Orthop Sports Res. 2007;25:1589-1597. doi:10.1002/jor.20459.
- Yu B, Lin CF, Garrett WE. Lower extremity biomechanics during the landing of a stop-jump task. Clin Bio. 2006;21:297-305. doi:10.1016/j.clinbiomech.2005.11.003.
- Cortes N, Onate J, Van Lunen B. Pivot task increases knee frontal plane loading compared with sidestep and drop-jump. J Sports Sci. 2011;29:83-92. doi:10.1080/02640414.2010.523087.
- Blackburn JT, Padua DA. Influence of trunk flexion on hip and knee joint kinematics during a controlled drop landing. Clin Biomech. 2008;23:313-319. doi:10.1016/j.clinbiomech.2007.10.003.
- Li G, Papannagari R, DeFrate LE, Yoo JD, Park SE, Gill TJ. Comparison of the ACL and ACL graft forces before and after ACL reconstruction: an in-vitro robotic investigation. Acta Orthop. 2006;77:267-274. doi:10.1080/17453670610046019.
- Zheng N, Fleisig GS, Escamilla RF, Barrentine SW. An analytical model of the knee for estimation of internal forces during exercise. J Biomech. 1998;31:963-967. doi:10.1016/S0021-9290(98)00056-6.
- Li G, Rudy TW, Sakan M, Kanamori A, Ma CB, Woo SL. The importance of quadriceps and hamstring muscle loading on knee kinematics and in situ forces in the ACL. J Biomech. 1999;32:395-400. doi:10.1016/S0021-9290(98)00181-X.
- Hewett TE, Myer GD, Ford KR. Anterior cruciate ligament injuries in female athletes, Part l: Mechanisms and risk factors. Am J Sports Med.2006;34:299-311. doi:10.1177/0363546505284183.
- Daniel DM, Stone ML, Barnett P. Use of the quadriceps active test to diagnose to diagnose posterior cruciate ligament disruption and measure posterior laxity of the knee. J Bone Jt SurA, (Am.).1988;70:386-391.
- Hoffman M, Schrader J, Koceja D. An investigation of postural control in postoperative anterior cruciate ligament reconstruction patients. J Athl Train. 1999;34:130-136.
- Mattacola CG, Perrin DH, Gansneder BM, Gieck JH, Saliba EN, McCue FC. Strength, functional outcome, and postural stability after anterior cruciate ligament reconstruction. J Athl Train.2002;37:262-268.
- McLean SG, Lipfert SW, van den Boget AJ. Effect of gender and defensive opponent on the biomechanics of sidestep cutting. Med Sci Sports Exerc. 2004;36:1008-1016. doi:10.1249/01.MSS.0000128180.51443.83.
- McLean SG, Neal RJ, Myers PT, Walters MR. Knee joint kinematics during the sidestep cutting maneuver: potential for injury in women. Med Sci Sports Exerc. 1999;31:959-968. doi:10.1097/00005768-199907000-00007.
- O’Connell M, George K, Stock D. Postural sway and balane testing: a comparison of normal and anterior cruciate ligament deficient knees. Gait Posture. 1998;8:136-142. doi:10.1016/S0966-6362(98)00023-X.
- Okuda K, Abe N, Katayama Y, Senda M, Kuroda T, Inoue H. Effect of vision on postural sway in anterior cruciate ligament injured knees. J Orthop Sci. 2005;10:277-283. doi:10.1007/s00776-005-0893-9.
- Swanik CB, Lephart SM, Giraldo JL, Demont RG, Fu FH. Reactive muscle firing of anterior cruciate ligament-injured females during functional activities. J Athl Train. 1999;34:121-129.
- Bennett S, Ashford D, Rioja N, Elliott D. Intermittent vision and one-handed catching: the effect of general and specific task experience. J Mot Behav. 2004;36:442-449. doi:10.3200/JMBR.36.4.442-449.
- Bennett SJ, Elliott D, Weeks DJ, Keil D. The effects of intermittent vision on prehension under binocular and monocular viewing. Mot Contr. 2003;7:46-56. doi:10.1123/mcj.7.1.46.
- Grooms D, Appelbaum G, Onate J. Neuroplasticity following anterior cruciate ligament injury: a framework for visual-motor training approaches in rehabilitation. J Orthop Sports Phys Ther.2015;45(5):381-393. doi:10.2519/jospt.2015.5549.
- Ardern CL. Anterior cruciate ligament reconstruction-not exactly a one-way ticket back to the preinjury level: a review of contextual factors affecting return to sport after surgery. Sports Health.2015;7(3):224-230. doi:10.1177/1941738115578131.
- Hsu CJ, Meierbachtol A, George SZ, Chmielewski TL. Fear of reinjury in athletes. Sports Health.2017;9(2):162-167. doi:10.1177/1941738116666813.
- Medvecky MJ, Nelson S. Kinesiophobia and return to sports after anterior cruciate ligament reconstruction. Conn Med. 2015;79(3):155-157.
- Chmielewski TL, ZG, Lentz TA, Tillman SM, Moser MW, Indelicato PA, George SZ. Longitudinal changes in psychosocial factors and their association with knee pain and function after anterior cruciate ligament reconstruction. Phys Ther. 2011;91:1355-1366. doi:10.2522/ptj.20100277.
- Clement D, Arvinen-Barrow M, Fetty T. A-BM, Fetty T. Psychosocial responses during different phases of sport-injury rehabilitation: a qualitative study. J Athl Train. 2014;50:95-104. doi:10.4085/1062-6050-49.3.52.
- Te Wierike SC, van der Sluis A, van den Akker-Scheek I, Elferink-Gemser MT, Visscher C. Psychosocial factors influencing the recovery of athletes with anterior cruciate ligament injury: a systematic review. Scand J Med Sci Sports. 2013;23(5):527-540. doi:10.1111/sms.12010.
- Wiese-Bjornstal DM. Psychology and socioculture affect injury risk, response, and recovery in high-intensity athletes: a consensus statement. Scand J Med Sci Sports. 2010;20(Suppl. 2):103-111. doi:10.1111/j.1600-0838.2010.01195.x.
- Crossman J, GL, Jamieson J. The emotional responses of injured athletes. NZ J Sports Med. 1995;23:1-2.
- Hambly K. The use of the tegner activity scale for articular cartilage repair of the knee: a systematic review. Knee Surg Sports Traumatol Arthrosc. 2011;19(4):604-614. doi:10.1007/s00167-010-1301-3.
- Hambly K, Griva K. IKDC or KOOS: which one captures symptoms and disabilities most important to patients who have undergone initial anterior cruciate ligament reconstruction? Am J Sports Med. 2010;38(7):1395-1404. doi:10.1177/0363546509359678.
- Archer K, Reinke E, Huston LJ, Bird M, Scaramuzza E, Coronado R, Haug C, Vanston S, Spindler KP. Impact of preoperative expectations and fear of movement on return to sport and KOOS scores at 6 months following ACL reconstruction. Orthop J Sports Med. 2015;3(7 suppl.2):2325967115S2325900113. doi:10.1177/2325967115S00113.
- Zarzycki R, Failla M, Arundale AJH, Capin JJ, Snyder-Mackler L. Athletes with a positive psychological response to return to sport training have better outcomes one and two years after ACL reconstruction. Orthop J Sports Med. 2017;5(7 suppl.6):2325967117S2325900324. doi:10.1177/2325967117
- Shumway-Cook A, Woollacott MH Motor control: translating research into clinical practice. 4th ed: Lippincott, Williams and Wilkins, 2012.
- Ardern CL, Webster KE, Taylor NF, Feller JA. Return to the preinjury level of competitive sport after anterior cruciate ligament reconstruction surgery: two-thirds of patients have not returned by 12 months after surgery. Am J Sports Med. 2011;39(3):538-543. doi:10.1177/0363546510384798.
- Bauer M, Feeley BT, Wawrzyniak JR, Pinkowsky G, Gallo RA. Factors affecting return to play after anterior cruciate ligament reconstruction: a review of the current literature. Phys Sportsmed. 2014;42(4):71-79. doi:10.3810/psm.2014.11.2093.
- Eisenstein ED, Rawicki NL, Rensing NJ, Kusnezov NA, Lanzi JT. Variables afftecting return to play after anterior cruciate ligament injury in the national football league. Orthop J Sports Med. 2016;4(10):2325967116670117.
- Ellman MB, Sherman SL, Forsythe B, LaPrade RF, Cole BJ, Bach BR. Return to play following anterior cruciate ligament reconstruction. J Am Acad Orthop Surg. 2015;23(5):283-296. doi:10.5435/JAAOS-D-13-00183.
- Fabricant PD, Chin CS, Conte S, Conte S, Coleman SH, Pearle AD, Dines JS. Return to play after anterior cruciate ligament reconstruction in major league baseball athletes. Arthroscopy. 2015;31(5):896-900. doi:10.1016/j.arthro.2014.12.008.
- Morris RC, Hulstyn MJ, Fleming BC, Owens BD, Fadale PD. Return to play following anterior cruciate ligament reconstruction. Clin Sports Med. 2016;35:(4):655-668. doi:10.1016/j.csm.2016.05.009.
- Paterno MV, Rauh MJ, Schmitt LC, Ford KR, Hewett TE. Incidence of second ACL injuries 2 years after primary ACL reconstruction and return to sport. Am J Sports Med. 2014;42(7):1567-1573. doi:10.1177/0363546514530088.
- Sclafani MP, Davis CC. Return to play progression for rugby following injury to the lower extremity. A clinical commentary and review of the literature. Int J Sports Phys Ther. 2016;11(2):302-320.
- Walden M, Hagglund M, Magnusson H, Ekstrand J. ACL injuries in men’s professional football: a 15-year prospective study on time trends and return-to-play rates reveals only 65% of players still play at the top level 3 years after ACL rupture. Br J Sports Med. 2016;50(12):744-750. doi:10.1136/bjsports-2015-095952.
- Wiggins AJ, Grandhi RK, Schneider DK, Stanfield D, Webster KE, Myer GD. Risk of secondary injury in younger athletes after anterior cruciate ligament reconstruction: a systematic review and meta-analysis. Am J Sports Med. 2016;44(7):1861-1876. doi:10.1177/0363546515621554.
- Arundale AJ, Cummer K, Capin JJ, Zarzycki R, Snyder-Mackler L. Report of the clinical and functional primary outcomes in men of the ACL-SPORTS trial: similar outcomes in men receiving secondary prevention with and without perturbation training 1 and 2 years after ACL reconstruction. Clin Orthop Relat Res. 2017;475(10):2523-2534. doi:10.1007/s11999-017-5280-2.
- Brumitt J, HB, Manske RC, Niemuth PE, Rauh MJ. Lower extremity functional tests and risk of injury in division III collegiate athletes. Int J Sports Phys Ther. 2013;8:216-227.
- Cacolice PA, Carcia CR, Scibek JS, Phelps AL. The use of functional tests to predict sagittal plane knee kinematics in ncaa-d1 female athlets. Int J Sports Phys Ther. 2015;10(4):493-504.
- Goodstadt NM, Hunter-Giordano A, Axe MJ, Snyder-Mackler L. Functonal testing to determine readiness to discontinue brace use one year after acl reconstruction. Int J Sports Phys Ther. 2013;8(2):91-96.
- Herbst E, Hoser C, Hildebrandt C, Raschner C, Hepperger C, Pointner H, Fink C. Functional assessments for decision-making regarding return to sports following ACL reconstruction. Part ll: clinical application of a new test battery. Knee Surg Sports Traumatol Arthrosc. 2015;23(5):1283-1291. doi:10.1007/s00167-015-3546-3.
- Hoog P, Warren M, Smith CA, Chimera NJ. Functional hop tests and tuck jump assessment scores between female division l collegiate athletes participating in high versus low acl injury prone sports: a cross sectional analysis. Int J Sports Phys Ther. 2016;11(6):945-953.
- Mohammadi F, Salavati M, Akhbari B, Mazaheri M, Mohsen Mir S, Etemadi Y. Comparison of functional outcome measures after ACL reconstruction in competitive soccer players: a randomized trial. J Bone Joint Surg, (Am.). 2013;95(14):1271-1277. doi:10.2106/JBJS.L.00724.
- Rambaud A, Samozino P, Edouard P. Functional tests can they help in the decision to return to sports after anterior cruciate ligament? Example with hop tests. Ann Phys Rehabil Med. 2016;59s:e19-ee20. doi:10.1016/j.rehab.2016.07.047.
- Xergia SA, Pappas E, Zampeli F, Georgiou S, Georgoulis AD. Asymmetries in functional hop tests, lower extremity kinematics, and isokinetic strength persist 6 to 9 months following anterior cruciate ligament reconstruction. J Orthop Sports Phys Ther. 2013;43(3):154-162. doi:10.2519/jospt.2013.3967.
- Ardern CL, Taylor NF, Feller JA, Webster KE. A systematic review of the psychological factors associated with returning to sport following injury. Br J Sports Med. 2013;47(17):1120-1126. doi:10.1136/bjsports-2012-091203.
- Ardern CL, Taylor NF, Feller JA, Whitehead TS, Webster KE. Psychological responses matter in returning to preinjury level of sport after anterior cruciate ligament reconstruction surgery. Am J Sports Med. 2013;41(7):1549-1558. doi:10.1177/0363546513489284.
- Christino MA, Fantry AJ, Vopat BG. Psychological aspects of recovery following anterior cruciate ligament reconstruction. J Am Acad Orthop Surg. 2015;23(8):501-509. doi:10.5435/JAAOS-D-14-00173.
- Naghdi S, Nakhostin Ansari N, Farhadi Y, Ebadi S, Entezary E, Glazer D. Cross-cultural adaptation and validation of the Injury-Psychological Readiness to Return to Sport scale to Persian language. Physiother Theory Pract. 2016;32(7):528-535. doi:10.1080/09593985.2016.1221486.
- Hui C, Salmon LJ, Kok A, Maeno S, Linklater J, Pinczewski LA. Fifteen-year outcome of endoscopic anterior cruciate ligament reconstruction with patellar tendon autograft for “isolated” anterior cruciate ligament tear. Am J Sports Med. 2011;39:89-98. doi:10.1177/0363546510379975.
- Paterno MV, Rauh MJ, Schmitt LC, Ford KR, Hewett TE. Incidence of contralateral and ipsilateral anterior cruciate ligament (ACL) injury after primary ACL reconstruction and return to sport. Clin J Sport Med. 2012;22:116-121. doi:10.1097/JSM.0b013e318246ef9e.
- Paterno MV, Schmitt LC, Ford KR, Rauh MJ, Myer GD, Huang B, Hewett TE. Biomechanical measures during landing and postural stability predict second anterior cruciate ligament injury after anterior cruciate ligament reconstruction and return to sport. Am J Sports Med. 2010;38:1968-1978. doi:10.1177/0363546510376053.
- Wright RW, Dunn WR, Amendola A, Andrish JT, Bergfeld J, Kaeding CC, Marx RG, McCarty EC, Parker RD, Wolcott M, Wolf BR, Spindler KP. Risk of tearing the intact anterior cruciate ligament in the contralateral knee and rupturing the anterior cruciate ligament graft during the first 2 years after anterior cruciate ligament reconstruction: a prospective MOON cohort study. Am J Sports Med. 2007;35:1131-1134. doi:10.1177/0363546507301318.
- Bystrom MG, Rasmussen-Barr E, Grooten WJ. Motor control exercises reduces pain and disability in chronic and recurrent low back pain: a meta-analysis. Spine. 2013;38(6):E350-E358. doi:10.1097/BRS.0b013e31828435fb.
- Macedo LG, Maher CG, Latimer J, McAuley JH. Motor control exercise for persistent nonspecific low back pain: a systematic review. Phys Ther. 2009;89(1):9-25. doi:10.2522/ptj.20080103.
- Grindstaff TL, Hammill RR, Tuzson AE, Hertel J. Neuromuscular control training programs and noncontact anterior cruciate ligament injur rates in female athletes: a numbers-needed-to-treat analysis. J Athl Train. 2006;41:450-456.
- Myer GD, Ford KR, Brent JL, Hewett TE. An integrated approach to change the outcome part II: Targeted neuromuscular training techniques to reduce identified ACL injury risk factors. J Strength Cond Res. 2012;26:2272-2292. doi:10.1519/JSC.0b013e31825c2c7d.
- Myer GD, Paterno MV, Ford KR, Hewett TE. Neuromuscular training techniques to target deficits before return to sport after anterior cruciate ligament reconstruction. J Strength Cond Res. 2008;22:987-1014. doi:10.1519/JSC.0b013e31816a86cd.
- Yoo JH, Lim BO, Ha M, Lee SW, Oh SJ, Lee YS, Kim JG. A meta-analysis of the effect of neuromuscular training on the prevention of the anterior cruciate ligament injury in female athletes. Knee Surg Sports Traumatol Arthrosc. 2010;18:824-830. doi:10.1007/s00167-009-0901-2.
- Moseley GL, Flor H. Targeting cortical representations in the treatment of chronic pain; a review. Neurorehabil Neural Repair. 2012;26(6):646-652. doi:10.1177/1545968311433209.
- Cramer SC. Brain repair after stroke. New Engl J Med 2010;362(19):1827-1829. doi:10.1056/NEJMe1003399.
- Baumeister J, Reinecke K, Weiss M. Changed cortical activity after anterior cruciate ligament reconstruction in a joint position paradigm: an EEG study. Scand J Med Sci Sports. 2008;18:473-484. doi:10.1111/j.1600-0838.2007.00702.x.
- Baumeister J, Reinecke K, Schubert M, Weiss M. Altered electrocortical brain activity after ACL reconstruction during force control. J Orthop Res. 2011;29:1383-1389. doi:10.1002/jor.21380.
- Courtney C, Rine RM. Central somatosensory changes associated with improved dynamic balance in subjects with anterior cruciate ligament deficiency. Gait Posture. 2006;24:190-195. doi:10.1016/j.gaitpost.2005.08.006.
- Courtney C, Rine RM, Kroll P. Central somatosensory changes and altered muscle synergies in subjects with anterior cruciate ligament deficiency. Gait Posture. 2005;22:69-74. doi:10.1016/j.gaitpost.2004.07.002.
- Kapreli E, Athanasopoulos S, Gliatis J, Papathanasiou M, Peeters R, Strimpakos N, Van Hecke P, Gouliamos A, Sunaert S. Anterior cruciate ligament deficiency causes brain plasticity: a functional MRI study. Am J Sports Med. 2009;37:2419-2426. doi:10.1177/0363546509343201.
- Valeriani M, Restuccia D, Di Lazaro V, Franceschi F, Fabbriciani C, Tonali P. Clinical and neurophysiological abnormalities before and after reconstruction of the anterior cruciate ligament of the knee. Acta Neurol Scand. 1999;99:303-307. doi:10.1111/j.1600-0404.1999.tb00680.x.
- Burland JP, Toonstra J, Werner JL, Mattacola CG, Howell DM, Howard JS. Decision to return to sport after anterior cruciate ligament reconstruction, Part 1: A qualitative investigation of psychosocial factors. J Athl Train. 2018;53(5):452-463. doi:10.4085/1062-6050-313-16
- Johansson H, Sjolander P, Sojka P. A sensory role for the cruciate ligaments. Clin Orthop Relat Res. 1991;268:161-178.
- Kaprelli E, Athanasopoulos S. The anterior cruciate ligament deficiency as a model of brain plasticity. Med Hypo. 2006;67:645-650. doi:10.1016/j.mehy.2006.01.063.
- Valeriani M, Restuccia D, Di Lazzaro V, Franceschi F, Fabbriciani C, Tonali P. Central nervous system modifications in patients with lesion of the anterior cruciate ligament of the knee. Brain. 1996;119(Pt 5):1751-1762. doi:10.1093/brain/119.5.1751.
- Nyland J, Fisher B, Brad E, Krupp R, Caborn DN. Osseous deficits after anterior cruciate ligament injury and reconstruction: a systematic review with suggestions to improve osseous homeostasis. Arthroscopy. 2010;26:1248-1257. doi:10.1016/j.arthro.2010.03.017.
- Nyland J, Klein S, Caborn DN. Lower extremity compensatory neuromuscular and biomechanical adaptations 2 to 11 years after anterior cruciate ligament reconstruction. Arthroscopy. 2010;26:1212-1225. doi:10.1016/j.arthro.2010.01.003.
- Paterno MV, Ford KR, Myer GD, Heyl R, Hewett TE. Limb asymmetries in landing and jumping 2 years following anterior cruciate ligament reconstruction. Clin. J Sports Med. 2007;17:258-262. doi:10.1097/JSM.0b013e31804c77ea.
- Wojtys EM, Huston LJ. Longitudinal effects of anterior cruciate ligament injury and patellar tendon autograft reconstruction on neuromuscular performance. Am J Sports Med. 2000;28:336-344. doi:10.1177/03635465000280030901.
- Levine JD, Dardick SJ, Basbaum AI, Scipio E. Reflex neurogenic inflammation. I. Contribution of the peripheral nervous system to spatially remote inflammatory responses that follow injury. J Neurosci. 1985;5:1380-1386. doi:10.1523/JNEUROSCI.05-05-01380.1985.
- McNair PJ, Marshall RN, Maguire K, Brown C. Knee joint effusion and proprioception. Arch Phys Med Rehabil. 1995;76:566-568. doi:10.1016/S0003-9993(95)80512-5.
- Jerosch J, Prymka M. Knee joint proprioception in normal volunteers and patients with anterior cruciate ligament tears, taking special account of the effect of a knee bandage. Arch Orthop Trauma Surg. 1996;115:162-166. doi:10.1007/BF00434546.
- Lattanzio PJ, Petrella RJ. Knee proprioception: a review of mechanisms, measurements, and implications of muscular fatigue. Orthopedics. 1998;21:463-470.
- Lephart SM, Pincivero DM, Giraido JL, Fu FH. The role of proprioception in the management and rehabilitation of athletic injuries. Am J Sports Med. 1997;25:130-137. doi:10.1177/036354659702500126.
- Lephart SM, Riemann BL, Fu FH. Introduction to the sensorimotor system. In: Lephart SM, Fu FH, editors. Proprioception and neuromuscular control in joint stability: Champaign (IL): Human Kinetics Publishers, 2000.
- Pelletier R, Higgins J, Bourbonnais D. Is neuroplasticity in the central nervous system the missing link to our understanding of chronic musculoskeletal disorders? BMC Musculoskelet Disord. 2015;16:25. doi:10.1186/s12891-015-0480-y.
- Magill R Motor learning and control: concepts and applications. Boston, MA: WCB/McGraw-hill. 8th ed, 2007.
- Winter DA Biomechanics and motor control of human movement. 4th ed: Hoboken, NJ:Wiley, 2009.
- Hoffman M, Koceja D. Hoffmann reflex profiles and strength rations in postoperative anterior cruciate ligament reconstruction patients. Int J Neurosci. 2000;104:17-27. doi:10.3109/00207450009035006.
- Duthon VB, Barea C, Abrassart S, Fasel JH, Fritschy D, Ménétrey J. Anatomy of the anterior cruciate ligament. Knee Surg Sports Traumatol Arthrosc. 2006;14:204-213. doi:10.1007/s00167-005-0679-9.
- Zimny ML. Mechanoreceptors in articular tissues. Am J Anat. 1988;182:16-32. doi:10.1002/aja.1001820103.
- Zimny ML, Schutte M, Dabezies E. Mechanoreceptors in the human anterior cruciate ligament. Anat Rec. 1986;214:204-209. doi:10.1002/ar.1092140216.
- Zimny ML, Wink CS. Neuroreceptors in the tissues of the knee joint. J Electromyogr Kinesiol. 1991;1:148-157. doi:10.1016/1050-6411(91)90031-Y.
- Ward S, Pearce AJ, Pietrosimone B, Bennell K, Clark R, Bryant AL. Neuromuscular deficits after peripheral joint injury: a neurophysiological hypothesis. Muscle Nerve. 2015;51(3):327-332. doi:10.1002/mus.24463.
- Ball T, Schreiber A, Feige B, Wagner M, Lücking CH, Kristeva-Feige R. The role of higher-order motor areas in voluntary movement as revealed by high resolution EEG and fMRI. Neuroimage. 1999;10:682-694. doi:10.1006/nimg.1999.0507.
- Nachev P, Wydell H, O’Neill K, Husain M, Kennard C. The role of the pre-supplementary motor area in the control of action. Neuroimage. 2007;36(suppl. 2):T155-TT163. doi:10.1016/j.neuroimage.2007.03.034.
- Binder JR, Desai RH. The neurobiology of semantic memory. Trends Cogn Sci. 2011;15:527-536. doi:10.1016/j.tics.2011.10.001.
- Bonner MF, Price AR. Where is the anterior temporal lobe and what does it do? J Neurosci. 2013;33:4213-4215. doi:10.1523/JNEUROSCI.0041-13.2013.
- Peuskens H, Vanrie J, Verfaillie K, Orban GA. Specificity of regions processing biological motion. Eur J Neurosci. 2005;21:2864-2875. doi:10.1111/j.1460-9568.2005.04106.x.
- Heroux ME, Tremblay F. Corticomotor excitability associated with unilateral knee dysfunction secondary to anterior cruciate ligament injury. Knee Surg Sports Traumatol Arthrosc. 2006;14:823-833. doi:10.1007/s00167-006-0063-4.
- Grooms D, Page S, Onate J. Brain activation for knee movement measured days before second anterior cruciate ligament injury: neuroimaging in musculoskeletal medicine. J Athl Train.2015;50(10):1005-1010. doi:10.4085/1062-6050-50.10.02.
- Stoodley CJ, Schmahmann JD. Functional topography in the human cerebellum: a meta-analysis of neuroimaging studies. Neuroimage. 2009;44(2):489-501. doi:10.1016/j.neuroimage.2008.08.039.
- Mansour A, Farmer M, Baliki M, Apkarian AV. Chronic pain: the role of learning and brain plasticity. Restor Neurol Neurosci. 2014;32:129-139. doi:10.3233/RNN-139003.
- Nyland J, Wera J, Klein S, Caborn DN. Lower extremity neuromuscular compensations during instrumented single leg hop testing 2-10 years following ACL reconstruction. Knee. 2014;21:1191-1197. doi:10.1016/j.knee.2014.07.017.
- Van Vliet PM, Heneghan N. Motor control and the management of musculoskeletal dysfunction. Man Ther. 2006;11(3):208-213. doi:10.1016/j.math.2006.03.009.
- Bayona NA, Bitensky J, Teasell R. Plasticity and reorganization of the uninjured brain. Top Stroke Rehabil. 2005;12:1-10. doi:10.1310/A422-G91U-Q4HB-86XC.
- Remple M, Bruneau R, VandenBerg P, Goertzen C, Kleim JA. Sensitivity of cortical movement representations to motor experience evidence that skilled learning but not strength training induces cortical reorganization. Behav Brain Res. 2001;123:133-141. doi:10.1016/S0166-4328(01)00199-1.
- Jull GA, Falla D, Vicenzino B, Hodges PW. The effect of therapeutic exercise on activation of the deep cervical flexor muscles in people with chronic neck pain. Man Ther. 2009;14:696-701. doi:10.1016/j.math.2009.05.004.
- Karni A, Meyer G, Jezzard P, Adams MM, Turner R, Ungerleider LG. Functional MRI evidence for adult motor cortex plasticity during motor skill learning. Nature. 1995;377:155-158. doi:10.1038/377155a0.
- Koeneke S, Lutz K, Herwig U, Ziemann U, Jäncke L. Extensive training of elementary finger tapping movements changes the pattern of motor cortex excitability. Exp Brain Res. 2006;174:199-209. doi:10.1007/s00221-006-0440-8.
- O’Leary S, Falla D, Elliott JM, Jull G. Muscle dysfunction in cervical spine pain: implications for assessment and management. J Orthop Sports Phys Ther. 2009;39:324-333. doi:10.2519/jospt.2009.2872.
- Pascual-Leone A, Nguyet D, Cohen LG, Brasil-Neto JP, Cammarota A, Hallett M. Modulation of muscle responses evoked by transcranial magnetic stimulation during the acquisition of new fine motor skills. J Neurophysiol. 1995;74:1037-1045. doi:10.1152/jn.1995.74.3.1037.
- Svensson P, Romaniello A, Wang K, Arendt-Nielsen L, Sessle BJ. One hour of tongue-task training is associated with plasticity in corticomotor control of the human tongue musculature. Exp Brain Res. 2006;173:165-173. doi:10.1007/s00221-006-0380-3.
- Tsao H, Druitt TR, Schollum TM, Hodges PW. Motor training of the lumbar paraspinal muscles induces immediate changes in motor coordination in patients with recurrent low back pain. J Pain. 2010;11:1120-1128. doi:10.1016/j.jpain.2010.02.004.
- Gokeler A, Banjaminse A, Hewett TE, Paterno MV, Ford KR, Otten E, Myer GD. Feedback techniques to target functional deficits following anterior cruciate ligament reconstruction: implications for motor control and reduction of second injury risk. Sports. Med. 2013;43:1065-1074. doi:10.1007/s40279-013-0095-0.
- Powers CM, Fisher B. Mechanisms underlying ACL injury-prevention training: the brain-behavior relationship. J Athl Train. 2010;45:513-515. doi:10.4085/1062-6050-45.5.513.
- Seidler RD, Noll DC. Neuroanatomical correlates of motor acquisition and motor transfer. J Neurophysiol. 2008;99:1836-1845. doi:10.1152/jn.01187.2007.
- Brophy RH, Stepan JG, Silvers HL, Mandelbaum BR. Defending puts the anterior cruciate ligament at risk during soccer: a gender-based analysis. Sports Health. 2015;7:244-249. doi:10.1177/1941738114535184.
- Sell TC, Ferris CM, Abt JP, Tsai YS, Myers JB, Fu FH, Lephart SM. Predictors of proximal tibia anterior shear force during a vertical stop-jump. J Orthop Sports Res. 2007;25:1589-1597. doi:10.1002/jor.20459.
- Yu B, Lin CF, Garrett WE. Lower extremity biomechanics during the landing of a stop-jump task. Clin Bio. 2006;21:297-305. doi:10.1016/j.clinbiomech.2005.11.003.
- Cortes N, Onate J, Van Lunen B. Pivot task increases knee frontal plane loading compared with sidestep and drop-jump. J Sports Sci. 2011;29:83-92. doi:10.1080/02640414.2010.523087.
- Blackburn JT, Padua DA. Influence of trunk flexion on hip and knee joint kinematics during a controlled drop landing. Clin Biomech. 2008;23:313-319. doi:10.1016/j.clinbiomech.2007.10.003.
- Li G, Papannagari R, DeFrate LE, Yoo JD, Park SE, Gill TJ. Comparison of the ACL and ACL graft forces before and after ACL reconstruction: an in-vitro robotic investigation. Acta Orthop. 2006;77:267-274. doi:10.1080/17453670610046019.
- Zheng N, Fleisig GS, Escamilla RF, Barrentine SW. An analytical model of the knee for estimation of internal forces during exercise. J Biomech. 1998;31:963-967. doi:10.1016/S0021-9290(98)00056-6.
- Li G, Rudy TW, Sakan M, Kanamori A, Ma CB, Woo SL. The importance of quadriceps and hamstring muscle loading on knee kinematics and in situ forces in the ACL. J Biomech. 1999;32:395-400. doi:10.1016/S0021-9290(98)00181-X.
- Hewett TE, Myer GD, Ford KR. Anterior cruciate ligament injuries in female athletes, Part l: Mechanisms and risk factors. Am J Sports Med.2006;34:299-311. doi:10.1177/0363546505284183.
- Daniel DM, Stone ML, Barnett P. Use of the quadriceps active test to diagnose to diagnose posterior cruciate ligament disruption and measure posterior laxity of the knee. J Bone Jt SurA, (Am.).1988;70:386-391.
- Hoffman M, Schrader J, Koceja D. An investigation of postural control in postoperative anterior cruciate ligament reconstruction patients. J Athl Train. 1999;34:130-136.
- Mattacola CG, Perrin DH, Gansneder BM, Gieck JH, Saliba EN, McCue FC. Strength, functional outcome, and postural stability after anterior cruciate ligament reconstruction. J Athl Train.2002;37:262-268.
- McLean SG, Lipfert SW, van den Boget AJ. Effect of gender and defensive opponent on the biomechanics of sidestep cutting. Med Sci Sports Exerc. 2004;36:1008-1016. doi:10.1249/01.MSS.0000128180.51443.83.
- McLean SG, Neal RJ, Myers PT, Walters MR. Knee joint kinematics during the sidestep cutting maneuver: potential for injury in women. Med Sci Sports Exerc. 1999;31:959-968. doi:10.1097/00005768-199907000-00007.
- O’Connell M, George K, Stock D. Postural sway and balane testing: a comparison of normal and anterior cruciate ligament deficient knees. Gait Posture. 1998;8:136-142. doi:10.1016/S0966-6362(98)00023-X.
- Okuda K, Abe N, Katayama Y, Senda M, Kuroda T, Inoue H. Effect of vision on postural sway in anterior cruciate ligament injured knees. J Orthop Sci. 2005;10:277-283. doi:10.1007/s00776-005-0893-9.
- Swanik CB, Lephart SM, Giraldo JL, Demont RG, Fu FH. Reactive muscle firing of anterior cruciate ligament-injured females during functional activities. J Athl Train. 1999;34:121-129.
- Bennett S, Ashford D, Rioja N, Elliott D. Intermittent vision and one-handed catching: the effect of general and specific task experience. J Mot Behav. 2004;36:442-449. doi:10.3200/JMBR.36.4.442-449.
- Bennett SJ, Elliott D, Weeks DJ, Keil D. The effects of intermittent vision on prehension under binocular and monocular viewing. Mot Contr. 2003;7:46-56. doi:10.1123/mcj.7.1.46.
- Grooms D, Appelbaum G, Onate J. Neuroplasticity following anterior cruciate ligament injury: a framework for visual-motor training approaches in rehabilitation. J Orthop Sports Phys Ther.2015;45(5):381-393. doi:10.2519/jospt.2015.5549.
- Ardern CL. Anterior cruciate ligament reconstruction-not exactly a one-way ticket back to the preinjury level: a review of contextual factors affecting return to sport after surgery. Sports Health.2015;7(3):224-230. doi:10.1177/1941738115578131.
- Hsu CJ, Meierbachtol A, George SZ, Chmielewski TL. Fear of reinjury in athletes. Sports Health.2017;9(2):162-167. doi:10.1177/1941738116666813.
- Medvecky MJ, Nelson S. Kinesiophobia and return to sports after anterior cruciate ligament reconstruction. Conn Med. 2015;79(3):155-157.
- Chmielewski TL, ZG, Lentz TA, Tillman SM, Moser MW, Indelicato PA, George SZ. Longitudinal changes in psychosocial factors and their association with knee pain and function after anterior cruciate ligament reconstruction. Phys Ther. 2011;91:1355-1366. doi:10.2522/ptj.20100277.
- Clement D, Arvinen-Barrow M, Fetty T. A-BM, Fetty T. Psychosocial responses during different phases of sport-injury rehabilitation: a qualitative study. J Athl Train. 2014;50:95-104. doi:10.4085/1062-6050-49.3.52.
- Te Wierike SC, van der Sluis A, van den Akker-Scheek I, Elferink-Gemser MT, Visscher C. Psychosocial factors influencing the recovery of athletes with anterior cruciate ligament injury: a systematic review. Scand J Med Sci Sports. 2013;23(5):527-540. doi:10.1111/sms.12010.
- Wiese-Bjornstal DM. Psychology and socioculture affect injury risk, response, and recovery in high-intensity athletes: a consensus statement. Scand J Med Sci Sports. 2010;20(Suppl. 2):103-111. doi:10.1111/j.1600-0838.2010.01195.x.
- Crossman J, GL, Jamieson J. The emotional responses of injured athletes. NZ J Sports Med. 1995;23:1-2.
- Hambly K. The use of the tegner activity scale for articular cartilage repair of the knee: a systematic review. Knee Surg Sports Traumatol Arthrosc. 2011;19(4):604-614. doi:10.1007/s00167-010-1301-3.
- Hambly K, Griva K. IKDC or KOOS: which one captures symptoms and disabilities most important to patients who have undergone initial anterior cruciate ligament reconstruction? Am J Sports Med. 2010;38(7):1395-1404. doi:10.1177/0363546509359678.
- Archer K, Reinke E, Huston LJ, Bird M, Scaramuzza E, Coronado R, Haug C, Vanston S, Spindler KP. Impact of preoperative expectations and fear of movement on return to sport and KOOS scores at 6 months following ACL reconstruction. Orthop J Sports Med. 2015;3(7 suppl.2):2325967115S2325900113. doi:10.1177/2325967115S00113.
- Zarzycki R, Failla M, Arundale AJH, Capin JJ, Snyder-Mackler L. Athletes with a positive psychological response to return to sport training have better outcomes one and two years after ACL reconstruction. Orthop J Sports Med. 2017;5(7 suppl.6):2325967117S2325900324. doi:10.1177/2325967117
TAKE-HOME POINTS
- The CNS demonstrates neurophysiological changes during an ACL injury.
- Traditional orthopedic treatment based on principals of musculoskeletal rehabilitation may not be sufficient to address CNS deficits.
- The CNS is neuroplastic and able to change with neuromotor rehabilitation that focuses on the CNS.
- Psychosocial factors may contribute to impairments after an ACL injury, and adversely affect functional outcomes.
- Assessment of RTP criteria should consider psychosocial, and central neural factors to minimize risk, and optimize outcomes.
A Retrospective Analysis of the Modified Intervastus Approach
ABSTRACT
The subvastus (SV) approach is a well-known muscle- and tendon-sparing approach for total knee arthroplasty (TKA), which has been shown in some studies to provide better outcomes in the visual analog pain score (VAS), knee range of motion (ROM), straight-leg raise, as well as faster rehabilitation, compared with the standard medial parapatellar (MP) approach. We previously described a new knee replacement technique known as the modified intervastus (MIV) approach. The MIV approach is a muscle- and tendon-sparing approach that is extensile and simple to perform. It may be used in the majority of complex primary cases and revisions. Here we describe the surgical technique for performing the MIV approach and provide functional outcome measures. A total of 127 patients (mean age, 66.75 years) underwent TKA using the MIV approach with 1-year follow-up. Clinical outcomes were assessed by recording both a VAS and knee ROM preoperatively, and again at several postoperative time points when the length of time required to ambulate independently (without assistive devices) was also measured. The VAS decreased significantly from the preoperative period (3.69 ± 2.22) to postoperative day 1 (3.17 ± 1.97) (P < .05). Although knee ROM decreased 1 week after surgery, the ROM increased by 6 weeks after surgery compared with the preoperative ROM, and the trend continued over the 1-year follow-up. One-third (33%) of patients were able to walk independently (without assistive devices) at 2 weeks and 78% at 8 weeks. The MIV approach to the knee is a muscle- and tendon-sparing approach that offers advantages over the SV approach and may be used for complex primary and revision total knee cases.
Continue to: Total knee arthroplasty...
Total knee arthroplasty (TKA) is one of the most common orthopedic surgical procedures, with more than 600,000 TKAs performed annually in the United States, and by 2030 the number is expected to reach 3.48 million per year.1Several approaches have been described for total TKA, and the medial parapatellar (MP) approach to the knee is considered the workhorse of total knee replacements. It is an extensile approach that is easy to perform but may delay active knee extension and straight-leg raise after surgery.2 Alternative approaches such as the subvastus (SV) and midvastus approaches to the knee (Figure 1A) typically allow a more rapid straight-leg raise but may be more challenging to perform and time-consuming in morbidly obese patients, muscular patients, and patients with severe deformities.3
The intervastus approach (Figure 1A) described by Jojima4 and others utilizes the interval between the quadriceps tendon and vastus medialis. Although it is a simple approach to perform that is extensile, it is not considered tendon-sparing since the vastus medialis inserts onto the medial aspect of the quadriceps tendon (Figure 2).5 Therefore, dissecting through this interval, without elevation of the vastus medialis (Figure 3A), damages the quadriceps tendon, and strangulation of the muscle occurs with repair of the arthrotomy site (Figure 3B). This situation is even more likely in patients with a low-lying vastus medialis.6
The modified intervastus (MIV) approach described previously,7 may be used in the majority of patients undergoing TKA. The advantages of this approach include its extensile nature, similarities to an MP approach, and preservation of both the extensor mechanism and the vastus medialis, leading to a more rapid return to active knee extension than is traditionally observed.2,7 The approach is simple to perform, easy to close, and is compatible with more extensile approaches such as a quadriceps snip if required in revision scenarios.7 However, functional outcomes of the MIV approach have not been quantified. It is unknown whether these outcomes will offer any advantages compared with the SV approach. Therefore, the objective of this study was to measure functional outcomes of the MIV approach and to compare the results with those previously published for the SV approach. We hypothesized that using the MIV approach for TKA surgery would lead to early straight-leg raise and increase in knee range of motion (ROM) postoperatively.
SURGICAL TECHNIQUE
The MIV approach preserves the quadriceps tendon and vastus medialis.7 After exposure of the vastus medialis muscle, the interval between the quadriceps tendon and vastus medialis is identified (Figure 4). The fascia overlying the lateral edge of the vastus medialis is incised where it meets the quadriceps tendon (Figure 5). The muscle is then bluntly elevated off the underlying capsule just enough to allow for a capsular repair later (Figure 6). An arthrotomy is then performed from cephalad to caudal (Figure 7). This interval may be extended proximally between the vastus intermedius and vastus medialis to expose the distal femur if needed. The closure is performed by repairing the capsule with absorbable suture (Figure 8A), and the vastus medialis fascia is repaired back to the medial edge of the quadriceps tendon, restoring the anatomy (Figure 8B).
Continue to: PATIENTS AND METHODS...
PATIENTS AND METHODS
A retrospective review of functional outcomes after TKA using the MIV approach was conducted; the study was approved by the University of Illinois Institutional Review Board. A total of 127 patients of mean age 66.75 years (range, 48–86 years) with primary osteoarthritis of the knee who were indicated for a total knee replacement with 1-year follow-up were included. The patient demographics are shown in Table 1. All patients underwent TKA using the MIV approach described above by 2 experienced orthopedic surgeons at the same institution. Patients received spinal anesthesia and a periarticular pain block intraoperatively. A measured resection technique was used by 1 surgeon, and a gap-balancing technique by the other. Surgeon 1 used the Persona PS cemented knee system (Zimmer Biomet, and Surgeon 2 used the Sigma PS cemented knee system (Depuy). Patellar resurfacing was done in all cases. Patellar tracking was checked intraoperatively using the ‘no-touch’ technique, and the need for a lateral release was noted. Drains were removed on postoperative day 1. Oral opioids were given as needed. Intravenous antibiotics were continued for 24 hours. Oral anticoagulants were used for thromboembolism prophylaxis for 3 weeks. Patients were mobilized on the day of surgery with full weight-bearing under the supervision of an experienced physical therapist. Static and dynamic quadriceps exercises were started on the same day of surgery along with active knee ROM exercises. Pain score, extensor lag, ROM, walking ability, and complications were recorded in all patients.
Table 1. Patient Demographics
Total no. of patients | 127 | |
Gender | Male | 44 |
Female | 83 | |
Age (years) | Mean ± Standard deviation | 66.75 ± 9.12 |
Range | 48 – 86 | |
Weight (lb) | Mean ± Standard deviation | 218.38 ± 54.47 |
Range | 125 – 364 | |
BMI (kg/m2) | Mean ± Standard deviation | 34.10 ± 7.22 |
Range | 21.1 – 62.5 |
The visual analog score (VAS) was obtained preoperatively and recorded on postoperative day 1. Patient walking distance with assistance was measured on the day of surgery, after surgery, and on the day of hospital discharge. Patients were assessed preoperatively and postoperatively at 1 week, 2 weeks, 6 weeks, 3 months, 6 months, and 1 year for knee ROM. A one-way ANOVA was conducted to compare the preoperative and postoperative day 1 VAS with significance set at P < .05 (OriginPro 2015, OriginLab Corporation). Differences in knee ROM between preoperative and postoperative follow-up periods (1 week, 2 weeks, 6 weeks, 3 months, 6 months, and 1 year) were identified using a 1-way ANOVA with a post hoc Tukey test. Significance was set at P < .05.
RESULTS
All patients were able to fully straight-leg raise and demonstrate functional knee ROM by postoperative day 1. The patella tracked centrally in all patients, and none required a lateral retinacular release. The majority of patients were discharged in the first 48 hours after surgery on oral narcotics. None required IV narcotics during their hospital stay or a blood transfusion. Two cases were complicated by severe knee skin blistering postoperatively due to a reaction to an adhesive dressing; one was complicated by skin necrosis leading to a flap reconstruction that became infected, requiring a 2-stage revision. A separate case had an acute postoperative infection that required irrigation and debridement with polyethylene exchange. After a 12-week course of antibiotics, the infection was eradicated. All patients reported a high satisfaction rate during their acute postoperative phase.
Postoperatively, all patients were able to walk on the day of surgery either independently or with some assistance. On the day of surgery, 10% of patients were able to walk >200 feet, and this increased to 65% of patients able to walk >200 feet on the day of discharge (compare Figure 9A and Figure 9B). Within 2 weeks of surgery, 30% of patients could walk independently (without assistive devices), and this number increased to 78% by 8 weeks after surgery (Figure 10).
Pain assessed using the VAS was lower on postoperative day 1 (3.17 ± 1.97) than the preoperative score (3.69 ± 2.22, P< .05). Overall, knee ROM significantly increased during the follow-up after surgery. Initially, the ROM decreased 1 week after surgery (90.82 ± 10.28) compared with preoperative ROM (101.04 ± 19.48, P < .001) (Figure 10). At 2 weeks after surgery, knee ROM returned to the preoperative value (100.70 ± 13.36). By 6 weeks after surgery, knee ROM was 17° greater than the preoperative ROM (118.45 ± 11.89, P < .001). Knee ROM remained stable at 3- and 6-month assessments, and showed further improvement by 1 year (126.62 ± 9.81, P < .001) compared with the preoperative state (Figure 10). The net improvement in knee ROM was 25° of increased knee flexion by 1 year.
Continue to: DISCUSSION...
DISCUSSION
TKA is a successful procedure that restores knee function with pain relief in osteoarthritis patients. The SV approach for TKA has better outcomes in terms of the VAS, ROM, straight-leg raise with faster rehabilitation compared with the standard MP approach;8–12 however, it can be challenging and time-consuming when used in morbidly obese and muscular patients.3 The SV approach can also increase the risk of complications such as patellar tendon avulsion or medial collateral injury because of the difficulty in exposure specifically for knees with limited ROM.13 Here we introduce the MIV approach as an alternative to the SV approach, overcoming most of these difficulties.
With the prevalence of morbid obesity and the market demand for minimally invasive techniques, we believe the MIV approach represents a good approach for surgeons since it is easy to perform, does not require specialized instrumentation, and is a reproducible approach even on the most complex deformities. The minimal time added to ensure blunt elevation of the vastus medialis muscle and an anatomic repair of the underlying knee capsule and vastus medialis fascia to the medial edge of the quadriceps tendon allows restoration of the anatomy and a robust double-layered watertight seal closure with no strangulation of soft tissues. We believe this reproducible muscle- and tendon-sparing approach that allows gentle, soft tissue handling even in the most complex primary total knee cases may lead to less soft tissue swelling, and therefore, less postoperative pain resulting in an accelerated recovery.
The pain level in this group of patients was reduced after the MIV approach as indicated by the VAS. The VAS was significantly decreased on postoperative day 1 compared with the VAS recorded preoperatively (P < .05), indicating patients felt less pain on the day after surgery. The average VAS on postoperative day 1 from other studies for SV approach ranged from 2.1 to 5,9,12,14–19 whereas our MIV approach value was 3.17. Periarticular blocks were available for this study group, and no peripheral nerve blocks were used. Some studies of the SV approach mention the use of peripheral nerve blocks, while others did not describe the method used for treatment or control of postoperative pain. The decreased reported pain levels and the observed increased knee ROM seen in the MIV and SV approach study groups might be attributable to the treatment of postoperative pain.
Patient ambulation also increased from the day of surgery to the day of discharge for the MIV approach. Only 10% of patients were able to walk with assistance >200 feet on the day of surgery; however, this percentage increased to 65% on the discharge day showing an excellent recovery of walking ability within 2 days of surgery. Mehta and colleagues20reported that 95% of patients undergoing subvastus/midvastus approaches could walk >10 blocks at 6 months follow-up. For the MIV approach, 78% of patients were able to walk independently within 8 weeks of surgery.
Continue to: The MIV approach shows improvement...
The MIV approach shows improvement in functional outcomes in terms of knee ROM from preoperative to postoperative. Although knee ROM decreased significantly at postoperative week 1, which is most likely because of a peak in postoperative pain and swelling; the ROM started to increase significantly at postoperative week 6. After 1 year, the knee ROM increased by 25% compared with preoperative ROM for the MIV approach. We found the average knee ROM at postoperative 3 months using the MIV approach to be 120.4°. Knee ROM at postoperative 3 months using the SV approach reported by other studies ranged from 87.1° to 120°.19,21–23 Similarly, after 1 year, the average ROM by MIV approach was 126.62°, whereas average knee ROM from other studies using the SV approach was 114.1°.2,10,19,21 Achieving a knee ROM of 120° allows patients to return to their baseline function and perform activities of daily living without limitation. With the prevalence of knee osteoarthritis in the younger population, the need for increased knee ROM post-TKA becomes more important so patients can return to work and function at their baseline.
In addition to being both a muscle- and tendon-sparing approach, other advantages of the MIV approach are that it is simple to perform and gives similar exposure to the MP approach even in difficult primary cases. It is also as extensile as the medial parapatellar approach, and it can be converted to a quadriceps snip if required. There is also minimal, if any, muscle retraction preventing muscle injury that may be seen with some other muscle-sparing approaches to the knee, especially in more difficult primary total knee cases. Another advantage to this approach, as opposed to the other approaches to the knee described here, is that it allows a double-layered closure, decreasing the possibility of arthrotomy dehiscence. Because of this double-layered closure, there is no muscle strangulation with repair of the arthrotomy site as seen in some other approaches to the knee, and both vascularity and innervation to the vastus medialis are preserved.
One limitation of this study is that it is a retrospective analysis. Future studies of the consequences of using this approach need to include quantitative assessment of muscle function using electromyography or dynamometer measurements, utilization of any number of validated knee score systems to measure functional outcomes, and incorporation of a randomized controlled trial.
In summary, the new MIV approach is easy to perform and is compatible with more extensile approaches such as a quadriceps snip, if required in revision scenarios. The MIV approach preserves the extensor mechanism, vascularity, and innervation to the vastus medialis. Our study documents both safety and good clinical outcomes using the MIV approach in TKA. Therefore, the MIV approach may be used in the majority of patients undergoing TKA.
- Kurtz S, Ong K, Lau E, Mowat F, Halpern M. Projections of primary and revision hip and knee arthroplasty in the United States from 2005 to 2030. J Bone Joint Surg Am. 2007;89(4):780-785. doi:10.2106/JBJS.F.00222.
- Dutka J, Skowronek M, Sosin P, Skowronek P. Subvastus and medial parapatellar approaches in TKA: Comparison of functional results. Orthopedics. 2011;34(6):148-153. doi:10.3928/01477447-20110427-05.
- Hofmann AA, Plaster RL, Murdock LE. Subvastus (Southern) approach for primary total knee arthroplasty. Clin Orthop Relat Res. 1991;(269):70-77.
- Jojima H, Whiteside LA, Ogata K. Anatomic consideration of nerve supply to the vastus medialis in knee surgery. Clin Orthop Relat Res. 2004;423:157-160. doi:10.1097/01.blo.0000128642.61260.b3.
- Chavan SK, Wabale RN. Reviewing morphology of quadriceps femoris muscle. J Morphol Sci. 2016;33(2):112-117. doi:10.4322/jms.053513.
- Holt G, Nunn T, Allen RA, Forrester AW, Gregori A. Variation of the vastus medialis obliquus insertion and its relevance to minimally invasive total knee arthroplasty. J Arthroplasty. 2008;23(4):600-604. doi:10.1016/j.arth.2007.05.053.
- Sartawi M, Kohlman J, Valle C Della. Modified intervastus approach to the knee. J Knee Surg. 2017;31(05):422-424. doi:10.1055/s-0037-1604150.
- Hu X, Wang G, Pei F, et al. A meta-analysis of the sub-vastus approach and medial parapatellar approach in total knee arthroplasty. Knee Surg Sports Traumatol Arthrosc. 2013;21(10):2398-2404. doi:10.1007/s00167-012-2080-9.
- Jain S, Wasnik S, Mittal A, Hegde C. Outcome of subvastus approach in elderly nonobese patients undergoing bilateral simultaneous total knee arthroplasty: A randomized controlled study. Indian J Orthop. 2013;47(1):45-49. doi:10.4103/0019-5413.106900.
- Kholeif AM, Radwan YA, Mansour AM, Almoalem HA. Early functional results of the subvastus and medial parapatellar approaches in total knee arthroplasty. Med J Cairo Univ. 2017;85(3):849-854.
- Tzatzairis T, Fiska A, Ververidis A, Tilkeridis K, Kazakos K, Drosos GI. Minimally invasive versus conventional approaches in total knee replacement/arthroplasty: A review of the literature. J Orthop. 2018;15(2):459-466. doi:10.1016/j.jor.2018.03.026.
- Li Z, Cheng W, Sun L, Yao Y, Cao Q, Ye S, et al. Mini-subvastus versus medial parapatellar approach for total knee arthroplasty: a prospective randomized controlled study. Int Orthop. 2018;42(3):543-549. doi:10.1007/s00264-017-3703-z.
- Shah NA, Patil HG, Vaishnav VO, Savale A. Total knee arthroplasty using subvastus approach in stiff knee: a retrospective analysis of 110 cases. Indian J Orthop. 2016;50(2):166-171. doi:10.4103/0019-5413.177582.
- Berstock JR, Murray JR, Whitehouse MR, Blom AW, Beswick AD. Medial subvastus versus the medial parapatellar approach for total knee replacement: A systematic review and meta-analysis of randomized controlled trials. EFORT Open Rev. 2018;3(3):78-84. doi:10.1302/2058-5241.3.170030.
- Pan WM, Li XG, Tang TS, Qian ZL, Zhang Q, Zhang CM. Mini-subvastus versus a standard approach in total knee arthroplasty: A prospective, randomized, controlled study. J Int Med Res. 2010;38(3):890-900. doi:10.1177/147323001003800315.
- Bourke MG, Jull GA, Buttrum PJ, Fitzpatrick PL, Dalton PA, Russell TG. Comparing outcomes of medial parapatellar and subvastus approaches in total knee arthroplasty a randomized controlled trial. J Arthroplasty. 2011;27(3):347-353.e1. doi:10.1016/j.arth.2011.06.005.
- Tomek IM, Kantor SR, Cori LAA, et al. Early patient outcomes after primary total knee arthroplasty with quadriceps-sparing subvastus and medial parapatellar techniques: a randomized, double-blind clinical trial. J Bone Jt Surg Am. 2014;96(11):907-915. doi:10.2106/JBJS.L.01578.
- van Hemert WLW, Senden R, Grimm B, van der Linde MJA, Lataster A, Heyligers IC. Early functional outcome after subvastus or parapatellar approach in knee arthroplasty is comparable. Knee Surg Sports Traumatol Arthrosc. 2011;19(6):943-951. doi:10.1007/s00167-010-1292-0.
- Koh IJ, Kim MW, Kim MS, Jang SW, Park DC, In Y. The patient’s perception does not differ following subvastus and medial parapatellar approaches in total knee arthroplasty: a simultaneous bilateral randomized study. J Arthroplasty. 2016;31(1):112-117. doi:10.1016/j.arth.2015.08.004.
- Mehta N, Bhat MS, Goyal A, Mishra P, Joshi D, Chaudhary D. Quadriceps sparing (subvastus/midvastus) approach versus the conventional medial parapatellar approach in primary knee arthroplasty. J Arthrosc Jt Surg. 2017;4(1):15-20. doi:10.1016/j.jajs.2017.02.004.
- Bridgman SA, Walley G, MacKenzie G, Clement D, Griffiths D, Maffulli N. Sub-vastus approach is more effective than a medial parapatellar approach in primary total knee arthroplasty: A randomized controlled trial. Knee. 2009;16(3):216-222. doi:10.1016/j.knee.2008.11.012.
- Liu HW, Gu WD, Xu NW, Sun JY. Surgical approaches in total knee arthroplasty: A meta-analysis comparing the midvastus and subvastus to the medial peripatellar approach. J Arthroplasty. 2014;29(12):2298-2304. doi:10.1016/j.arth.2013.10.023.
- Varela-Egocheaga JR, Suarez-Suarez MA, Fernandez-Villan M, Gonzalez-Sastre V, Varela-Gomez JR, Rodriguez-Merchan C. Minimally invasive subvastus approach: Improving the results of total knee arthroplasty: a prospective, randomized trial. Clin Orthop Relat Res. 2010;468(5):1200-1208. doi:10.1007/s11999-009-1160-8.
ABSTRACT
The subvastus (SV) approach is a well-known muscle- and tendon-sparing approach for total knee arthroplasty (TKA), which has been shown in some studies to provide better outcomes in the visual analog pain score (VAS), knee range of motion (ROM), straight-leg raise, as well as faster rehabilitation, compared with the standard medial parapatellar (MP) approach. We previously described a new knee replacement technique known as the modified intervastus (MIV) approach. The MIV approach is a muscle- and tendon-sparing approach that is extensile and simple to perform. It may be used in the majority of complex primary cases and revisions. Here we describe the surgical technique for performing the MIV approach and provide functional outcome measures. A total of 127 patients (mean age, 66.75 years) underwent TKA using the MIV approach with 1-year follow-up. Clinical outcomes were assessed by recording both a VAS and knee ROM preoperatively, and again at several postoperative time points when the length of time required to ambulate independently (without assistive devices) was also measured. The VAS decreased significantly from the preoperative period (3.69 ± 2.22) to postoperative day 1 (3.17 ± 1.97) (P < .05). Although knee ROM decreased 1 week after surgery, the ROM increased by 6 weeks after surgery compared with the preoperative ROM, and the trend continued over the 1-year follow-up. One-third (33%) of patients were able to walk independently (without assistive devices) at 2 weeks and 78% at 8 weeks. The MIV approach to the knee is a muscle- and tendon-sparing approach that offers advantages over the SV approach and may be used for complex primary and revision total knee cases.
Continue to: Total knee arthroplasty...
Total knee arthroplasty (TKA) is one of the most common orthopedic surgical procedures, with more than 600,000 TKAs performed annually in the United States, and by 2030 the number is expected to reach 3.48 million per year.1Several approaches have been described for total TKA, and the medial parapatellar (MP) approach to the knee is considered the workhorse of total knee replacements. It is an extensile approach that is easy to perform but may delay active knee extension and straight-leg raise after surgery.2 Alternative approaches such as the subvastus (SV) and midvastus approaches to the knee (Figure 1A) typically allow a more rapid straight-leg raise but may be more challenging to perform and time-consuming in morbidly obese patients, muscular patients, and patients with severe deformities.3
The intervastus approach (Figure 1A) described by Jojima4 and others utilizes the interval between the quadriceps tendon and vastus medialis. Although it is a simple approach to perform that is extensile, it is not considered tendon-sparing since the vastus medialis inserts onto the medial aspect of the quadriceps tendon (Figure 2).5 Therefore, dissecting through this interval, without elevation of the vastus medialis (Figure 3A), damages the quadriceps tendon, and strangulation of the muscle occurs with repair of the arthrotomy site (Figure 3B). This situation is even more likely in patients with a low-lying vastus medialis.6
The modified intervastus (MIV) approach described previously,7 may be used in the majority of patients undergoing TKA. The advantages of this approach include its extensile nature, similarities to an MP approach, and preservation of both the extensor mechanism and the vastus medialis, leading to a more rapid return to active knee extension than is traditionally observed.2,7 The approach is simple to perform, easy to close, and is compatible with more extensile approaches such as a quadriceps snip if required in revision scenarios.7 However, functional outcomes of the MIV approach have not been quantified. It is unknown whether these outcomes will offer any advantages compared with the SV approach. Therefore, the objective of this study was to measure functional outcomes of the MIV approach and to compare the results with those previously published for the SV approach. We hypothesized that using the MIV approach for TKA surgery would lead to early straight-leg raise and increase in knee range of motion (ROM) postoperatively.
SURGICAL TECHNIQUE
The MIV approach preserves the quadriceps tendon and vastus medialis.7 After exposure of the vastus medialis muscle, the interval between the quadriceps tendon and vastus medialis is identified (Figure 4). The fascia overlying the lateral edge of the vastus medialis is incised where it meets the quadriceps tendon (Figure 5). The muscle is then bluntly elevated off the underlying capsule just enough to allow for a capsular repair later (Figure 6). An arthrotomy is then performed from cephalad to caudal (Figure 7). This interval may be extended proximally between the vastus intermedius and vastus medialis to expose the distal femur if needed. The closure is performed by repairing the capsule with absorbable suture (Figure 8A), and the vastus medialis fascia is repaired back to the medial edge of the quadriceps tendon, restoring the anatomy (Figure 8B).
Continue to: PATIENTS AND METHODS...
PATIENTS AND METHODS
A retrospective review of functional outcomes after TKA using the MIV approach was conducted; the study was approved by the University of Illinois Institutional Review Board. A total of 127 patients of mean age 66.75 years (range, 48–86 years) with primary osteoarthritis of the knee who were indicated for a total knee replacement with 1-year follow-up were included. The patient demographics are shown in Table 1. All patients underwent TKA using the MIV approach described above by 2 experienced orthopedic surgeons at the same institution. Patients received spinal anesthesia and a periarticular pain block intraoperatively. A measured resection technique was used by 1 surgeon, and a gap-balancing technique by the other. Surgeon 1 used the Persona PS cemented knee system (Zimmer Biomet, and Surgeon 2 used the Sigma PS cemented knee system (Depuy). Patellar resurfacing was done in all cases. Patellar tracking was checked intraoperatively using the ‘no-touch’ technique, and the need for a lateral release was noted. Drains were removed on postoperative day 1. Oral opioids were given as needed. Intravenous antibiotics were continued for 24 hours. Oral anticoagulants were used for thromboembolism prophylaxis for 3 weeks. Patients were mobilized on the day of surgery with full weight-bearing under the supervision of an experienced physical therapist. Static and dynamic quadriceps exercises were started on the same day of surgery along with active knee ROM exercises. Pain score, extensor lag, ROM, walking ability, and complications were recorded in all patients.
Table 1. Patient Demographics
Total no. of patients | 127 | |
Gender | Male | 44 |
Female | 83 | |
Age (years) | Mean ± Standard deviation | 66.75 ± 9.12 |
Range | 48 – 86 | |
Weight (lb) | Mean ± Standard deviation | 218.38 ± 54.47 |
Range | 125 – 364 | |
BMI (kg/m2) | Mean ± Standard deviation | 34.10 ± 7.22 |
Range | 21.1 – 62.5 |
The visual analog score (VAS) was obtained preoperatively and recorded on postoperative day 1. Patient walking distance with assistance was measured on the day of surgery, after surgery, and on the day of hospital discharge. Patients were assessed preoperatively and postoperatively at 1 week, 2 weeks, 6 weeks, 3 months, 6 months, and 1 year for knee ROM. A one-way ANOVA was conducted to compare the preoperative and postoperative day 1 VAS with significance set at P < .05 (OriginPro 2015, OriginLab Corporation). Differences in knee ROM between preoperative and postoperative follow-up periods (1 week, 2 weeks, 6 weeks, 3 months, 6 months, and 1 year) were identified using a 1-way ANOVA with a post hoc Tukey test. Significance was set at P < .05.
RESULTS
All patients were able to fully straight-leg raise and demonstrate functional knee ROM by postoperative day 1. The patella tracked centrally in all patients, and none required a lateral retinacular release. The majority of patients were discharged in the first 48 hours after surgery on oral narcotics. None required IV narcotics during their hospital stay or a blood transfusion. Two cases were complicated by severe knee skin blistering postoperatively due to a reaction to an adhesive dressing; one was complicated by skin necrosis leading to a flap reconstruction that became infected, requiring a 2-stage revision. A separate case had an acute postoperative infection that required irrigation and debridement with polyethylene exchange. After a 12-week course of antibiotics, the infection was eradicated. All patients reported a high satisfaction rate during their acute postoperative phase.
Postoperatively, all patients were able to walk on the day of surgery either independently or with some assistance. On the day of surgery, 10% of patients were able to walk >200 feet, and this increased to 65% of patients able to walk >200 feet on the day of discharge (compare Figure 9A and Figure 9B). Within 2 weeks of surgery, 30% of patients could walk independently (without assistive devices), and this number increased to 78% by 8 weeks after surgery (Figure 10).
Pain assessed using the VAS was lower on postoperative day 1 (3.17 ± 1.97) than the preoperative score (3.69 ± 2.22, P< .05). Overall, knee ROM significantly increased during the follow-up after surgery. Initially, the ROM decreased 1 week after surgery (90.82 ± 10.28) compared with preoperative ROM (101.04 ± 19.48, P < .001) (Figure 10). At 2 weeks after surgery, knee ROM returned to the preoperative value (100.70 ± 13.36). By 6 weeks after surgery, knee ROM was 17° greater than the preoperative ROM (118.45 ± 11.89, P < .001). Knee ROM remained stable at 3- and 6-month assessments, and showed further improvement by 1 year (126.62 ± 9.81, P < .001) compared with the preoperative state (Figure 10). The net improvement in knee ROM was 25° of increased knee flexion by 1 year.
Continue to: DISCUSSION...
DISCUSSION
TKA is a successful procedure that restores knee function with pain relief in osteoarthritis patients. The SV approach for TKA has better outcomes in terms of the VAS, ROM, straight-leg raise with faster rehabilitation compared with the standard MP approach;8–12 however, it can be challenging and time-consuming when used in morbidly obese and muscular patients.3 The SV approach can also increase the risk of complications such as patellar tendon avulsion or medial collateral injury because of the difficulty in exposure specifically for knees with limited ROM.13 Here we introduce the MIV approach as an alternative to the SV approach, overcoming most of these difficulties.
With the prevalence of morbid obesity and the market demand for minimally invasive techniques, we believe the MIV approach represents a good approach for surgeons since it is easy to perform, does not require specialized instrumentation, and is a reproducible approach even on the most complex deformities. The minimal time added to ensure blunt elevation of the vastus medialis muscle and an anatomic repair of the underlying knee capsule and vastus medialis fascia to the medial edge of the quadriceps tendon allows restoration of the anatomy and a robust double-layered watertight seal closure with no strangulation of soft tissues. We believe this reproducible muscle- and tendon-sparing approach that allows gentle, soft tissue handling even in the most complex primary total knee cases may lead to less soft tissue swelling, and therefore, less postoperative pain resulting in an accelerated recovery.
The pain level in this group of patients was reduced after the MIV approach as indicated by the VAS. The VAS was significantly decreased on postoperative day 1 compared with the VAS recorded preoperatively (P < .05), indicating patients felt less pain on the day after surgery. The average VAS on postoperative day 1 from other studies for SV approach ranged from 2.1 to 5,9,12,14–19 whereas our MIV approach value was 3.17. Periarticular blocks were available for this study group, and no peripheral nerve blocks were used. Some studies of the SV approach mention the use of peripheral nerve blocks, while others did not describe the method used for treatment or control of postoperative pain. The decreased reported pain levels and the observed increased knee ROM seen in the MIV and SV approach study groups might be attributable to the treatment of postoperative pain.
Patient ambulation also increased from the day of surgery to the day of discharge for the MIV approach. Only 10% of patients were able to walk with assistance >200 feet on the day of surgery; however, this percentage increased to 65% on the discharge day showing an excellent recovery of walking ability within 2 days of surgery. Mehta and colleagues20reported that 95% of patients undergoing subvastus/midvastus approaches could walk >10 blocks at 6 months follow-up. For the MIV approach, 78% of patients were able to walk independently within 8 weeks of surgery.
Continue to: The MIV approach shows improvement...
The MIV approach shows improvement in functional outcomes in terms of knee ROM from preoperative to postoperative. Although knee ROM decreased significantly at postoperative week 1, which is most likely because of a peak in postoperative pain and swelling; the ROM started to increase significantly at postoperative week 6. After 1 year, the knee ROM increased by 25% compared with preoperative ROM for the MIV approach. We found the average knee ROM at postoperative 3 months using the MIV approach to be 120.4°. Knee ROM at postoperative 3 months using the SV approach reported by other studies ranged from 87.1° to 120°.19,21–23 Similarly, after 1 year, the average ROM by MIV approach was 126.62°, whereas average knee ROM from other studies using the SV approach was 114.1°.2,10,19,21 Achieving a knee ROM of 120° allows patients to return to their baseline function and perform activities of daily living without limitation. With the prevalence of knee osteoarthritis in the younger population, the need for increased knee ROM post-TKA becomes more important so patients can return to work and function at their baseline.
In addition to being both a muscle- and tendon-sparing approach, other advantages of the MIV approach are that it is simple to perform and gives similar exposure to the MP approach even in difficult primary cases. It is also as extensile as the medial parapatellar approach, and it can be converted to a quadriceps snip if required. There is also minimal, if any, muscle retraction preventing muscle injury that may be seen with some other muscle-sparing approaches to the knee, especially in more difficult primary total knee cases. Another advantage to this approach, as opposed to the other approaches to the knee described here, is that it allows a double-layered closure, decreasing the possibility of arthrotomy dehiscence. Because of this double-layered closure, there is no muscle strangulation with repair of the arthrotomy site as seen in some other approaches to the knee, and both vascularity and innervation to the vastus medialis are preserved.
One limitation of this study is that it is a retrospective analysis. Future studies of the consequences of using this approach need to include quantitative assessment of muscle function using electromyography or dynamometer measurements, utilization of any number of validated knee score systems to measure functional outcomes, and incorporation of a randomized controlled trial.
In summary, the new MIV approach is easy to perform and is compatible with more extensile approaches such as a quadriceps snip, if required in revision scenarios. The MIV approach preserves the extensor mechanism, vascularity, and innervation to the vastus medialis. Our study documents both safety and good clinical outcomes using the MIV approach in TKA. Therefore, the MIV approach may be used in the majority of patients undergoing TKA.
ABSTRACT
The subvastus (SV) approach is a well-known muscle- and tendon-sparing approach for total knee arthroplasty (TKA), which has been shown in some studies to provide better outcomes in the visual analog pain score (VAS), knee range of motion (ROM), straight-leg raise, as well as faster rehabilitation, compared with the standard medial parapatellar (MP) approach. We previously described a new knee replacement technique known as the modified intervastus (MIV) approach. The MIV approach is a muscle- and tendon-sparing approach that is extensile and simple to perform. It may be used in the majority of complex primary cases and revisions. Here we describe the surgical technique for performing the MIV approach and provide functional outcome measures. A total of 127 patients (mean age, 66.75 years) underwent TKA using the MIV approach with 1-year follow-up. Clinical outcomes were assessed by recording both a VAS and knee ROM preoperatively, and again at several postoperative time points when the length of time required to ambulate independently (without assistive devices) was also measured. The VAS decreased significantly from the preoperative period (3.69 ± 2.22) to postoperative day 1 (3.17 ± 1.97) (P < .05). Although knee ROM decreased 1 week after surgery, the ROM increased by 6 weeks after surgery compared with the preoperative ROM, and the trend continued over the 1-year follow-up. One-third (33%) of patients were able to walk independently (without assistive devices) at 2 weeks and 78% at 8 weeks. The MIV approach to the knee is a muscle- and tendon-sparing approach that offers advantages over the SV approach and may be used for complex primary and revision total knee cases.
Continue to: Total knee arthroplasty...
Total knee arthroplasty (TKA) is one of the most common orthopedic surgical procedures, with more than 600,000 TKAs performed annually in the United States, and by 2030 the number is expected to reach 3.48 million per year.1Several approaches have been described for total TKA, and the medial parapatellar (MP) approach to the knee is considered the workhorse of total knee replacements. It is an extensile approach that is easy to perform but may delay active knee extension and straight-leg raise after surgery.2 Alternative approaches such as the subvastus (SV) and midvastus approaches to the knee (Figure 1A) typically allow a more rapid straight-leg raise but may be more challenging to perform and time-consuming in morbidly obese patients, muscular patients, and patients with severe deformities.3
The intervastus approach (Figure 1A) described by Jojima4 and others utilizes the interval between the quadriceps tendon and vastus medialis. Although it is a simple approach to perform that is extensile, it is not considered tendon-sparing since the vastus medialis inserts onto the medial aspect of the quadriceps tendon (Figure 2).5 Therefore, dissecting through this interval, without elevation of the vastus medialis (Figure 3A), damages the quadriceps tendon, and strangulation of the muscle occurs with repair of the arthrotomy site (Figure 3B). This situation is even more likely in patients with a low-lying vastus medialis.6
The modified intervastus (MIV) approach described previously,7 may be used in the majority of patients undergoing TKA. The advantages of this approach include its extensile nature, similarities to an MP approach, and preservation of both the extensor mechanism and the vastus medialis, leading to a more rapid return to active knee extension than is traditionally observed.2,7 The approach is simple to perform, easy to close, and is compatible with more extensile approaches such as a quadriceps snip if required in revision scenarios.7 However, functional outcomes of the MIV approach have not been quantified. It is unknown whether these outcomes will offer any advantages compared with the SV approach. Therefore, the objective of this study was to measure functional outcomes of the MIV approach and to compare the results with those previously published for the SV approach. We hypothesized that using the MIV approach for TKA surgery would lead to early straight-leg raise and increase in knee range of motion (ROM) postoperatively.
SURGICAL TECHNIQUE
The MIV approach preserves the quadriceps tendon and vastus medialis.7 After exposure of the vastus medialis muscle, the interval between the quadriceps tendon and vastus medialis is identified (Figure 4). The fascia overlying the lateral edge of the vastus medialis is incised where it meets the quadriceps tendon (Figure 5). The muscle is then bluntly elevated off the underlying capsule just enough to allow for a capsular repair later (Figure 6). An arthrotomy is then performed from cephalad to caudal (Figure 7). This interval may be extended proximally between the vastus intermedius and vastus medialis to expose the distal femur if needed. The closure is performed by repairing the capsule with absorbable suture (Figure 8A), and the vastus medialis fascia is repaired back to the medial edge of the quadriceps tendon, restoring the anatomy (Figure 8B).
Continue to: PATIENTS AND METHODS...
PATIENTS AND METHODS
A retrospective review of functional outcomes after TKA using the MIV approach was conducted; the study was approved by the University of Illinois Institutional Review Board. A total of 127 patients of mean age 66.75 years (range, 48–86 years) with primary osteoarthritis of the knee who were indicated for a total knee replacement with 1-year follow-up were included. The patient demographics are shown in Table 1. All patients underwent TKA using the MIV approach described above by 2 experienced orthopedic surgeons at the same institution. Patients received spinal anesthesia and a periarticular pain block intraoperatively. A measured resection technique was used by 1 surgeon, and a gap-balancing technique by the other. Surgeon 1 used the Persona PS cemented knee system (Zimmer Biomet, and Surgeon 2 used the Sigma PS cemented knee system (Depuy). Patellar resurfacing was done in all cases. Patellar tracking was checked intraoperatively using the ‘no-touch’ technique, and the need for a lateral release was noted. Drains were removed on postoperative day 1. Oral opioids were given as needed. Intravenous antibiotics were continued for 24 hours. Oral anticoagulants were used for thromboembolism prophylaxis for 3 weeks. Patients were mobilized on the day of surgery with full weight-bearing under the supervision of an experienced physical therapist. Static and dynamic quadriceps exercises were started on the same day of surgery along with active knee ROM exercises. Pain score, extensor lag, ROM, walking ability, and complications were recorded in all patients.
Table 1. Patient Demographics
Total no. of patients | 127 | |
Gender | Male | 44 |
Female | 83 | |
Age (years) | Mean ± Standard deviation | 66.75 ± 9.12 |
Range | 48 – 86 | |
Weight (lb) | Mean ± Standard deviation | 218.38 ± 54.47 |
Range | 125 – 364 | |
BMI (kg/m2) | Mean ± Standard deviation | 34.10 ± 7.22 |
Range | 21.1 – 62.5 |
The visual analog score (VAS) was obtained preoperatively and recorded on postoperative day 1. Patient walking distance with assistance was measured on the day of surgery, after surgery, and on the day of hospital discharge. Patients were assessed preoperatively and postoperatively at 1 week, 2 weeks, 6 weeks, 3 months, 6 months, and 1 year for knee ROM. A one-way ANOVA was conducted to compare the preoperative and postoperative day 1 VAS with significance set at P < .05 (OriginPro 2015, OriginLab Corporation). Differences in knee ROM between preoperative and postoperative follow-up periods (1 week, 2 weeks, 6 weeks, 3 months, 6 months, and 1 year) were identified using a 1-way ANOVA with a post hoc Tukey test. Significance was set at P < .05.
RESULTS
All patients were able to fully straight-leg raise and demonstrate functional knee ROM by postoperative day 1. The patella tracked centrally in all patients, and none required a lateral retinacular release. The majority of patients were discharged in the first 48 hours after surgery on oral narcotics. None required IV narcotics during their hospital stay or a blood transfusion. Two cases were complicated by severe knee skin blistering postoperatively due to a reaction to an adhesive dressing; one was complicated by skin necrosis leading to a flap reconstruction that became infected, requiring a 2-stage revision. A separate case had an acute postoperative infection that required irrigation and debridement with polyethylene exchange. After a 12-week course of antibiotics, the infection was eradicated. All patients reported a high satisfaction rate during their acute postoperative phase.
Postoperatively, all patients were able to walk on the day of surgery either independently or with some assistance. On the day of surgery, 10% of patients were able to walk >200 feet, and this increased to 65% of patients able to walk >200 feet on the day of discharge (compare Figure 9A and Figure 9B). Within 2 weeks of surgery, 30% of patients could walk independently (without assistive devices), and this number increased to 78% by 8 weeks after surgery (Figure 10).
Pain assessed using the VAS was lower on postoperative day 1 (3.17 ± 1.97) than the preoperative score (3.69 ± 2.22, P< .05). Overall, knee ROM significantly increased during the follow-up after surgery. Initially, the ROM decreased 1 week after surgery (90.82 ± 10.28) compared with preoperative ROM (101.04 ± 19.48, P < .001) (Figure 10). At 2 weeks after surgery, knee ROM returned to the preoperative value (100.70 ± 13.36). By 6 weeks after surgery, knee ROM was 17° greater than the preoperative ROM (118.45 ± 11.89, P < .001). Knee ROM remained stable at 3- and 6-month assessments, and showed further improvement by 1 year (126.62 ± 9.81, P < .001) compared with the preoperative state (Figure 10). The net improvement in knee ROM was 25° of increased knee flexion by 1 year.
Continue to: DISCUSSION...
DISCUSSION
TKA is a successful procedure that restores knee function with pain relief in osteoarthritis patients. The SV approach for TKA has better outcomes in terms of the VAS, ROM, straight-leg raise with faster rehabilitation compared with the standard MP approach;8–12 however, it can be challenging and time-consuming when used in morbidly obese and muscular patients.3 The SV approach can also increase the risk of complications such as patellar tendon avulsion or medial collateral injury because of the difficulty in exposure specifically for knees with limited ROM.13 Here we introduce the MIV approach as an alternative to the SV approach, overcoming most of these difficulties.
With the prevalence of morbid obesity and the market demand for minimally invasive techniques, we believe the MIV approach represents a good approach for surgeons since it is easy to perform, does not require specialized instrumentation, and is a reproducible approach even on the most complex deformities. The minimal time added to ensure blunt elevation of the vastus medialis muscle and an anatomic repair of the underlying knee capsule and vastus medialis fascia to the medial edge of the quadriceps tendon allows restoration of the anatomy and a robust double-layered watertight seal closure with no strangulation of soft tissues. We believe this reproducible muscle- and tendon-sparing approach that allows gentle, soft tissue handling even in the most complex primary total knee cases may lead to less soft tissue swelling, and therefore, less postoperative pain resulting in an accelerated recovery.
The pain level in this group of patients was reduced after the MIV approach as indicated by the VAS. The VAS was significantly decreased on postoperative day 1 compared with the VAS recorded preoperatively (P < .05), indicating patients felt less pain on the day after surgery. The average VAS on postoperative day 1 from other studies for SV approach ranged from 2.1 to 5,9,12,14–19 whereas our MIV approach value was 3.17. Periarticular blocks were available for this study group, and no peripheral nerve blocks were used. Some studies of the SV approach mention the use of peripheral nerve blocks, while others did not describe the method used for treatment or control of postoperative pain. The decreased reported pain levels and the observed increased knee ROM seen in the MIV and SV approach study groups might be attributable to the treatment of postoperative pain.
Patient ambulation also increased from the day of surgery to the day of discharge for the MIV approach. Only 10% of patients were able to walk with assistance >200 feet on the day of surgery; however, this percentage increased to 65% on the discharge day showing an excellent recovery of walking ability within 2 days of surgery. Mehta and colleagues20reported that 95% of patients undergoing subvastus/midvastus approaches could walk >10 blocks at 6 months follow-up. For the MIV approach, 78% of patients were able to walk independently within 8 weeks of surgery.
Continue to: The MIV approach shows improvement...
The MIV approach shows improvement in functional outcomes in terms of knee ROM from preoperative to postoperative. Although knee ROM decreased significantly at postoperative week 1, which is most likely because of a peak in postoperative pain and swelling; the ROM started to increase significantly at postoperative week 6. After 1 year, the knee ROM increased by 25% compared with preoperative ROM for the MIV approach. We found the average knee ROM at postoperative 3 months using the MIV approach to be 120.4°. Knee ROM at postoperative 3 months using the SV approach reported by other studies ranged from 87.1° to 120°.19,21–23 Similarly, after 1 year, the average ROM by MIV approach was 126.62°, whereas average knee ROM from other studies using the SV approach was 114.1°.2,10,19,21 Achieving a knee ROM of 120° allows patients to return to their baseline function and perform activities of daily living without limitation. With the prevalence of knee osteoarthritis in the younger population, the need for increased knee ROM post-TKA becomes more important so patients can return to work and function at their baseline.
In addition to being both a muscle- and tendon-sparing approach, other advantages of the MIV approach are that it is simple to perform and gives similar exposure to the MP approach even in difficult primary cases. It is also as extensile as the medial parapatellar approach, and it can be converted to a quadriceps snip if required. There is also minimal, if any, muscle retraction preventing muscle injury that may be seen with some other muscle-sparing approaches to the knee, especially in more difficult primary total knee cases. Another advantage to this approach, as opposed to the other approaches to the knee described here, is that it allows a double-layered closure, decreasing the possibility of arthrotomy dehiscence. Because of this double-layered closure, there is no muscle strangulation with repair of the arthrotomy site as seen in some other approaches to the knee, and both vascularity and innervation to the vastus medialis are preserved.
One limitation of this study is that it is a retrospective analysis. Future studies of the consequences of using this approach need to include quantitative assessment of muscle function using electromyography or dynamometer measurements, utilization of any number of validated knee score systems to measure functional outcomes, and incorporation of a randomized controlled trial.
In summary, the new MIV approach is easy to perform and is compatible with more extensile approaches such as a quadriceps snip, if required in revision scenarios. The MIV approach preserves the extensor mechanism, vascularity, and innervation to the vastus medialis. Our study documents both safety and good clinical outcomes using the MIV approach in TKA. Therefore, the MIV approach may be used in the majority of patients undergoing TKA.
- Kurtz S, Ong K, Lau E, Mowat F, Halpern M. Projections of primary and revision hip and knee arthroplasty in the United States from 2005 to 2030. J Bone Joint Surg Am. 2007;89(4):780-785. doi:10.2106/JBJS.F.00222.
- Dutka J, Skowronek M, Sosin P, Skowronek P. Subvastus and medial parapatellar approaches in TKA: Comparison of functional results. Orthopedics. 2011;34(6):148-153. doi:10.3928/01477447-20110427-05.
- Hofmann AA, Plaster RL, Murdock LE. Subvastus (Southern) approach for primary total knee arthroplasty. Clin Orthop Relat Res. 1991;(269):70-77.
- Jojima H, Whiteside LA, Ogata K. Anatomic consideration of nerve supply to the vastus medialis in knee surgery. Clin Orthop Relat Res. 2004;423:157-160. doi:10.1097/01.blo.0000128642.61260.b3.
- Chavan SK, Wabale RN. Reviewing morphology of quadriceps femoris muscle. J Morphol Sci. 2016;33(2):112-117. doi:10.4322/jms.053513.
- Holt G, Nunn T, Allen RA, Forrester AW, Gregori A. Variation of the vastus medialis obliquus insertion and its relevance to minimally invasive total knee arthroplasty. J Arthroplasty. 2008;23(4):600-604. doi:10.1016/j.arth.2007.05.053.
- Sartawi M, Kohlman J, Valle C Della. Modified intervastus approach to the knee. J Knee Surg. 2017;31(05):422-424. doi:10.1055/s-0037-1604150.
- Hu X, Wang G, Pei F, et al. A meta-analysis of the sub-vastus approach and medial parapatellar approach in total knee arthroplasty. Knee Surg Sports Traumatol Arthrosc. 2013;21(10):2398-2404. doi:10.1007/s00167-012-2080-9.
- Jain S, Wasnik S, Mittal A, Hegde C. Outcome of subvastus approach in elderly nonobese patients undergoing bilateral simultaneous total knee arthroplasty: A randomized controlled study. Indian J Orthop. 2013;47(1):45-49. doi:10.4103/0019-5413.106900.
- Kholeif AM, Radwan YA, Mansour AM, Almoalem HA. Early functional results of the subvastus and medial parapatellar approaches in total knee arthroplasty. Med J Cairo Univ. 2017;85(3):849-854.
- Tzatzairis T, Fiska A, Ververidis A, Tilkeridis K, Kazakos K, Drosos GI. Minimally invasive versus conventional approaches in total knee replacement/arthroplasty: A review of the literature. J Orthop. 2018;15(2):459-466. doi:10.1016/j.jor.2018.03.026.
- Li Z, Cheng W, Sun L, Yao Y, Cao Q, Ye S, et al. Mini-subvastus versus medial parapatellar approach for total knee arthroplasty: a prospective randomized controlled study. Int Orthop. 2018;42(3):543-549. doi:10.1007/s00264-017-3703-z.
- Shah NA, Patil HG, Vaishnav VO, Savale A. Total knee arthroplasty using subvastus approach in stiff knee: a retrospective analysis of 110 cases. Indian J Orthop. 2016;50(2):166-171. doi:10.4103/0019-5413.177582.
- Berstock JR, Murray JR, Whitehouse MR, Blom AW, Beswick AD. Medial subvastus versus the medial parapatellar approach for total knee replacement: A systematic review and meta-analysis of randomized controlled trials. EFORT Open Rev. 2018;3(3):78-84. doi:10.1302/2058-5241.3.170030.
- Pan WM, Li XG, Tang TS, Qian ZL, Zhang Q, Zhang CM. Mini-subvastus versus a standard approach in total knee arthroplasty: A prospective, randomized, controlled study. J Int Med Res. 2010;38(3):890-900. doi:10.1177/147323001003800315.
- Bourke MG, Jull GA, Buttrum PJ, Fitzpatrick PL, Dalton PA, Russell TG. Comparing outcomes of medial parapatellar and subvastus approaches in total knee arthroplasty a randomized controlled trial. J Arthroplasty. 2011;27(3):347-353.e1. doi:10.1016/j.arth.2011.06.005.
- Tomek IM, Kantor SR, Cori LAA, et al. Early patient outcomes after primary total knee arthroplasty with quadriceps-sparing subvastus and medial parapatellar techniques: a randomized, double-blind clinical trial. J Bone Jt Surg Am. 2014;96(11):907-915. doi:10.2106/JBJS.L.01578.
- van Hemert WLW, Senden R, Grimm B, van der Linde MJA, Lataster A, Heyligers IC. Early functional outcome after subvastus or parapatellar approach in knee arthroplasty is comparable. Knee Surg Sports Traumatol Arthrosc. 2011;19(6):943-951. doi:10.1007/s00167-010-1292-0.
- Koh IJ, Kim MW, Kim MS, Jang SW, Park DC, In Y. The patient’s perception does not differ following subvastus and medial parapatellar approaches in total knee arthroplasty: a simultaneous bilateral randomized study. J Arthroplasty. 2016;31(1):112-117. doi:10.1016/j.arth.2015.08.004.
- Mehta N, Bhat MS, Goyal A, Mishra P, Joshi D, Chaudhary D. Quadriceps sparing (subvastus/midvastus) approach versus the conventional medial parapatellar approach in primary knee arthroplasty. J Arthrosc Jt Surg. 2017;4(1):15-20. doi:10.1016/j.jajs.2017.02.004.
- Bridgman SA, Walley G, MacKenzie G, Clement D, Griffiths D, Maffulli N. Sub-vastus approach is more effective than a medial parapatellar approach in primary total knee arthroplasty: A randomized controlled trial. Knee. 2009;16(3):216-222. doi:10.1016/j.knee.2008.11.012.
- Liu HW, Gu WD, Xu NW, Sun JY. Surgical approaches in total knee arthroplasty: A meta-analysis comparing the midvastus and subvastus to the medial peripatellar approach. J Arthroplasty. 2014;29(12):2298-2304. doi:10.1016/j.arth.2013.10.023.
- Varela-Egocheaga JR, Suarez-Suarez MA, Fernandez-Villan M, Gonzalez-Sastre V, Varela-Gomez JR, Rodriguez-Merchan C. Minimally invasive subvastus approach: Improving the results of total knee arthroplasty: a prospective, randomized trial. Clin Orthop Relat Res. 2010;468(5):1200-1208. doi:10.1007/s11999-009-1160-8.
- Kurtz S, Ong K, Lau E, Mowat F, Halpern M. Projections of primary and revision hip and knee arthroplasty in the United States from 2005 to 2030. J Bone Joint Surg Am. 2007;89(4):780-785. doi:10.2106/JBJS.F.00222.
- Dutka J, Skowronek M, Sosin P, Skowronek P. Subvastus and medial parapatellar approaches in TKA: Comparison of functional results. Orthopedics. 2011;34(6):148-153. doi:10.3928/01477447-20110427-05.
- Hofmann AA, Plaster RL, Murdock LE. Subvastus (Southern) approach for primary total knee arthroplasty. Clin Orthop Relat Res. 1991;(269):70-77.
- Jojima H, Whiteside LA, Ogata K. Anatomic consideration of nerve supply to the vastus medialis in knee surgery. Clin Orthop Relat Res. 2004;423:157-160. doi:10.1097/01.blo.0000128642.61260.b3.
- Chavan SK, Wabale RN. Reviewing morphology of quadriceps femoris muscle. J Morphol Sci. 2016;33(2):112-117. doi:10.4322/jms.053513.
- Holt G, Nunn T, Allen RA, Forrester AW, Gregori A. Variation of the vastus medialis obliquus insertion and its relevance to minimally invasive total knee arthroplasty. J Arthroplasty. 2008;23(4):600-604. doi:10.1016/j.arth.2007.05.053.
- Sartawi M, Kohlman J, Valle C Della. Modified intervastus approach to the knee. J Knee Surg. 2017;31(05):422-424. doi:10.1055/s-0037-1604150.
- Hu X, Wang G, Pei F, et al. A meta-analysis of the sub-vastus approach and medial parapatellar approach in total knee arthroplasty. Knee Surg Sports Traumatol Arthrosc. 2013;21(10):2398-2404. doi:10.1007/s00167-012-2080-9.
- Jain S, Wasnik S, Mittal A, Hegde C. Outcome of subvastus approach in elderly nonobese patients undergoing bilateral simultaneous total knee arthroplasty: A randomized controlled study. Indian J Orthop. 2013;47(1):45-49. doi:10.4103/0019-5413.106900.
- Kholeif AM, Radwan YA, Mansour AM, Almoalem HA. Early functional results of the subvastus and medial parapatellar approaches in total knee arthroplasty. Med J Cairo Univ. 2017;85(3):849-854.
- Tzatzairis T, Fiska A, Ververidis A, Tilkeridis K, Kazakos K, Drosos GI. Minimally invasive versus conventional approaches in total knee replacement/arthroplasty: A review of the literature. J Orthop. 2018;15(2):459-466. doi:10.1016/j.jor.2018.03.026.
- Li Z, Cheng W, Sun L, Yao Y, Cao Q, Ye S, et al. Mini-subvastus versus medial parapatellar approach for total knee arthroplasty: a prospective randomized controlled study. Int Orthop. 2018;42(3):543-549. doi:10.1007/s00264-017-3703-z.
- Shah NA, Patil HG, Vaishnav VO, Savale A. Total knee arthroplasty using subvastus approach in stiff knee: a retrospective analysis of 110 cases. Indian J Orthop. 2016;50(2):166-171. doi:10.4103/0019-5413.177582.
- Berstock JR, Murray JR, Whitehouse MR, Blom AW, Beswick AD. Medial subvastus versus the medial parapatellar approach for total knee replacement: A systematic review and meta-analysis of randomized controlled trials. EFORT Open Rev. 2018;3(3):78-84. doi:10.1302/2058-5241.3.170030.
- Pan WM, Li XG, Tang TS, Qian ZL, Zhang Q, Zhang CM. Mini-subvastus versus a standard approach in total knee arthroplasty: A prospective, randomized, controlled study. J Int Med Res. 2010;38(3):890-900. doi:10.1177/147323001003800315.
- Bourke MG, Jull GA, Buttrum PJ, Fitzpatrick PL, Dalton PA, Russell TG. Comparing outcomes of medial parapatellar and subvastus approaches in total knee arthroplasty a randomized controlled trial. J Arthroplasty. 2011;27(3):347-353.e1. doi:10.1016/j.arth.2011.06.005.
- Tomek IM, Kantor SR, Cori LAA, et al. Early patient outcomes after primary total knee arthroplasty with quadriceps-sparing subvastus and medial parapatellar techniques: a randomized, double-blind clinical trial. J Bone Jt Surg Am. 2014;96(11):907-915. doi:10.2106/JBJS.L.01578.
- van Hemert WLW, Senden R, Grimm B, van der Linde MJA, Lataster A, Heyligers IC. Early functional outcome after subvastus or parapatellar approach in knee arthroplasty is comparable. Knee Surg Sports Traumatol Arthrosc. 2011;19(6):943-951. doi:10.1007/s00167-010-1292-0.
- Koh IJ, Kim MW, Kim MS, Jang SW, Park DC, In Y. The patient’s perception does not differ following subvastus and medial parapatellar approaches in total knee arthroplasty: a simultaneous bilateral randomized study. J Arthroplasty. 2016;31(1):112-117. doi:10.1016/j.arth.2015.08.004.
- Mehta N, Bhat MS, Goyal A, Mishra P, Joshi D, Chaudhary D. Quadriceps sparing (subvastus/midvastus) approach versus the conventional medial parapatellar approach in primary knee arthroplasty. J Arthrosc Jt Surg. 2017;4(1):15-20. doi:10.1016/j.jajs.2017.02.004.
- Bridgman SA, Walley G, MacKenzie G, Clement D, Griffiths D, Maffulli N. Sub-vastus approach is more effective than a medial parapatellar approach in primary total knee arthroplasty: A randomized controlled trial. Knee. 2009;16(3):216-222. doi:10.1016/j.knee.2008.11.012.
- Liu HW, Gu WD, Xu NW, Sun JY. Surgical approaches in total knee arthroplasty: A meta-analysis comparing the midvastus and subvastus to the medial peripatellar approach. J Arthroplasty. 2014;29(12):2298-2304. doi:10.1016/j.arth.2013.10.023.
- Varela-Egocheaga JR, Suarez-Suarez MA, Fernandez-Villan M, Gonzalez-Sastre V, Varela-Gomez JR, Rodriguez-Merchan C. Minimally invasive subvastus approach: Improving the results of total knee arthroplasty: a prospective, randomized trial. Clin Orthop Relat Res. 2010;468(5):1200-1208. doi:10.1007/s11999-009-1160-8.
TAKE-HOME POINTS
- The modified intervastus approach to the knee spares the quadriceps tendon and the vastus medialis muscle.
- The modified intervastus approach is an extensile approach.
- The modified intersvastus approach is a safe approach to the knee that can be used in total knee arthroplasty and leads to early straight-leg raise and a rapid recovery.
- The modified intervastus approach can be used on the majority of patients requiring a total knee replacement.
- The modified intervastus approach utilizes a unique closure that avoids soft tissue strangulation.
Review of Common Clinical Conditions of the Proximal Tibiofibular Joint
ABSTRACT
Current literature is limited with respect to the proximal tibiofibular joint (PTFJ) and clinical conditions relating to the PTFJ. Diagnosis and treatment of conditions that affect the PTFJ are not well described and are a topic of debate among many physicians. This manuscript aims to review and summarize the most recent literature that relates to traumatic dislocations, fractures, chronic instability, and osteoarthritis, with a focus on both diagnostic and treatment strategies of these conditions. We also review PTFJ anatomy, biomechanics, and the clinical presentation of some common PTFJ conditions.
Continue to: Clinical conditions...
Clinical conditions of the proximal tibiofibular joint (PTFJ) are an uncommon source of lateral knee complaints and are often overlooked in the differential diagnosis as a source of the knee complaint. The most common conditions of the PTFJ include traumatic dislocations, fractures, chronic instability, and osteoarthritis. This article reviews the most common diseases affecting this joint and discusses both diagnostic and treatment strategies in an attempt to raise awareness of this joint as a source of lateral knee complaints.
ANATOMY
The PTFJ is an arthrodial synovial joint between the posterolateral surface of the tibia and the proximal fibular head.1 Surrounding the synovial membrane of the articulation is a fibrous joint capsule with distinct anterior and posterior tibiofibular ligaments.2,3 The anterior tibiofibular ligament has been described as 1 or 2 bands whereas the posterior ligament consists of 1 band.3 The anterior ligament attaches anteroinferiorly to the fibular styloid and posteriorly to Gerdy’s tubercle on the tibia. It runs linearly from posterior to anterior, and the fibular footprint is immediately anterior to the insertion of the biceps femoris. The posterior ligament is located inferior to the lateral joint space, and the fibular footprint is posterior to the insertion of the biceps femoris.3 Anatomy of the PTFJ is shown schematically in Figure 1.
Both the lateral collateral ligament (LCL) and the tibiofibular interosseous membrane add stability to the PTFJ. The LCL travels from the lateral femoral epicondyle to the lateral side of fibula head, anterior to the fibular styloid. The interosseous membrane extends obliquely between the borders of the tibia and fibula. Additionally, the short head of the biceps femoris, fibular collateral ligament, fabellofibular ligament, popliteofibular ligament, and popliteus muscle all attach to the PTFJ and provide additional stability to the joint.
It is important to note that the common peroneal nerve passes posteriorly over the fibula neck, can be involved in the clinical presentation, and is a potential source of concern with any injury to or surgery on the joint.4
Many studies have demonstrated that a communication with the tibiofemoral joint exists through the subpopliteal recess, but the rate of communication has varied widely.5-8 Most recently, Bozkurt and colleagues5 found the rate of communication between the PTFJ and lateral femorotibial space to be 57.9%. When distinct communication exists, the PTFJ must be considered as a fourth compartment of the knee and is subject to any process that affects the knee joint proper.
Continue to: Ogden described 2 types...
Ogden1 described 2 types of PTFJs, horizontal and oblique, with the latter being considered less stable because of less rotational mobility. The horizontal configuration is defined as <20° of inclination of joint surface in relation to the horizontal plane, and the oblique variation is defined as >20° of inclination of the joint surface in relation to the horizontal plane.1
BIOMECHANICS AND FUNCTION
The primary function of the PTFJ is to dissipate torsional loads applied to the ankle, attenuate lateral tibial bending moments, and transmit axial loads from weight bearing on the extremity.1 The degree of knee flexion, ankle dorsiflexion, and tibial rotation all play an important role in PTFJ biomechanics. In knee flexion, the proximal fibula moves anteriorly because of the relative laxity of the LCL and biceps femoris tendons. In knee extension, the LCL and biceps femoris tighten, pulling the proximal fibula posteriorly.1 Because the LCL and biceps tendon are both relaxed and less supportive during knee flexion, the PTFJ is more prone to injury with a flexed knee. The ankle plays an important role in the biomechanics of the PTFJ because it contains the distal syndesmosis, where both the tibia and fibula are firmly attached distally. During ankle dorsiflexion, the fibula must externally rotate to accommodate a wider anterior talus.1 In regard to tibia rotation, Scott and colleagues9 demonstrated the relationship between tibial rotation and fibular translation. With internal tibial rotation, the fibular head translated posteriorly and with external tibial rotation, the fibula translated anteriorly. The greatest translational motion was seen during loading of the knee into varus and during external tibial rotation at all flexion angles.
CLINICAL CONDITIONS
Ogden10 classified instability of the PTFJ into 4 main groups: anterolateral dislocation, posteromedial dislocation, superior dislocation, and atraumatic subluxation. Injury to the PTFJ usually occurs in younger, athletic patients during sports that require violent twisting motions such as soccer, basketball, dance, skiing, horseback riding, parachute jumping, jet skiing, and judo. Patients with generalized ligamentous laxity have been described as at increased risk for joint instability.10,11
ACUTE DISLOCATION
The most common injury to the PTFJ is an anterolateral dislocation and involves injury to both the anterior and posterior capsular ligaments, and occasionally the LCL.10 Anterolateral dislocation is usually the result of a fall on a hyperflexed knee with the foot inverted and plantarflexed.11 While most anterolateral dislocations are the result of indirect sports trauma, several have been associated with other types of skeletal injuries such as fracture-dislocation of the hip, crush injury of the proximal and distal ends of the tibia, fracture-dislocation of the ankle, proximal tibial fracture, and fracture-dislocation of the distal femoral epiphysis.10 Ogden10 described the mechanism as follows: (1) sudden inversion and plantar flexion of the foot causing tension in the peroneal muscle group, extensor digitorum longus, and extensor halluces longus, which applies a forward dislocating force to the proximal end of the fibula; (2) simultaneous flexion of the knee, relaxing the biceps tendon and LCL; and (3) twisting of the body over the knee, transmitting energy along the femur to the tibia, exerting a relative external rotatory torque of the tibia on the foot, which is already fixed in inversion. Steps (2) and (3) spring the proximal end of the fibula laterally while the contracting muscles of (1) pull the fibula anteriorly.
Posteromedial dislocation is the second most common type of acute PTFJ dislocation. Posteromedial dislocations usually involve direct trauma and are associated with peroneal nerve injuries.1,2,10 The mechanism of dislocation results in tearing of the anterior and posterior PTFJ capsular ligaments, followed by injury to the LCL and other surrounding ligaments. This allows the biceps femoris to draw the unsupported proximal part of the fibula posteromedially along the posterolateral tibial metaphysis.7
Continue to: Superior dislocations are...
Superior dislocations are the least frequent form of acute PTFJ dislocations and are associated with high-energy ankle injuries.2,10,12 Superior dislocation results in injury to the interosseous membrane between the tibia and fibula and is frequently associated with tibial shaft fracture.10,11 Atraumatic, acquired superior dislocation of the PTFJ has also been associated with congenital dislocation of the knee.10,11
SUBLUXATION/CHRONIC INSTABILITY
Subluxation of the PTFJ classically involves excessive and symptomatic anterior-posterior motion without actual dislocation of the joint.11 Subluxations of the PTFJ typically occur without any known trauma or injury and are most frequently associated with benign hyperlaxity syndrome, Ehlers-Danlos syndrome, or muscular dystrophy.4,10
Semonian and colleagues13 suggest that subluxation of the PTFJ is not given enough recognition in the literature and that instability should not be considered a rare condition. They hypothesize that many patients have joints that do not tolerate increases in fibula rotation secondary to subclinical trauma, repetitive overuse, or biomechanical variation of the joint. Semonian and associates13 state that the condition begins with the anterior capsule and anterior tibiofibular ligament attenuation as a result of excessive fibular rotation. Once stretched, the functional pull of the biceps femoris and soleus maintain the fibula in a relatively posterior and externally rotated position. Furthermore, Ogden10 found that 70% of the dislocations and subluxations he studied were of the oblique variant compared with that of the horizontal variant. Many authors suggest that the oblique variant is more at risk for injury because of its decreased joint surface area causing decreased rotational mobility.1,2,10
Early recognition is extremely important in dislocations and subluxations of the PTFJ as undiagnosed acute trauma can turn into chronic subluxation, and chronic subluxation may lead to dislocation.13 Additionally, chronic subluxation or dislocation are thought result in osteoarthritis of the PTFJ.14
OSTEOARTHRITIS
The literature on osteoarthritis of the PTFJ is limited. Eichenblat and Nathan7 studied the PTFJ in cadavers and dry bones and found that 28% had evidence of osteoarthritis. Clinically, however, osteoarthritis of the PTFJ is a rare primary diagnosis, suggesting that involvement of the PTFJ is either asymptomatic or that symptoms are associated with osteoarthritis of the knee joint. Boya and colleagues15 and Eichenblat and Nathan7 both found a high correlation between the presence of osteoarthritis of the PTFJ and osteoarthritis of the tibiofemoral joint in cadavers. The authors suggest this correlation may be related to the presence of anatomical communication between the 2 joints. Theoretically, inflammatory mediators flow freely between the joint spaces and contribute to arthritis in both joints. The possibility of degenerative arthritis of the PTFJ accompanying degenerative arthritis of the knee warrants evaluation, especially in patients considering total knee arthroplasty. Unrecognized arthritis of the PTFJ might influence outcome scores and be an unsolved source of lateral knee pain post-knee replacement.16
Continue to: CLINICAL PRESENTATION
CLINCIAL PRESENTATION
ACUTE DISLOCATION
Patients with acute PTFJ dislocation present with pain, tenderness, swelling, and asymmetry of the lateral side of the knee, while the knee joint is not swollen and range of knee motion is not limited.17 A bony prominence might be felt, and the biceps femoris tendon can often appear to be tense.13 Active or passive ankle movements often exacerbate the lateral knee pain.11 It is also important to examine the peroneal nerve, as transient peroneal palsy has been described in all types of PTFJ dislocations but most often with posteromedial dislocations. Sensory disturbance in the peroneal nerve distribution is more common than motor loss, but foot drop is also a potential presenting sign.11 On examination, palpation of the fibular head illustrates tenderness and aggravates the pain.
SUBLUXATION/CHRONIC INSTABILITY
Subluxation of the PTFJ can be difficult to recognize because the history, signs, and symptoms of lateral knee pain can be subtle and sometimes misleading. In addition, current literature provides little information on specific tests, measurements, signs, or subjective information regarding subluxation. Patients rarely reveal a history of trauma or mechanism of injury. Subluxations are often associated with patients participating in repetitive sports requiring running, jumping, or twisting movements, and can be present bilateral. Instability has also been described in patients with osteomyelitis, rheumatoid arthritis, septic arthritis, pigmented villonodular synovitis, below-knee amputations, osteochondroma, and in runners who recently increased mileage (especially during the first 2-3 miles and during downhill running).11,13 Patients normally do not have difficulty with activities of daily living, but symptoms may arise when making movements with a sudden change of direction.11
These patients usually complain of instability of the knee and pain along the lateral aspect of the knee. Pain radiating proximally into the region of the iliotibial band and medially into the patellofemoral joint can be seen.13 Patients may also report clicking, popping, or catching of the lateral knee; while others will report a sense of giving way of the knee joint.11,13 Progressive peroneal nerve symptoms are usually seen in older patients; however, they are more common with acute PTFJ dislocations as discussed.13
A clinical method for examining a PTFJ with possible subluxation or chronic instability has been described by Sijbrandij.18 With the patient in the supine position, the knee is flexed to 90° to relax the LCL and biceps femoris tendon. The fibular head is then held between the thumb and index finger, and moved anteriorly and laterally. Dislocation or subluxation will be felt and visualized as the fibular head translates, and should be compared with the uninjured PTFJ. On release, the fibular head will return to its normal position, often with a click. Asking the patient if this subluxation/reduction maneuver reproduces the symptoms or causes apprehension or pain may also be helpful.18 Another method for examination is eliciting the Radulescu sign.11,13 While the patient lies prone, the examiner stabilizes the thigh with 1 hand while the knee is flexed to 90°. The examiner then applies an internal rotation force on the lower leg. Observing an abnormal excursion of the fibular head in an anterior and lateral direction represents a positive test.11,13
OSTEOARTHRITIS
Clinical evaluation for osteoarthritis in the PTFJ is not well described in the literature. A single report describes applying manual pressure over the fibular head during active ankle motion.16 A test known as the grinding test is used as a sign to detect the involvement of the PTFJ as a component of osteoarthritis of the knee. A positive test will elicit pain and/or tenderness of the joint.16
Continue to: DIAGNOSTIC IMAGING
DIAGNOSTIC IMAGING
Plain radiographs in the anteroposterior (AP) and true lateral views are useful as first-line investigations in suspected PTFJ dislocation. Comparable AP and lateral radiographs of each knee are highly recommended to detect findings that suggest dislocation.2 Abnormal findings include increased interosseous space, medial or lateral displacement of the fibula on the anteroposterior view, and anterior or posterior displacement of the fibula head on lateral view as shown in Figure 2.19,20
Resnick and colleagues2 proposed the use of the linear sloping radiodensity that defines the posteromedial corner of the lateral tibial condyle as an indicator of anterolateral or posteromedial PTFJ dislocation. However, this application is limited because of the PTFJ’s highly variable morphology.8 In a recent study conducted by Hey and colleagues,21 5968 (2984 patients) knee radiographs were retrospectively collected and subjected to radiographical measurements and statistical analysis. The tibiofibular overlap method had a specificity of 94.1% and 84.5% when diagnosing PTFJ dislocations on the AP and lateral views, respectively.21
If a diagnosis of PTFJ is suspected but not clearly established based on radiography, computed tomography with comparison views of the contralateral knee are recommended to confirm the diagnosis.17,22 This becomes more critical in cases of suspected subluxation/chronic PTFJ instability. Additionally, magnetic resonance imaging (MRI) can be used to assess chronic PTFJ instability. Recently, Burke and colleagues23 performed a 10-year retrospective case series that included 7 patients with chronic PTFJ instability and included MRI as part of their evaluation. The MRI abnormalities in these patients included periarticular soft tissue edema, including in the proximal soleus muscle (n = 5), periarticular ganglion or ganglia (n = 4), tibiofibular ligament edema (n = 4), subchondral marrow edema (n = 3), posterior tibiofibular ligament thickening (n = 2), subcortical cyst at a ligament insertion (n = 2), partial-thickness tear of the anterior tibiofibular ligament (n = 1), and tibiofibular joint effusion (n = 1).
OSTEOARTHRITIS
Routine knee radiographs can show PTFJ joint space narrowing, sclerosis, marginal osteophytes, and local osteopenia as conventional components of osteoarthritis of any joint. Serial radiographs have also been described as effective in evaluating progressive degenerative changes of the PTFJ.14 An MRI will show osteophyte formation, subchondral cysts, subchondral sclerosis, joint effusion, joint space narrowing, and is highly sensitive for detecting degenerative changes in cartilage, as well as identifying other possible pathologies such as synovial cysts or pigmented villonodular synovitis. Chronic PTFJ instability appears to predispose to tibiofibular osteoarthritis as reported by Burke and colleagues,23 who found a particularly high incidence (42.9%) of osteoarthritis in patients with chronic PTFJ instability. Additionally, Veth and colleagues14 found degenerative changes in 8 of 19 patients presenting with PTFJ dislocations.
TREATMENT
ACUTE DISLOCATION
Prompt recognition and treatment of any acute PTFJ dislocation are necessary to avoid long-term instability and other possible sequelae.11 Treatment consists of reduction followed by restriction of weight-bearing.11 Traditionally, the knee is immobilized with a cast in extension for 3 to 4 weeks followed by knee mobilization and progressive range of motion exercises,24 but there is some controversy regarding complete immobilization.11,25,26
Continue to: Initially, closed reduction...
Initially, closed reduction is advised as the treatment for acute PTFJ dislocation.11,19,24 It involves placing the knee in 80°to 110° of flexion to relax the biceps femoris and LCL, then applying an appropriate force to the fibular head in a direction opposite the displacement.11,24 An audible pop is often heard as the fibula reduces back into normal alignment. Stability of the reduction and stability of the knee should be determined with respect to both posterolateral structures and the LCL after reduction.11 Anterolateral dislocations are usually easier to reduce, as a posteromedial or superior dislocation can result in the fibular head being perched on the lateral tibial ridge, and held by the LCL.11
Calabró and colleagues27 described a new, simple, and safe alternative technique of closed reduction of an anterior dislocation if the classical method fails. This technique relies on ligamentotaxis and a dynamic counteraction between muscles and ligaments to reduce the joint. The patient flexes the knee >90° while the physician applies a counterforce to the heel with the palm. Simultaneously, gentle direct pressure should be applied to the fibular head to move it toward the lateral tibial ridge. With a relaxed LCL, the biceps femoris tendon will actively reduce the proximal fibular head back into its correct anatomic orientation.27
When a closed reduction in an awake patient has failed, a reduction under sedation or anesthesia should be performed. If that fails, an open reduction should be performed. Following an open reduction, the joint is stabilized with Kirschner wires, bioabsorbable pins, or cortical screws.24-26,28 The torn capsule and any injured ligaments should also be primarily repaired. After approximately 6 weeks, the stabilization hardware may be removed.24,25
Acute posteromedial dislocations are treated similarly to anterolateral dislocations; however, open reduction and repair of the capsule and ligaments are more frequently required.10 Superior dislocations are also more frequently reduced by open methods, sometimes dictated by open treatment of associated tibia or ankle fracture.10 Damage to any structure of the posterolateral knee as a result of acute PTFJ injury should be repaired, as this has been associated with better outcomes.14
PTFJ dislocation and tibia fracture can occur together, and in a retrospective study conducted by Herzog and colleagues29 the authors recorded the incidence of PTFJ dislocation as 1.5% of operative tibial shaft fractures and 1.9% of operative tibial plateau fractures. Haupt and colleagues30 also conducted a retrospective study in which the authors recorded the incidence of PTFJ dislocations in 1.06% of all tibial shaft fractures in their series. In both studies combined, all except 1 PTFJ dislocation had been caused by high-energy trauma.29,30 In the case of PTFJ dislocation with tibial shaft fracture, intramedullary nailing of the tibial shaft fracture followed by open reduction of the PTFJ with 1 or 2 positioning screws just below the PTFJ has yielded satisfactory results.30 The positioning screw should be removed 6 weeks post-operation to prevent PTFJ arthrodesis and patients should be supported in full weight-bearing.30 An illustrative case report from our institution is shown in Figure 3.
Continue to: CHRONIC INSTABILITY
CHRONIC INSTABILITY
Chronic instability is commonly the result of untreated or misdiagnosed subluxation of the PTFJ. Ogden10 reported that 57% of patients with acute proximal tibiofibular dislocations required surgery for ongoing symptoms after treatment failure with closed reduction and 3 weeks of immobilization. The first step in the management of chronic instability of the PTFJ is usually a nonoperative approach. Immobilization, activity level modification, utilization of a supportive strap placed 1 centimeter below the fibular head for pain relief, and participation in a strength-training program of the lower leg are initial treatment recommendations.10,11,13 Many patients with chronic PTFJ instability do not respond to conservative treatment and may pursue surgical intervention. Surgical treatment options include permanent arthrodesis, resection of the fibular head, soft tissue reconstruction, and temporary fixation.10,13,26,31 Given the rare nature of the injury and lack of data on varying treatments, there is no clear consensus on the optimal surgical procedure.
Arthrodesis and fibular head resection are 2 traditional methods of surgically addressing the PTFJ, but both have limitations that need to be recognized. Arthrodesis involves clearing the PTFJ of all articular cartilage, bone grafting, and then reducing the joint using screw fixation.11 Rigid fixation prevents rotation of the fibula which puts additional stress on the ankle, frequently causing pain and instability of the ankle joint.10,11 The other traditional surgical option, fibular head resection, involves excision of the head and neck of the fibula while preserving the fibular styloid and LCL.4 Fibular head resection is indicated when peroneal nerve symptoms or palsy occur in PTFJ injuries.4,10 Unfortunately, resection may disrupt the posterolateral corner structures of the knee and result in pain and instability.3,13 As a general rule, it is advisable to avoid arthrodesis and fibular head resection in children and athletes because of the length of time during which complications can develop.11
Van den Bekerom and colleagues26 suggest that their technique of temporary fixation of the PTFJ using a cancellous screw yields satisfactory outcomes in treating chronic PTFJ instability. The method entails having the ankle dorsiflexed and the head of the fibula slightly externally rotated and reduced into the most stable position. A hole is drilled in the anteromedial direction from posterior fibula head into the tibia. A non-tapped cortical screw is used to fix the fibula head in the reduced position.26 The screw is removed after 3 to 6 months. Seven of 8 patients treated with this technique by Van den Bekerom and colleagues26 have had alleviation of symptoms, although the screws broke in 2 cases before their planned removal.
Soft tissue reconstruction of the PTFJ has been an evolving area of treatment. Several techniques using a variety of tissue grafts and fixation methods have been described. Giachino32 proposed a reconstruction in which the fibular head is stabilized with a strip of ipsilateral biceps femoris tendon still attached distally to the fibular head and deep fascia of the leg. Drill holes are made in the tibia, and the tissue is secured anteriorly to the fascia with a suture. Shapiro and colleagues33 performed a stabilization using a strip of the iliotibial band still connected to its insertion on Gerdy’s tubercle. The graft was tunneled through a tibial drill hole from anterior to posterior and through the fibular head from posterior to anterior before suturing back onto itself. Another technique described by Mena and colleagues34 uses a split biceps tendon autograft, harnessing the strong attachments of the tendon to the fibular head to stabilize the reduced PTFJ.
More recently, Miller35 employed elements of the techniques described by Gianchino,32 Shapiro and colleagues,33 and Mena and colleagues34 to develop a method involving the biceps femoris tendon and iliotibial band in a soft tissue reconstruction. The technique involves harvesting the biceps femoris tendon while preserving the distal insertion and harvesting the iliotibial band while leaving the distal insertion at the Gerdy’s tubercle intact.35 The biceps tendon is passed from posterior to anterior through a tibial bone tunnel, and the iliotibial band graft is passed through the same tunnel but in the anterior to posterior direction. A bioabsorbable interference screw is placed into the tibial tunnel from anterior to posterior. The remaining biceps tendon graft is sewn into the soft tissue of the anterior tibia. The tail of the iliotibial band graft is passed through the fibular bone tunnel in the posterior to anterior direction and looped around the fibular head and under the LCL before being sewn back onto itself.35 Kobbe and colleagues36 described a surgical technique using the ipsilateral semitendinosis tendon while Maffulli and colleagues37 and Morrison and colleagues38 presented techniques using the ipsilateral gracilis tendon. These techniques do not jeopardize lateral knee or fibular head stability by avoiding the use of the biceps femoris and iliotibial band, as well as local knee stabilizers. Warner and colleagues31 proposed a similar technique to the aforementioned using a semitendinosus tendon; however, they do not reconstruct the anterior ligamentous structures, as seen in Figures 4A and 4B.39 The authors have concerns that a 2-limb reconstruction as described by Kobbe and colleagues36 and Morrison and colleagues38 may be prone to overconstraint or other errors in tensioning because it does not allow for differential tension to be applied to each limb.31 According to Warner and colleagues,31 the anterior structures of their patients with chronic PTFJ instability have appeared normal, and isolated posterior ligamentous reconstruction has been adequate for the restoration of stability. Camarda and colleagues28 proposed a technique very similar to that of Warner and colleagues;31 however, the tibial tunnel is reamed from posterolateral to the anteromedial aspect of the tibia, exploiting the skin incision previously used for tendon harvest.
Continue to: ARTHRITIS
ARTHRITIS
The management options for secondary arthritis due to chronic PTFJ instability have rarely been discussed in the literature. Arthrodesis or fibular head resection are options for the treatment of arthritis, and the above discussion applies here as well. Yaniv and colleagues40 describe a technical procedure for addressing both instability and secondary joint arthritis. The authors performed a ligament reconstruction of the PTFJ using the anterior part of the biceps femoris combined with interpositional joint arthroplasty using a vascularized fascia lata strip. Two weeks post-operation, the patient was walking with full weight-bearing and 6 weeks post-operation the first sports activities were allowed.40
CONCLUSION
Conditions affecting the PTFJ, their diagnosis, and treatment are infrequent topics of discussion in the literature. While PTFJ injury, instability, and other disease states are admittedly rare, clinicians need to include them in the differential diagnosis of patients presenting with lateral knee complaints. Diagnostic imaging is a critical component in early identification of PTFJ conditions to prevent long-term complications. Most injuries are treated first with conservative methods, reserving surgery as an option when first-line measures are unsuccessful. Advancements in surgical options for dislocation and subluxation/chronic instability of the joint have been made, but further research on their effectiveness and long-term outcomes is needed before a gold-standard treatment can be determined.
- Ogden JA. The anatomy and function of the proximal tibiofibular joint. Clin Orthop Relat Res. 1974(101):186-191.
- Resnick D, Newell JD, Guerra J, Jr., Danzig LA, Niwayama G, Goergen TG. Proximal tibiofibular joint: anatomic-pathologic-radiographic correlation. AJR Am J Rroentgenol. 1978;131(1):133-138. doi: 10.2214/ajr.131.1.133.
- See A, Bear RR, Owens BD. Anatomic mapping for surgical reconstruction of the proximal tibiofibular ligaments. Orthopedics. 2013;36(1):e58-63. doi: 10.3928/01477447-20121217-19.
- Ogden JA. Subluxation of the proximal tibiofibular joint. Clin Orthop Relat Res. 1974(101):192-197. doi: 10.2106/00004623-197456010-00015
- Bozkurt M, Yilmaz E, Akseki D, Havitcioglu H, Gunal I. The evaluation of the proximal tibiofibular joint for patients with lateral knee pain. Knee. 2004;11(4):307-312. doi: 10.1016/j.knee.2003.08.006
- Dirim B, Wangwinyuvirat M, Frank A, et al. Communication between the proximal tibiofibular joint and knee via the subpopliteal recess: MR arthrography with histologic correlation and stratigraphic dissection. AJR Am J Roentgenol. 2008;191(2):W44-W51. doi: 10.2214/AJR.07.3406.
- Eichenblat M, Nathan H. The proximal tibio fibular joint. An anatomical study with clinical and pathological considerations. Int Orthop. 1983;7(1):31-39. doi: 10.1007/bf00267557
- Espregueira-Mendes JD, da Silva MV. Anatomy of the proximal tibiofibular joint. Knee Surg Sports Traumatol Arthrosc. 2006;14(3):241-249. doi: 10.1007/s00167-005-0684-z.
- Scott J, Lee H, Barsoum W, van den Bogert AJ. The effect of tibiofemoral loading on proximal tibiofibular joint motion. J Anat. 2007;211(5):647-653. doi: 10.1111/j.1469-7580.2007.00803.x.
- Ogden JA. Subluxation and dislocation of the proximal tibiofibular joint. J Bone Joint Surg Am. 1974;56(1):145-154. doi: 10.2106/00004623-197456010-00015
- Sekiya JK, Kuhn JE. Instability of the proximal tibiofibular joint. J Am Acad Orthop Surg. 2003;11(2):120-128. doi: 10.5435/00124635-200303000-00006
- Horan J, Quin G. Proximal tibiofibular dislocation. Emerg Med Jl : EMJ. 2006;23(5):e33. doi: 10.1136/emj.2005.032144.
- Semonian RH, Denlinger PM, Duggan RJ. Proximal tibiofibular subluxation relationship to lateral knee pain: a review of proximal tibiofibular joint pathologies. J Orthop Sports Phys Ther. 1995;21(5):248-257. doi: 10.2519/jospt.1995.21.5.248.
- Veth RP, Kingma LM, Nielsen HK. The abnormal proximal tibiofibular joint. Arch Orthop Trauma Surg. 1984;102(3):167-171. doi: 10.1007/bf00575227
- Boya H, Ozcan O, Oztekin HH. Radiological evaluation of the proximal tibiofibular joint in knees with severe primary osteoarthritis. Knee Surg Sports Traumatol Arthrosc. 2008;16(2):157-159. doi: 10.1007/s00167-007-0442-5.
- Öztuna V, Yildiz A, Özer C, Milcan A, Kuyurtar F, Turgut A. Involvement of the proximal tibiofibular joint in osteoarthritis of the knee. The Knee. 2003;10(4):347-349. doi: 10.1016/s0968-0160(03)00004-8
- Milankov M, Kecojević V, Gvozdenović N, Obradović M. Dislocation of the proximal tibiofibular joint. Med Pregl. 2013;66(9-10):387-391. doi: 10.2298/mpns1310387m
- Sijbrandij S. Instability of the proximal tibio-fibular joint. Acta Orthop Scand. 1978;49(6):621-626. doi: 10.3109/17453677808993250
- Aladin A, Lam KS, Szypryt EP. The importance of early diagnosis in the management of proximal tibiofibular dislocation: a 9- and 5-year follow-up of a bilateral case. Knee. 2002;9(3):233-236. doi: 10.1016/S0968-0160(02)00012-1
- Turco VJ, Spinella AJ. Anterolateral dislocation of the head of the fibula in sports. Am J Sports Med. 1985;13(4):209-215. doi: 10.1177/036354658501300401.
- Hey HW, Ng LW, Ng YH, Sng WZ, Manohara R, Thambiah JS. Radiographical definition of the proximal tibiofibular joint - A cross-sectional study of 2984 knees and literature review. Injury. 2016;47(6):1276-1281. doi: 10.1016/j.injury.2016.01.035.
- Voglino JA, Denton JR. Acute traumatic proximal tibiofibular joint dislocation confirmed by computed tomography. Orthopedics. 1999;22(2):255-258.
- Burke CJ, Grimm LJ, Boyle MJ, Moorman CT, 3rd, Hash TW, 2nd. Imaging of Proximal Tibiofibular Joint Instability: A 10 year retrospective case series. Clin Imaging. 2016;40(3):470-476. doi: 10.1016/j.clinimag.2015.12.011.
- Parkes JC II, Zelko RR. Isolated acute dislocation of the proximal tibiofibular joint. Case report. J Bone Joint Surg Am. 1973;55(1):177-183. Doi: 10.2106/00004623-197355010-00019
- Gvozdenović N, Gvozdenović K, Obradović M, Stanković M. Modified technique of the treatment for proximal tibiofibular joint dislocation. Vojnosanitetski Pregled. 2017;74(3):282-286. doi: 10.2298/VSP150318177G
- van den Bekerom MP, Weir A, van der Flier RE. Surgical stabilisation of the proximal tibiofibular joint using temporary fixation: a technical note. Acta Orthop Belg. 2004;70(6):604-608.
- Calabró T, Cevolani L, Chehrassan M, Gasbarrini A. A new technique of reduction for isolated proximal tibiofibular joint dislocation: a case report. Eur Rev Med Pharmacol Sci. 2014;18(1):93-95.
- Camarda L, Abruzzese A, D'Arienzo M. Proximal tibiofibular joint reconstruction with autogenous semitendinosus tendon graft. Tech Orthop. 2013;28(3):269-272. doi. 10.1097/BTO.0b013e31827b7182
- Herzog GA, Serrano-Riera R, Sagi HC. Traumatic Proximal Tibiofibular Dislocation: A Marker of Severely Traumatized Extremities. J Orthop Trauma. 2015;29(10):456-459. doi: 10.1097/BOT.0000000000000348.
- Haupt S, Frima H, Sommer C. Proximal tibiofibular joint dislocation associated with tibial shaft fractures - 7 cases. Injury. 2016;47(4):950-953. doi: 10.1016/j.injury.2016.01.037.
- Warner BT, Moulton SG, Cram TR, LaPrade RF. Anatomic Reconstruction of the Proximal Tibiofibular Joint. Arthrosc Tech. 2016;5(1):e207-e210. doi: 10.1016/j.eats.2015.11.004
- Giachino AA. Recurrent dislocations of the proximal tibiofibular joint. Report of two cases. J Bone Joint Surg Am. 1986;68(7):1104-1106. doi: 10.2106/00004623-198668070-00023
- Shapiro GS, Fanton GS, Dillingham MF. Reconstruction for recurrent dislocation of the proximal tibiofibular joint. A new technique. Orthop Rev. 1993;22(11):1229-1232.
- Mena H, Brautigan B, Johnson DL. Split biceps femoris tendon reconstruction for proximal tibiofibular joint instability. Arthroscopy. 2001;17(6):668-671. doi: 10.1053/jars.2001.22359.
- Miller T. New technique of soft tissue reconstruction for proximal tibiofibular joint instability using iliotibial band and biceps femoris longhead autograft. Tech Orthop. 2014;29(4):243-247. doi: 10.1097/BTO.0000000000000046
- Kobbe P, Flohe S, Wellmann M, Russe K. Stabilization of chronic proximal tibiofibular joint instability with a semitendinosus graft. Acta Orthop Belg. 2010;76(6):830-833.
- Maffulli N, Spiezia F, Oliva F, Testa V, Capasso G, Denaro V. Gracilis autograft for recurrent posttraumatic instability of the superior tibiofibular joint. Am J Sports Med. 2010;38(11):2294-2298. doi: 10.1177/0363546510373472.
- Morrison TD, Shaer JA, Little JE. Bilateral, atraumatic, proximal tibiofibular joint instability. Orthopedics. 2011;34(2):133. doi: 10.3928/01477447-20101221-28.
- Horst PK, LaPrade RF. Anatomic reconstruction of chronic symptomatic anterolateral proximal tibiofibular joint instability. Knee Surg Sports Traumatol Arthrosc. 2010;18(11):1452-1455. doi: 10.1007/s00167-010-1049-9.
- Yaniv M, Koenig U, Imhoff AB. A technical solution for secondary arthritis due to chronic proximal tibiofibular joint instability. Knee Surg Sports Traumatol Arthrosc. 1999;7(5):334-336. doi: 10.1007/s001670050173.
ABSTRACT
Current literature is limited with respect to the proximal tibiofibular joint (PTFJ) and clinical conditions relating to the PTFJ. Diagnosis and treatment of conditions that affect the PTFJ are not well described and are a topic of debate among many physicians. This manuscript aims to review and summarize the most recent literature that relates to traumatic dislocations, fractures, chronic instability, and osteoarthritis, with a focus on both diagnostic and treatment strategies of these conditions. We also review PTFJ anatomy, biomechanics, and the clinical presentation of some common PTFJ conditions.
Continue to: Clinical conditions...
Clinical conditions of the proximal tibiofibular joint (PTFJ) are an uncommon source of lateral knee complaints and are often overlooked in the differential diagnosis as a source of the knee complaint. The most common conditions of the PTFJ include traumatic dislocations, fractures, chronic instability, and osteoarthritis. This article reviews the most common diseases affecting this joint and discusses both diagnostic and treatment strategies in an attempt to raise awareness of this joint as a source of lateral knee complaints.
ANATOMY
The PTFJ is an arthrodial synovial joint between the posterolateral surface of the tibia and the proximal fibular head.1 Surrounding the synovial membrane of the articulation is a fibrous joint capsule with distinct anterior and posterior tibiofibular ligaments.2,3 The anterior tibiofibular ligament has been described as 1 or 2 bands whereas the posterior ligament consists of 1 band.3 The anterior ligament attaches anteroinferiorly to the fibular styloid and posteriorly to Gerdy’s tubercle on the tibia. It runs linearly from posterior to anterior, and the fibular footprint is immediately anterior to the insertion of the biceps femoris. The posterior ligament is located inferior to the lateral joint space, and the fibular footprint is posterior to the insertion of the biceps femoris.3 Anatomy of the PTFJ is shown schematically in Figure 1.
Both the lateral collateral ligament (LCL) and the tibiofibular interosseous membrane add stability to the PTFJ. The LCL travels from the lateral femoral epicondyle to the lateral side of fibula head, anterior to the fibular styloid. The interosseous membrane extends obliquely between the borders of the tibia and fibula. Additionally, the short head of the biceps femoris, fibular collateral ligament, fabellofibular ligament, popliteofibular ligament, and popliteus muscle all attach to the PTFJ and provide additional stability to the joint.
It is important to note that the common peroneal nerve passes posteriorly over the fibula neck, can be involved in the clinical presentation, and is a potential source of concern with any injury to or surgery on the joint.4
Many studies have demonstrated that a communication with the tibiofemoral joint exists through the subpopliteal recess, but the rate of communication has varied widely.5-8 Most recently, Bozkurt and colleagues5 found the rate of communication between the PTFJ and lateral femorotibial space to be 57.9%. When distinct communication exists, the PTFJ must be considered as a fourth compartment of the knee and is subject to any process that affects the knee joint proper.
Continue to: Ogden described 2 types...
Ogden1 described 2 types of PTFJs, horizontal and oblique, with the latter being considered less stable because of less rotational mobility. The horizontal configuration is defined as <20° of inclination of joint surface in relation to the horizontal plane, and the oblique variation is defined as >20° of inclination of the joint surface in relation to the horizontal plane.1
BIOMECHANICS AND FUNCTION
The primary function of the PTFJ is to dissipate torsional loads applied to the ankle, attenuate lateral tibial bending moments, and transmit axial loads from weight bearing on the extremity.1 The degree of knee flexion, ankle dorsiflexion, and tibial rotation all play an important role in PTFJ biomechanics. In knee flexion, the proximal fibula moves anteriorly because of the relative laxity of the LCL and biceps femoris tendons. In knee extension, the LCL and biceps femoris tighten, pulling the proximal fibula posteriorly.1 Because the LCL and biceps tendon are both relaxed and less supportive during knee flexion, the PTFJ is more prone to injury with a flexed knee. The ankle plays an important role in the biomechanics of the PTFJ because it contains the distal syndesmosis, where both the tibia and fibula are firmly attached distally. During ankle dorsiflexion, the fibula must externally rotate to accommodate a wider anterior talus.1 In regard to tibia rotation, Scott and colleagues9 demonstrated the relationship between tibial rotation and fibular translation. With internal tibial rotation, the fibular head translated posteriorly and with external tibial rotation, the fibula translated anteriorly. The greatest translational motion was seen during loading of the knee into varus and during external tibial rotation at all flexion angles.
CLINICAL CONDITIONS
Ogden10 classified instability of the PTFJ into 4 main groups: anterolateral dislocation, posteromedial dislocation, superior dislocation, and atraumatic subluxation. Injury to the PTFJ usually occurs in younger, athletic patients during sports that require violent twisting motions such as soccer, basketball, dance, skiing, horseback riding, parachute jumping, jet skiing, and judo. Patients with generalized ligamentous laxity have been described as at increased risk for joint instability.10,11
ACUTE DISLOCATION
The most common injury to the PTFJ is an anterolateral dislocation and involves injury to both the anterior and posterior capsular ligaments, and occasionally the LCL.10 Anterolateral dislocation is usually the result of a fall on a hyperflexed knee with the foot inverted and plantarflexed.11 While most anterolateral dislocations are the result of indirect sports trauma, several have been associated with other types of skeletal injuries such as fracture-dislocation of the hip, crush injury of the proximal and distal ends of the tibia, fracture-dislocation of the ankle, proximal tibial fracture, and fracture-dislocation of the distal femoral epiphysis.10 Ogden10 described the mechanism as follows: (1) sudden inversion and plantar flexion of the foot causing tension in the peroneal muscle group, extensor digitorum longus, and extensor halluces longus, which applies a forward dislocating force to the proximal end of the fibula; (2) simultaneous flexion of the knee, relaxing the biceps tendon and LCL; and (3) twisting of the body over the knee, transmitting energy along the femur to the tibia, exerting a relative external rotatory torque of the tibia on the foot, which is already fixed in inversion. Steps (2) and (3) spring the proximal end of the fibula laterally while the contracting muscles of (1) pull the fibula anteriorly.
Posteromedial dislocation is the second most common type of acute PTFJ dislocation. Posteromedial dislocations usually involve direct trauma and are associated with peroneal nerve injuries.1,2,10 The mechanism of dislocation results in tearing of the anterior and posterior PTFJ capsular ligaments, followed by injury to the LCL and other surrounding ligaments. This allows the biceps femoris to draw the unsupported proximal part of the fibula posteromedially along the posterolateral tibial metaphysis.7
Continue to: Superior dislocations are...
Superior dislocations are the least frequent form of acute PTFJ dislocations and are associated with high-energy ankle injuries.2,10,12 Superior dislocation results in injury to the interosseous membrane between the tibia and fibula and is frequently associated with tibial shaft fracture.10,11 Atraumatic, acquired superior dislocation of the PTFJ has also been associated with congenital dislocation of the knee.10,11
SUBLUXATION/CHRONIC INSTABILITY
Subluxation of the PTFJ classically involves excessive and symptomatic anterior-posterior motion without actual dislocation of the joint.11 Subluxations of the PTFJ typically occur without any known trauma or injury and are most frequently associated with benign hyperlaxity syndrome, Ehlers-Danlos syndrome, or muscular dystrophy.4,10
Semonian and colleagues13 suggest that subluxation of the PTFJ is not given enough recognition in the literature and that instability should not be considered a rare condition. They hypothesize that many patients have joints that do not tolerate increases in fibula rotation secondary to subclinical trauma, repetitive overuse, or biomechanical variation of the joint. Semonian and associates13 state that the condition begins with the anterior capsule and anterior tibiofibular ligament attenuation as a result of excessive fibular rotation. Once stretched, the functional pull of the biceps femoris and soleus maintain the fibula in a relatively posterior and externally rotated position. Furthermore, Ogden10 found that 70% of the dislocations and subluxations he studied were of the oblique variant compared with that of the horizontal variant. Many authors suggest that the oblique variant is more at risk for injury because of its decreased joint surface area causing decreased rotational mobility.1,2,10
Early recognition is extremely important in dislocations and subluxations of the PTFJ as undiagnosed acute trauma can turn into chronic subluxation, and chronic subluxation may lead to dislocation.13 Additionally, chronic subluxation or dislocation are thought result in osteoarthritis of the PTFJ.14
OSTEOARTHRITIS
The literature on osteoarthritis of the PTFJ is limited. Eichenblat and Nathan7 studied the PTFJ in cadavers and dry bones and found that 28% had evidence of osteoarthritis. Clinically, however, osteoarthritis of the PTFJ is a rare primary diagnosis, suggesting that involvement of the PTFJ is either asymptomatic or that symptoms are associated with osteoarthritis of the knee joint. Boya and colleagues15 and Eichenblat and Nathan7 both found a high correlation between the presence of osteoarthritis of the PTFJ and osteoarthritis of the tibiofemoral joint in cadavers. The authors suggest this correlation may be related to the presence of anatomical communication between the 2 joints. Theoretically, inflammatory mediators flow freely between the joint spaces and contribute to arthritis in both joints. The possibility of degenerative arthritis of the PTFJ accompanying degenerative arthritis of the knee warrants evaluation, especially in patients considering total knee arthroplasty. Unrecognized arthritis of the PTFJ might influence outcome scores and be an unsolved source of lateral knee pain post-knee replacement.16
Continue to: CLINICAL PRESENTATION
CLINCIAL PRESENTATION
ACUTE DISLOCATION
Patients with acute PTFJ dislocation present with pain, tenderness, swelling, and asymmetry of the lateral side of the knee, while the knee joint is not swollen and range of knee motion is not limited.17 A bony prominence might be felt, and the biceps femoris tendon can often appear to be tense.13 Active or passive ankle movements often exacerbate the lateral knee pain.11 It is also important to examine the peroneal nerve, as transient peroneal palsy has been described in all types of PTFJ dislocations but most often with posteromedial dislocations. Sensory disturbance in the peroneal nerve distribution is more common than motor loss, but foot drop is also a potential presenting sign.11 On examination, palpation of the fibular head illustrates tenderness and aggravates the pain.
SUBLUXATION/CHRONIC INSTABILITY
Subluxation of the PTFJ can be difficult to recognize because the history, signs, and symptoms of lateral knee pain can be subtle and sometimes misleading. In addition, current literature provides little information on specific tests, measurements, signs, or subjective information regarding subluxation. Patients rarely reveal a history of trauma or mechanism of injury. Subluxations are often associated with patients participating in repetitive sports requiring running, jumping, or twisting movements, and can be present bilateral. Instability has also been described in patients with osteomyelitis, rheumatoid arthritis, septic arthritis, pigmented villonodular synovitis, below-knee amputations, osteochondroma, and in runners who recently increased mileage (especially during the first 2-3 miles and during downhill running).11,13 Patients normally do not have difficulty with activities of daily living, but symptoms may arise when making movements with a sudden change of direction.11
These patients usually complain of instability of the knee and pain along the lateral aspect of the knee. Pain radiating proximally into the region of the iliotibial band and medially into the patellofemoral joint can be seen.13 Patients may also report clicking, popping, or catching of the lateral knee; while others will report a sense of giving way of the knee joint.11,13 Progressive peroneal nerve symptoms are usually seen in older patients; however, they are more common with acute PTFJ dislocations as discussed.13
A clinical method for examining a PTFJ with possible subluxation or chronic instability has been described by Sijbrandij.18 With the patient in the supine position, the knee is flexed to 90° to relax the LCL and biceps femoris tendon. The fibular head is then held between the thumb and index finger, and moved anteriorly and laterally. Dislocation or subluxation will be felt and visualized as the fibular head translates, and should be compared with the uninjured PTFJ. On release, the fibular head will return to its normal position, often with a click. Asking the patient if this subluxation/reduction maneuver reproduces the symptoms or causes apprehension or pain may also be helpful.18 Another method for examination is eliciting the Radulescu sign.11,13 While the patient lies prone, the examiner stabilizes the thigh with 1 hand while the knee is flexed to 90°. The examiner then applies an internal rotation force on the lower leg. Observing an abnormal excursion of the fibular head in an anterior and lateral direction represents a positive test.11,13
OSTEOARTHRITIS
Clinical evaluation for osteoarthritis in the PTFJ is not well described in the literature. A single report describes applying manual pressure over the fibular head during active ankle motion.16 A test known as the grinding test is used as a sign to detect the involvement of the PTFJ as a component of osteoarthritis of the knee. A positive test will elicit pain and/or tenderness of the joint.16
Continue to: DIAGNOSTIC IMAGING
DIAGNOSTIC IMAGING
Plain radiographs in the anteroposterior (AP) and true lateral views are useful as first-line investigations in suspected PTFJ dislocation. Comparable AP and lateral radiographs of each knee are highly recommended to detect findings that suggest dislocation.2 Abnormal findings include increased interosseous space, medial or lateral displacement of the fibula on the anteroposterior view, and anterior or posterior displacement of the fibula head on lateral view as shown in Figure 2.19,20
Resnick and colleagues2 proposed the use of the linear sloping radiodensity that defines the posteromedial corner of the lateral tibial condyle as an indicator of anterolateral or posteromedial PTFJ dislocation. However, this application is limited because of the PTFJ’s highly variable morphology.8 In a recent study conducted by Hey and colleagues,21 5968 (2984 patients) knee radiographs were retrospectively collected and subjected to radiographical measurements and statistical analysis. The tibiofibular overlap method had a specificity of 94.1% and 84.5% when diagnosing PTFJ dislocations on the AP and lateral views, respectively.21
If a diagnosis of PTFJ is suspected but not clearly established based on radiography, computed tomography with comparison views of the contralateral knee are recommended to confirm the diagnosis.17,22 This becomes more critical in cases of suspected subluxation/chronic PTFJ instability. Additionally, magnetic resonance imaging (MRI) can be used to assess chronic PTFJ instability. Recently, Burke and colleagues23 performed a 10-year retrospective case series that included 7 patients with chronic PTFJ instability and included MRI as part of their evaluation. The MRI abnormalities in these patients included periarticular soft tissue edema, including in the proximal soleus muscle (n = 5), periarticular ganglion or ganglia (n = 4), tibiofibular ligament edema (n = 4), subchondral marrow edema (n = 3), posterior tibiofibular ligament thickening (n = 2), subcortical cyst at a ligament insertion (n = 2), partial-thickness tear of the anterior tibiofibular ligament (n = 1), and tibiofibular joint effusion (n = 1).
OSTEOARTHRITIS
Routine knee radiographs can show PTFJ joint space narrowing, sclerosis, marginal osteophytes, and local osteopenia as conventional components of osteoarthritis of any joint. Serial radiographs have also been described as effective in evaluating progressive degenerative changes of the PTFJ.14 An MRI will show osteophyte formation, subchondral cysts, subchondral sclerosis, joint effusion, joint space narrowing, and is highly sensitive for detecting degenerative changes in cartilage, as well as identifying other possible pathologies such as synovial cysts or pigmented villonodular synovitis. Chronic PTFJ instability appears to predispose to tibiofibular osteoarthritis as reported by Burke and colleagues,23 who found a particularly high incidence (42.9%) of osteoarthritis in patients with chronic PTFJ instability. Additionally, Veth and colleagues14 found degenerative changes in 8 of 19 patients presenting with PTFJ dislocations.
TREATMENT
ACUTE DISLOCATION
Prompt recognition and treatment of any acute PTFJ dislocation are necessary to avoid long-term instability and other possible sequelae.11 Treatment consists of reduction followed by restriction of weight-bearing.11 Traditionally, the knee is immobilized with a cast in extension for 3 to 4 weeks followed by knee mobilization and progressive range of motion exercises,24 but there is some controversy regarding complete immobilization.11,25,26
Continue to: Initially, closed reduction...
Initially, closed reduction is advised as the treatment for acute PTFJ dislocation.11,19,24 It involves placing the knee in 80°to 110° of flexion to relax the biceps femoris and LCL, then applying an appropriate force to the fibular head in a direction opposite the displacement.11,24 An audible pop is often heard as the fibula reduces back into normal alignment. Stability of the reduction and stability of the knee should be determined with respect to both posterolateral structures and the LCL after reduction.11 Anterolateral dislocations are usually easier to reduce, as a posteromedial or superior dislocation can result in the fibular head being perched on the lateral tibial ridge, and held by the LCL.11
Calabró and colleagues27 described a new, simple, and safe alternative technique of closed reduction of an anterior dislocation if the classical method fails. This technique relies on ligamentotaxis and a dynamic counteraction between muscles and ligaments to reduce the joint. The patient flexes the knee >90° while the physician applies a counterforce to the heel with the palm. Simultaneously, gentle direct pressure should be applied to the fibular head to move it toward the lateral tibial ridge. With a relaxed LCL, the biceps femoris tendon will actively reduce the proximal fibular head back into its correct anatomic orientation.27
When a closed reduction in an awake patient has failed, a reduction under sedation or anesthesia should be performed. If that fails, an open reduction should be performed. Following an open reduction, the joint is stabilized with Kirschner wires, bioabsorbable pins, or cortical screws.24-26,28 The torn capsule and any injured ligaments should also be primarily repaired. After approximately 6 weeks, the stabilization hardware may be removed.24,25
Acute posteromedial dislocations are treated similarly to anterolateral dislocations; however, open reduction and repair of the capsule and ligaments are more frequently required.10 Superior dislocations are also more frequently reduced by open methods, sometimes dictated by open treatment of associated tibia or ankle fracture.10 Damage to any structure of the posterolateral knee as a result of acute PTFJ injury should be repaired, as this has been associated with better outcomes.14
PTFJ dislocation and tibia fracture can occur together, and in a retrospective study conducted by Herzog and colleagues29 the authors recorded the incidence of PTFJ dislocation as 1.5% of operative tibial shaft fractures and 1.9% of operative tibial plateau fractures. Haupt and colleagues30 also conducted a retrospective study in which the authors recorded the incidence of PTFJ dislocations in 1.06% of all tibial shaft fractures in their series. In both studies combined, all except 1 PTFJ dislocation had been caused by high-energy trauma.29,30 In the case of PTFJ dislocation with tibial shaft fracture, intramedullary nailing of the tibial shaft fracture followed by open reduction of the PTFJ with 1 or 2 positioning screws just below the PTFJ has yielded satisfactory results.30 The positioning screw should be removed 6 weeks post-operation to prevent PTFJ arthrodesis and patients should be supported in full weight-bearing.30 An illustrative case report from our institution is shown in Figure 3.
Continue to: CHRONIC INSTABILITY
CHRONIC INSTABILITY
Chronic instability is commonly the result of untreated or misdiagnosed subluxation of the PTFJ. Ogden10 reported that 57% of patients with acute proximal tibiofibular dislocations required surgery for ongoing symptoms after treatment failure with closed reduction and 3 weeks of immobilization. The first step in the management of chronic instability of the PTFJ is usually a nonoperative approach. Immobilization, activity level modification, utilization of a supportive strap placed 1 centimeter below the fibular head for pain relief, and participation in a strength-training program of the lower leg are initial treatment recommendations.10,11,13 Many patients with chronic PTFJ instability do not respond to conservative treatment and may pursue surgical intervention. Surgical treatment options include permanent arthrodesis, resection of the fibular head, soft tissue reconstruction, and temporary fixation.10,13,26,31 Given the rare nature of the injury and lack of data on varying treatments, there is no clear consensus on the optimal surgical procedure.
Arthrodesis and fibular head resection are 2 traditional methods of surgically addressing the PTFJ, but both have limitations that need to be recognized. Arthrodesis involves clearing the PTFJ of all articular cartilage, bone grafting, and then reducing the joint using screw fixation.11 Rigid fixation prevents rotation of the fibula which puts additional stress on the ankle, frequently causing pain and instability of the ankle joint.10,11 The other traditional surgical option, fibular head resection, involves excision of the head and neck of the fibula while preserving the fibular styloid and LCL.4 Fibular head resection is indicated when peroneal nerve symptoms or palsy occur in PTFJ injuries.4,10 Unfortunately, resection may disrupt the posterolateral corner structures of the knee and result in pain and instability.3,13 As a general rule, it is advisable to avoid arthrodesis and fibular head resection in children and athletes because of the length of time during which complications can develop.11
Van den Bekerom and colleagues26 suggest that their technique of temporary fixation of the PTFJ using a cancellous screw yields satisfactory outcomes in treating chronic PTFJ instability. The method entails having the ankle dorsiflexed and the head of the fibula slightly externally rotated and reduced into the most stable position. A hole is drilled in the anteromedial direction from posterior fibula head into the tibia. A non-tapped cortical screw is used to fix the fibula head in the reduced position.26 The screw is removed after 3 to 6 months. Seven of 8 patients treated with this technique by Van den Bekerom and colleagues26 have had alleviation of symptoms, although the screws broke in 2 cases before their planned removal.
Soft tissue reconstruction of the PTFJ has been an evolving area of treatment. Several techniques using a variety of tissue grafts and fixation methods have been described. Giachino32 proposed a reconstruction in which the fibular head is stabilized with a strip of ipsilateral biceps femoris tendon still attached distally to the fibular head and deep fascia of the leg. Drill holes are made in the tibia, and the tissue is secured anteriorly to the fascia with a suture. Shapiro and colleagues33 performed a stabilization using a strip of the iliotibial band still connected to its insertion on Gerdy’s tubercle. The graft was tunneled through a tibial drill hole from anterior to posterior and through the fibular head from posterior to anterior before suturing back onto itself. Another technique described by Mena and colleagues34 uses a split biceps tendon autograft, harnessing the strong attachments of the tendon to the fibular head to stabilize the reduced PTFJ.
More recently, Miller35 employed elements of the techniques described by Gianchino,32 Shapiro and colleagues,33 and Mena and colleagues34 to develop a method involving the biceps femoris tendon and iliotibial band in a soft tissue reconstruction. The technique involves harvesting the biceps femoris tendon while preserving the distal insertion and harvesting the iliotibial band while leaving the distal insertion at the Gerdy’s tubercle intact.35 The biceps tendon is passed from posterior to anterior through a tibial bone tunnel, and the iliotibial band graft is passed through the same tunnel but in the anterior to posterior direction. A bioabsorbable interference screw is placed into the tibial tunnel from anterior to posterior. The remaining biceps tendon graft is sewn into the soft tissue of the anterior tibia. The tail of the iliotibial band graft is passed through the fibular bone tunnel in the posterior to anterior direction and looped around the fibular head and under the LCL before being sewn back onto itself.35 Kobbe and colleagues36 described a surgical technique using the ipsilateral semitendinosis tendon while Maffulli and colleagues37 and Morrison and colleagues38 presented techniques using the ipsilateral gracilis tendon. These techniques do not jeopardize lateral knee or fibular head stability by avoiding the use of the biceps femoris and iliotibial band, as well as local knee stabilizers. Warner and colleagues31 proposed a similar technique to the aforementioned using a semitendinosus tendon; however, they do not reconstruct the anterior ligamentous structures, as seen in Figures 4A and 4B.39 The authors have concerns that a 2-limb reconstruction as described by Kobbe and colleagues36 and Morrison and colleagues38 may be prone to overconstraint or other errors in tensioning because it does not allow for differential tension to be applied to each limb.31 According to Warner and colleagues,31 the anterior structures of their patients with chronic PTFJ instability have appeared normal, and isolated posterior ligamentous reconstruction has been adequate for the restoration of stability. Camarda and colleagues28 proposed a technique very similar to that of Warner and colleagues;31 however, the tibial tunnel is reamed from posterolateral to the anteromedial aspect of the tibia, exploiting the skin incision previously used for tendon harvest.
Continue to: ARTHRITIS
ARTHRITIS
The management options for secondary arthritis due to chronic PTFJ instability have rarely been discussed in the literature. Arthrodesis or fibular head resection are options for the treatment of arthritis, and the above discussion applies here as well. Yaniv and colleagues40 describe a technical procedure for addressing both instability and secondary joint arthritis. The authors performed a ligament reconstruction of the PTFJ using the anterior part of the biceps femoris combined with interpositional joint arthroplasty using a vascularized fascia lata strip. Two weeks post-operation, the patient was walking with full weight-bearing and 6 weeks post-operation the first sports activities were allowed.40
CONCLUSION
Conditions affecting the PTFJ, their diagnosis, and treatment are infrequent topics of discussion in the literature. While PTFJ injury, instability, and other disease states are admittedly rare, clinicians need to include them in the differential diagnosis of patients presenting with lateral knee complaints. Diagnostic imaging is a critical component in early identification of PTFJ conditions to prevent long-term complications. Most injuries are treated first with conservative methods, reserving surgery as an option when first-line measures are unsuccessful. Advancements in surgical options for dislocation and subluxation/chronic instability of the joint have been made, but further research on their effectiveness and long-term outcomes is needed before a gold-standard treatment can be determined.
ABSTRACT
Current literature is limited with respect to the proximal tibiofibular joint (PTFJ) and clinical conditions relating to the PTFJ. Diagnosis and treatment of conditions that affect the PTFJ are not well described and are a topic of debate among many physicians. This manuscript aims to review and summarize the most recent literature that relates to traumatic dislocations, fractures, chronic instability, and osteoarthritis, with a focus on both diagnostic and treatment strategies of these conditions. We also review PTFJ anatomy, biomechanics, and the clinical presentation of some common PTFJ conditions.
Continue to: Clinical conditions...
Clinical conditions of the proximal tibiofibular joint (PTFJ) are an uncommon source of lateral knee complaints and are often overlooked in the differential diagnosis as a source of the knee complaint. The most common conditions of the PTFJ include traumatic dislocations, fractures, chronic instability, and osteoarthritis. This article reviews the most common diseases affecting this joint and discusses both diagnostic and treatment strategies in an attempt to raise awareness of this joint as a source of lateral knee complaints.
ANATOMY
The PTFJ is an arthrodial synovial joint between the posterolateral surface of the tibia and the proximal fibular head.1 Surrounding the synovial membrane of the articulation is a fibrous joint capsule with distinct anterior and posterior tibiofibular ligaments.2,3 The anterior tibiofibular ligament has been described as 1 or 2 bands whereas the posterior ligament consists of 1 band.3 The anterior ligament attaches anteroinferiorly to the fibular styloid and posteriorly to Gerdy’s tubercle on the tibia. It runs linearly from posterior to anterior, and the fibular footprint is immediately anterior to the insertion of the biceps femoris. The posterior ligament is located inferior to the lateral joint space, and the fibular footprint is posterior to the insertion of the biceps femoris.3 Anatomy of the PTFJ is shown schematically in Figure 1.
Both the lateral collateral ligament (LCL) and the tibiofibular interosseous membrane add stability to the PTFJ. The LCL travels from the lateral femoral epicondyle to the lateral side of fibula head, anterior to the fibular styloid. The interosseous membrane extends obliquely between the borders of the tibia and fibula. Additionally, the short head of the biceps femoris, fibular collateral ligament, fabellofibular ligament, popliteofibular ligament, and popliteus muscle all attach to the PTFJ and provide additional stability to the joint.
It is important to note that the common peroneal nerve passes posteriorly over the fibula neck, can be involved in the clinical presentation, and is a potential source of concern with any injury to or surgery on the joint.4
Many studies have demonstrated that a communication with the tibiofemoral joint exists through the subpopliteal recess, but the rate of communication has varied widely.5-8 Most recently, Bozkurt and colleagues5 found the rate of communication between the PTFJ and lateral femorotibial space to be 57.9%. When distinct communication exists, the PTFJ must be considered as a fourth compartment of the knee and is subject to any process that affects the knee joint proper.
Continue to: Ogden described 2 types...
Ogden1 described 2 types of PTFJs, horizontal and oblique, with the latter being considered less stable because of less rotational mobility. The horizontal configuration is defined as <20° of inclination of joint surface in relation to the horizontal plane, and the oblique variation is defined as >20° of inclination of the joint surface in relation to the horizontal plane.1
BIOMECHANICS AND FUNCTION
The primary function of the PTFJ is to dissipate torsional loads applied to the ankle, attenuate lateral tibial bending moments, and transmit axial loads from weight bearing on the extremity.1 The degree of knee flexion, ankle dorsiflexion, and tibial rotation all play an important role in PTFJ biomechanics. In knee flexion, the proximal fibula moves anteriorly because of the relative laxity of the LCL and biceps femoris tendons. In knee extension, the LCL and biceps femoris tighten, pulling the proximal fibula posteriorly.1 Because the LCL and biceps tendon are both relaxed and less supportive during knee flexion, the PTFJ is more prone to injury with a flexed knee. The ankle plays an important role in the biomechanics of the PTFJ because it contains the distal syndesmosis, where both the tibia and fibula are firmly attached distally. During ankle dorsiflexion, the fibula must externally rotate to accommodate a wider anterior talus.1 In regard to tibia rotation, Scott and colleagues9 demonstrated the relationship between tibial rotation and fibular translation. With internal tibial rotation, the fibular head translated posteriorly and with external tibial rotation, the fibula translated anteriorly. The greatest translational motion was seen during loading of the knee into varus and during external tibial rotation at all flexion angles.
CLINICAL CONDITIONS
Ogden10 classified instability of the PTFJ into 4 main groups: anterolateral dislocation, posteromedial dislocation, superior dislocation, and atraumatic subluxation. Injury to the PTFJ usually occurs in younger, athletic patients during sports that require violent twisting motions such as soccer, basketball, dance, skiing, horseback riding, parachute jumping, jet skiing, and judo. Patients with generalized ligamentous laxity have been described as at increased risk for joint instability.10,11
ACUTE DISLOCATION
The most common injury to the PTFJ is an anterolateral dislocation and involves injury to both the anterior and posterior capsular ligaments, and occasionally the LCL.10 Anterolateral dislocation is usually the result of a fall on a hyperflexed knee with the foot inverted and plantarflexed.11 While most anterolateral dislocations are the result of indirect sports trauma, several have been associated with other types of skeletal injuries such as fracture-dislocation of the hip, crush injury of the proximal and distal ends of the tibia, fracture-dislocation of the ankle, proximal tibial fracture, and fracture-dislocation of the distal femoral epiphysis.10 Ogden10 described the mechanism as follows: (1) sudden inversion and plantar flexion of the foot causing tension in the peroneal muscle group, extensor digitorum longus, and extensor halluces longus, which applies a forward dislocating force to the proximal end of the fibula; (2) simultaneous flexion of the knee, relaxing the biceps tendon and LCL; and (3) twisting of the body over the knee, transmitting energy along the femur to the tibia, exerting a relative external rotatory torque of the tibia on the foot, which is already fixed in inversion. Steps (2) and (3) spring the proximal end of the fibula laterally while the contracting muscles of (1) pull the fibula anteriorly.
Posteromedial dislocation is the second most common type of acute PTFJ dislocation. Posteromedial dislocations usually involve direct trauma and are associated with peroneal nerve injuries.1,2,10 The mechanism of dislocation results in tearing of the anterior and posterior PTFJ capsular ligaments, followed by injury to the LCL and other surrounding ligaments. This allows the biceps femoris to draw the unsupported proximal part of the fibula posteromedially along the posterolateral tibial metaphysis.7
Continue to: Superior dislocations are...
Superior dislocations are the least frequent form of acute PTFJ dislocations and are associated with high-energy ankle injuries.2,10,12 Superior dislocation results in injury to the interosseous membrane between the tibia and fibula and is frequently associated with tibial shaft fracture.10,11 Atraumatic, acquired superior dislocation of the PTFJ has also been associated with congenital dislocation of the knee.10,11
SUBLUXATION/CHRONIC INSTABILITY
Subluxation of the PTFJ classically involves excessive and symptomatic anterior-posterior motion without actual dislocation of the joint.11 Subluxations of the PTFJ typically occur without any known trauma or injury and are most frequently associated with benign hyperlaxity syndrome, Ehlers-Danlos syndrome, or muscular dystrophy.4,10
Semonian and colleagues13 suggest that subluxation of the PTFJ is not given enough recognition in the literature and that instability should not be considered a rare condition. They hypothesize that many patients have joints that do not tolerate increases in fibula rotation secondary to subclinical trauma, repetitive overuse, or biomechanical variation of the joint. Semonian and associates13 state that the condition begins with the anterior capsule and anterior tibiofibular ligament attenuation as a result of excessive fibular rotation. Once stretched, the functional pull of the biceps femoris and soleus maintain the fibula in a relatively posterior and externally rotated position. Furthermore, Ogden10 found that 70% of the dislocations and subluxations he studied were of the oblique variant compared with that of the horizontal variant. Many authors suggest that the oblique variant is more at risk for injury because of its decreased joint surface area causing decreased rotational mobility.1,2,10
Early recognition is extremely important in dislocations and subluxations of the PTFJ as undiagnosed acute trauma can turn into chronic subluxation, and chronic subluxation may lead to dislocation.13 Additionally, chronic subluxation or dislocation are thought result in osteoarthritis of the PTFJ.14
OSTEOARTHRITIS
The literature on osteoarthritis of the PTFJ is limited. Eichenblat and Nathan7 studied the PTFJ in cadavers and dry bones and found that 28% had evidence of osteoarthritis. Clinically, however, osteoarthritis of the PTFJ is a rare primary diagnosis, suggesting that involvement of the PTFJ is either asymptomatic or that symptoms are associated with osteoarthritis of the knee joint. Boya and colleagues15 and Eichenblat and Nathan7 both found a high correlation between the presence of osteoarthritis of the PTFJ and osteoarthritis of the tibiofemoral joint in cadavers. The authors suggest this correlation may be related to the presence of anatomical communication between the 2 joints. Theoretically, inflammatory mediators flow freely between the joint spaces and contribute to arthritis in both joints. The possibility of degenerative arthritis of the PTFJ accompanying degenerative arthritis of the knee warrants evaluation, especially in patients considering total knee arthroplasty. Unrecognized arthritis of the PTFJ might influence outcome scores and be an unsolved source of lateral knee pain post-knee replacement.16
Continue to: CLINICAL PRESENTATION
CLINCIAL PRESENTATION
ACUTE DISLOCATION
Patients with acute PTFJ dislocation present with pain, tenderness, swelling, and asymmetry of the lateral side of the knee, while the knee joint is not swollen and range of knee motion is not limited.17 A bony prominence might be felt, and the biceps femoris tendon can often appear to be tense.13 Active or passive ankle movements often exacerbate the lateral knee pain.11 It is also important to examine the peroneal nerve, as transient peroneal palsy has been described in all types of PTFJ dislocations but most often with posteromedial dislocations. Sensory disturbance in the peroneal nerve distribution is more common than motor loss, but foot drop is also a potential presenting sign.11 On examination, palpation of the fibular head illustrates tenderness and aggravates the pain.
SUBLUXATION/CHRONIC INSTABILITY
Subluxation of the PTFJ can be difficult to recognize because the history, signs, and symptoms of lateral knee pain can be subtle and sometimes misleading. In addition, current literature provides little information on specific tests, measurements, signs, or subjective information regarding subluxation. Patients rarely reveal a history of trauma or mechanism of injury. Subluxations are often associated with patients participating in repetitive sports requiring running, jumping, or twisting movements, and can be present bilateral. Instability has also been described in patients with osteomyelitis, rheumatoid arthritis, septic arthritis, pigmented villonodular synovitis, below-knee amputations, osteochondroma, and in runners who recently increased mileage (especially during the first 2-3 miles and during downhill running).11,13 Patients normally do not have difficulty with activities of daily living, but symptoms may arise when making movements with a sudden change of direction.11
These patients usually complain of instability of the knee and pain along the lateral aspect of the knee. Pain radiating proximally into the region of the iliotibial band and medially into the patellofemoral joint can be seen.13 Patients may also report clicking, popping, or catching of the lateral knee; while others will report a sense of giving way of the knee joint.11,13 Progressive peroneal nerve symptoms are usually seen in older patients; however, they are more common with acute PTFJ dislocations as discussed.13
A clinical method for examining a PTFJ with possible subluxation or chronic instability has been described by Sijbrandij.18 With the patient in the supine position, the knee is flexed to 90° to relax the LCL and biceps femoris tendon. The fibular head is then held between the thumb and index finger, and moved anteriorly and laterally. Dislocation or subluxation will be felt and visualized as the fibular head translates, and should be compared with the uninjured PTFJ. On release, the fibular head will return to its normal position, often with a click. Asking the patient if this subluxation/reduction maneuver reproduces the symptoms or causes apprehension or pain may also be helpful.18 Another method for examination is eliciting the Radulescu sign.11,13 While the patient lies prone, the examiner stabilizes the thigh with 1 hand while the knee is flexed to 90°. The examiner then applies an internal rotation force on the lower leg. Observing an abnormal excursion of the fibular head in an anterior and lateral direction represents a positive test.11,13
OSTEOARTHRITIS
Clinical evaluation for osteoarthritis in the PTFJ is not well described in the literature. A single report describes applying manual pressure over the fibular head during active ankle motion.16 A test known as the grinding test is used as a sign to detect the involvement of the PTFJ as a component of osteoarthritis of the knee. A positive test will elicit pain and/or tenderness of the joint.16
Continue to: DIAGNOSTIC IMAGING
DIAGNOSTIC IMAGING
Plain radiographs in the anteroposterior (AP) and true lateral views are useful as first-line investigations in suspected PTFJ dislocation. Comparable AP and lateral radiographs of each knee are highly recommended to detect findings that suggest dislocation.2 Abnormal findings include increased interosseous space, medial or lateral displacement of the fibula on the anteroposterior view, and anterior or posterior displacement of the fibula head on lateral view as shown in Figure 2.19,20
Resnick and colleagues2 proposed the use of the linear sloping radiodensity that defines the posteromedial corner of the lateral tibial condyle as an indicator of anterolateral or posteromedial PTFJ dislocation. However, this application is limited because of the PTFJ’s highly variable morphology.8 In a recent study conducted by Hey and colleagues,21 5968 (2984 patients) knee radiographs were retrospectively collected and subjected to radiographical measurements and statistical analysis. The tibiofibular overlap method had a specificity of 94.1% and 84.5% when diagnosing PTFJ dislocations on the AP and lateral views, respectively.21
If a diagnosis of PTFJ is suspected but not clearly established based on radiography, computed tomography with comparison views of the contralateral knee are recommended to confirm the diagnosis.17,22 This becomes more critical in cases of suspected subluxation/chronic PTFJ instability. Additionally, magnetic resonance imaging (MRI) can be used to assess chronic PTFJ instability. Recently, Burke and colleagues23 performed a 10-year retrospective case series that included 7 patients with chronic PTFJ instability and included MRI as part of their evaluation. The MRI abnormalities in these patients included periarticular soft tissue edema, including in the proximal soleus muscle (n = 5), periarticular ganglion or ganglia (n = 4), tibiofibular ligament edema (n = 4), subchondral marrow edema (n = 3), posterior tibiofibular ligament thickening (n = 2), subcortical cyst at a ligament insertion (n = 2), partial-thickness tear of the anterior tibiofibular ligament (n = 1), and tibiofibular joint effusion (n = 1).
OSTEOARTHRITIS
Routine knee radiographs can show PTFJ joint space narrowing, sclerosis, marginal osteophytes, and local osteopenia as conventional components of osteoarthritis of any joint. Serial radiographs have also been described as effective in evaluating progressive degenerative changes of the PTFJ.14 An MRI will show osteophyte formation, subchondral cysts, subchondral sclerosis, joint effusion, joint space narrowing, and is highly sensitive for detecting degenerative changes in cartilage, as well as identifying other possible pathologies such as synovial cysts or pigmented villonodular synovitis. Chronic PTFJ instability appears to predispose to tibiofibular osteoarthritis as reported by Burke and colleagues,23 who found a particularly high incidence (42.9%) of osteoarthritis in patients with chronic PTFJ instability. Additionally, Veth and colleagues14 found degenerative changes in 8 of 19 patients presenting with PTFJ dislocations.
TREATMENT
ACUTE DISLOCATION
Prompt recognition and treatment of any acute PTFJ dislocation are necessary to avoid long-term instability and other possible sequelae.11 Treatment consists of reduction followed by restriction of weight-bearing.11 Traditionally, the knee is immobilized with a cast in extension for 3 to 4 weeks followed by knee mobilization and progressive range of motion exercises,24 but there is some controversy regarding complete immobilization.11,25,26
Continue to: Initially, closed reduction...
Initially, closed reduction is advised as the treatment for acute PTFJ dislocation.11,19,24 It involves placing the knee in 80°to 110° of flexion to relax the biceps femoris and LCL, then applying an appropriate force to the fibular head in a direction opposite the displacement.11,24 An audible pop is often heard as the fibula reduces back into normal alignment. Stability of the reduction and stability of the knee should be determined with respect to both posterolateral structures and the LCL after reduction.11 Anterolateral dislocations are usually easier to reduce, as a posteromedial or superior dislocation can result in the fibular head being perched on the lateral tibial ridge, and held by the LCL.11
Calabró and colleagues27 described a new, simple, and safe alternative technique of closed reduction of an anterior dislocation if the classical method fails. This technique relies on ligamentotaxis and a dynamic counteraction between muscles and ligaments to reduce the joint. The patient flexes the knee >90° while the physician applies a counterforce to the heel with the palm. Simultaneously, gentle direct pressure should be applied to the fibular head to move it toward the lateral tibial ridge. With a relaxed LCL, the biceps femoris tendon will actively reduce the proximal fibular head back into its correct anatomic orientation.27
When a closed reduction in an awake patient has failed, a reduction under sedation or anesthesia should be performed. If that fails, an open reduction should be performed. Following an open reduction, the joint is stabilized with Kirschner wires, bioabsorbable pins, or cortical screws.24-26,28 The torn capsule and any injured ligaments should also be primarily repaired. After approximately 6 weeks, the stabilization hardware may be removed.24,25
Acute posteromedial dislocations are treated similarly to anterolateral dislocations; however, open reduction and repair of the capsule and ligaments are more frequently required.10 Superior dislocations are also more frequently reduced by open methods, sometimes dictated by open treatment of associated tibia or ankle fracture.10 Damage to any structure of the posterolateral knee as a result of acute PTFJ injury should be repaired, as this has been associated with better outcomes.14
PTFJ dislocation and tibia fracture can occur together, and in a retrospective study conducted by Herzog and colleagues29 the authors recorded the incidence of PTFJ dislocation as 1.5% of operative tibial shaft fractures and 1.9% of operative tibial plateau fractures. Haupt and colleagues30 also conducted a retrospective study in which the authors recorded the incidence of PTFJ dislocations in 1.06% of all tibial shaft fractures in their series. In both studies combined, all except 1 PTFJ dislocation had been caused by high-energy trauma.29,30 In the case of PTFJ dislocation with tibial shaft fracture, intramedullary nailing of the tibial shaft fracture followed by open reduction of the PTFJ with 1 or 2 positioning screws just below the PTFJ has yielded satisfactory results.30 The positioning screw should be removed 6 weeks post-operation to prevent PTFJ arthrodesis and patients should be supported in full weight-bearing.30 An illustrative case report from our institution is shown in Figure 3.
Continue to: CHRONIC INSTABILITY
CHRONIC INSTABILITY
Chronic instability is commonly the result of untreated or misdiagnosed subluxation of the PTFJ. Ogden10 reported that 57% of patients with acute proximal tibiofibular dislocations required surgery for ongoing symptoms after treatment failure with closed reduction and 3 weeks of immobilization. The first step in the management of chronic instability of the PTFJ is usually a nonoperative approach. Immobilization, activity level modification, utilization of a supportive strap placed 1 centimeter below the fibular head for pain relief, and participation in a strength-training program of the lower leg are initial treatment recommendations.10,11,13 Many patients with chronic PTFJ instability do not respond to conservative treatment and may pursue surgical intervention. Surgical treatment options include permanent arthrodesis, resection of the fibular head, soft tissue reconstruction, and temporary fixation.10,13,26,31 Given the rare nature of the injury and lack of data on varying treatments, there is no clear consensus on the optimal surgical procedure.
Arthrodesis and fibular head resection are 2 traditional methods of surgically addressing the PTFJ, but both have limitations that need to be recognized. Arthrodesis involves clearing the PTFJ of all articular cartilage, bone grafting, and then reducing the joint using screw fixation.11 Rigid fixation prevents rotation of the fibula which puts additional stress on the ankle, frequently causing pain and instability of the ankle joint.10,11 The other traditional surgical option, fibular head resection, involves excision of the head and neck of the fibula while preserving the fibular styloid and LCL.4 Fibular head resection is indicated when peroneal nerve symptoms or palsy occur in PTFJ injuries.4,10 Unfortunately, resection may disrupt the posterolateral corner structures of the knee and result in pain and instability.3,13 As a general rule, it is advisable to avoid arthrodesis and fibular head resection in children and athletes because of the length of time during which complications can develop.11
Van den Bekerom and colleagues26 suggest that their technique of temporary fixation of the PTFJ using a cancellous screw yields satisfactory outcomes in treating chronic PTFJ instability. The method entails having the ankle dorsiflexed and the head of the fibula slightly externally rotated and reduced into the most stable position. A hole is drilled in the anteromedial direction from posterior fibula head into the tibia. A non-tapped cortical screw is used to fix the fibula head in the reduced position.26 The screw is removed after 3 to 6 months. Seven of 8 patients treated with this technique by Van den Bekerom and colleagues26 have had alleviation of symptoms, although the screws broke in 2 cases before their planned removal.
Soft tissue reconstruction of the PTFJ has been an evolving area of treatment. Several techniques using a variety of tissue grafts and fixation methods have been described. Giachino32 proposed a reconstruction in which the fibular head is stabilized with a strip of ipsilateral biceps femoris tendon still attached distally to the fibular head and deep fascia of the leg. Drill holes are made in the tibia, and the tissue is secured anteriorly to the fascia with a suture. Shapiro and colleagues33 performed a stabilization using a strip of the iliotibial band still connected to its insertion on Gerdy’s tubercle. The graft was tunneled through a tibial drill hole from anterior to posterior and through the fibular head from posterior to anterior before suturing back onto itself. Another technique described by Mena and colleagues34 uses a split biceps tendon autograft, harnessing the strong attachments of the tendon to the fibular head to stabilize the reduced PTFJ.
More recently, Miller35 employed elements of the techniques described by Gianchino,32 Shapiro and colleagues,33 and Mena and colleagues34 to develop a method involving the biceps femoris tendon and iliotibial band in a soft tissue reconstruction. The technique involves harvesting the biceps femoris tendon while preserving the distal insertion and harvesting the iliotibial band while leaving the distal insertion at the Gerdy’s tubercle intact.35 The biceps tendon is passed from posterior to anterior through a tibial bone tunnel, and the iliotibial band graft is passed through the same tunnel but in the anterior to posterior direction. A bioabsorbable interference screw is placed into the tibial tunnel from anterior to posterior. The remaining biceps tendon graft is sewn into the soft tissue of the anterior tibia. The tail of the iliotibial band graft is passed through the fibular bone tunnel in the posterior to anterior direction and looped around the fibular head and under the LCL before being sewn back onto itself.35 Kobbe and colleagues36 described a surgical technique using the ipsilateral semitendinosis tendon while Maffulli and colleagues37 and Morrison and colleagues38 presented techniques using the ipsilateral gracilis tendon. These techniques do not jeopardize lateral knee or fibular head stability by avoiding the use of the biceps femoris and iliotibial band, as well as local knee stabilizers. Warner and colleagues31 proposed a similar technique to the aforementioned using a semitendinosus tendon; however, they do not reconstruct the anterior ligamentous structures, as seen in Figures 4A and 4B.39 The authors have concerns that a 2-limb reconstruction as described by Kobbe and colleagues36 and Morrison and colleagues38 may be prone to overconstraint or other errors in tensioning because it does not allow for differential tension to be applied to each limb.31 According to Warner and colleagues,31 the anterior structures of their patients with chronic PTFJ instability have appeared normal, and isolated posterior ligamentous reconstruction has been adequate for the restoration of stability. Camarda and colleagues28 proposed a technique very similar to that of Warner and colleagues;31 however, the tibial tunnel is reamed from posterolateral to the anteromedial aspect of the tibia, exploiting the skin incision previously used for tendon harvest.
Continue to: ARTHRITIS
ARTHRITIS
The management options for secondary arthritis due to chronic PTFJ instability have rarely been discussed in the literature. Arthrodesis or fibular head resection are options for the treatment of arthritis, and the above discussion applies here as well. Yaniv and colleagues40 describe a technical procedure for addressing both instability and secondary joint arthritis. The authors performed a ligament reconstruction of the PTFJ using the anterior part of the biceps femoris combined with interpositional joint arthroplasty using a vascularized fascia lata strip. Two weeks post-operation, the patient was walking with full weight-bearing and 6 weeks post-operation the first sports activities were allowed.40
CONCLUSION
Conditions affecting the PTFJ, their diagnosis, and treatment are infrequent topics of discussion in the literature. While PTFJ injury, instability, and other disease states are admittedly rare, clinicians need to include them in the differential diagnosis of patients presenting with lateral knee complaints. Diagnostic imaging is a critical component in early identification of PTFJ conditions to prevent long-term complications. Most injuries are treated first with conservative methods, reserving surgery as an option when first-line measures are unsuccessful. Advancements in surgical options for dislocation and subluxation/chronic instability of the joint have been made, but further research on their effectiveness and long-term outcomes is needed before a gold-standard treatment can be determined.
- Ogden JA. The anatomy and function of the proximal tibiofibular joint. Clin Orthop Relat Res. 1974(101):186-191.
- Resnick D, Newell JD, Guerra J, Jr., Danzig LA, Niwayama G, Goergen TG. Proximal tibiofibular joint: anatomic-pathologic-radiographic correlation. AJR Am J Rroentgenol. 1978;131(1):133-138. doi: 10.2214/ajr.131.1.133.
- See A, Bear RR, Owens BD. Anatomic mapping for surgical reconstruction of the proximal tibiofibular ligaments. Orthopedics. 2013;36(1):e58-63. doi: 10.3928/01477447-20121217-19.
- Ogden JA. Subluxation of the proximal tibiofibular joint. Clin Orthop Relat Res. 1974(101):192-197. doi: 10.2106/00004623-197456010-00015
- Bozkurt M, Yilmaz E, Akseki D, Havitcioglu H, Gunal I. The evaluation of the proximal tibiofibular joint for patients with lateral knee pain. Knee. 2004;11(4):307-312. doi: 10.1016/j.knee.2003.08.006
- Dirim B, Wangwinyuvirat M, Frank A, et al. Communication between the proximal tibiofibular joint and knee via the subpopliteal recess: MR arthrography with histologic correlation and stratigraphic dissection. AJR Am J Roentgenol. 2008;191(2):W44-W51. doi: 10.2214/AJR.07.3406.
- Eichenblat M, Nathan H. The proximal tibio fibular joint. An anatomical study with clinical and pathological considerations. Int Orthop. 1983;7(1):31-39. doi: 10.1007/bf00267557
- Espregueira-Mendes JD, da Silva MV. Anatomy of the proximal tibiofibular joint. Knee Surg Sports Traumatol Arthrosc. 2006;14(3):241-249. doi: 10.1007/s00167-005-0684-z.
- Scott J, Lee H, Barsoum W, van den Bogert AJ. The effect of tibiofemoral loading on proximal tibiofibular joint motion. J Anat. 2007;211(5):647-653. doi: 10.1111/j.1469-7580.2007.00803.x.
- Ogden JA. Subluxation and dislocation of the proximal tibiofibular joint. J Bone Joint Surg Am. 1974;56(1):145-154. doi: 10.2106/00004623-197456010-00015
- Sekiya JK, Kuhn JE. Instability of the proximal tibiofibular joint. J Am Acad Orthop Surg. 2003;11(2):120-128. doi: 10.5435/00124635-200303000-00006
- Horan J, Quin G. Proximal tibiofibular dislocation. Emerg Med Jl : EMJ. 2006;23(5):e33. doi: 10.1136/emj.2005.032144.
- Semonian RH, Denlinger PM, Duggan RJ. Proximal tibiofibular subluxation relationship to lateral knee pain: a review of proximal tibiofibular joint pathologies. J Orthop Sports Phys Ther. 1995;21(5):248-257. doi: 10.2519/jospt.1995.21.5.248.
- Veth RP, Kingma LM, Nielsen HK. The abnormal proximal tibiofibular joint. Arch Orthop Trauma Surg. 1984;102(3):167-171. doi: 10.1007/bf00575227
- Boya H, Ozcan O, Oztekin HH. Radiological evaluation of the proximal tibiofibular joint in knees with severe primary osteoarthritis. Knee Surg Sports Traumatol Arthrosc. 2008;16(2):157-159. doi: 10.1007/s00167-007-0442-5.
- Öztuna V, Yildiz A, Özer C, Milcan A, Kuyurtar F, Turgut A. Involvement of the proximal tibiofibular joint in osteoarthritis of the knee. The Knee. 2003;10(4):347-349. doi: 10.1016/s0968-0160(03)00004-8
- Milankov M, Kecojević V, Gvozdenović N, Obradović M. Dislocation of the proximal tibiofibular joint. Med Pregl. 2013;66(9-10):387-391. doi: 10.2298/mpns1310387m
- Sijbrandij S. Instability of the proximal tibio-fibular joint. Acta Orthop Scand. 1978;49(6):621-626. doi: 10.3109/17453677808993250
- Aladin A, Lam KS, Szypryt EP. The importance of early diagnosis in the management of proximal tibiofibular dislocation: a 9- and 5-year follow-up of a bilateral case. Knee. 2002;9(3):233-236. doi: 10.1016/S0968-0160(02)00012-1
- Turco VJ, Spinella AJ. Anterolateral dislocation of the head of the fibula in sports. Am J Sports Med. 1985;13(4):209-215. doi: 10.1177/036354658501300401.
- Hey HW, Ng LW, Ng YH, Sng WZ, Manohara R, Thambiah JS. Radiographical definition of the proximal tibiofibular joint - A cross-sectional study of 2984 knees and literature review. Injury. 2016;47(6):1276-1281. doi: 10.1016/j.injury.2016.01.035.
- Voglino JA, Denton JR. Acute traumatic proximal tibiofibular joint dislocation confirmed by computed tomography. Orthopedics. 1999;22(2):255-258.
- Burke CJ, Grimm LJ, Boyle MJ, Moorman CT, 3rd, Hash TW, 2nd. Imaging of Proximal Tibiofibular Joint Instability: A 10 year retrospective case series. Clin Imaging. 2016;40(3):470-476. doi: 10.1016/j.clinimag.2015.12.011.
- Parkes JC II, Zelko RR. Isolated acute dislocation of the proximal tibiofibular joint. Case report. J Bone Joint Surg Am. 1973;55(1):177-183. Doi: 10.2106/00004623-197355010-00019
- Gvozdenović N, Gvozdenović K, Obradović M, Stanković M. Modified technique of the treatment for proximal tibiofibular joint dislocation. Vojnosanitetski Pregled. 2017;74(3):282-286. doi: 10.2298/VSP150318177G
- van den Bekerom MP, Weir A, van der Flier RE. Surgical stabilisation of the proximal tibiofibular joint using temporary fixation: a technical note. Acta Orthop Belg. 2004;70(6):604-608.
- Calabró T, Cevolani L, Chehrassan M, Gasbarrini A. A new technique of reduction for isolated proximal tibiofibular joint dislocation: a case report. Eur Rev Med Pharmacol Sci. 2014;18(1):93-95.
- Camarda L, Abruzzese A, D'Arienzo M. Proximal tibiofibular joint reconstruction with autogenous semitendinosus tendon graft. Tech Orthop. 2013;28(3):269-272. doi. 10.1097/BTO.0b013e31827b7182
- Herzog GA, Serrano-Riera R, Sagi HC. Traumatic Proximal Tibiofibular Dislocation: A Marker of Severely Traumatized Extremities. J Orthop Trauma. 2015;29(10):456-459. doi: 10.1097/BOT.0000000000000348.
- Haupt S, Frima H, Sommer C. Proximal tibiofibular joint dislocation associated with tibial shaft fractures - 7 cases. Injury. 2016;47(4):950-953. doi: 10.1016/j.injury.2016.01.037.
- Warner BT, Moulton SG, Cram TR, LaPrade RF. Anatomic Reconstruction of the Proximal Tibiofibular Joint. Arthrosc Tech. 2016;5(1):e207-e210. doi: 10.1016/j.eats.2015.11.004
- Giachino AA. Recurrent dislocations of the proximal tibiofibular joint. Report of two cases. J Bone Joint Surg Am. 1986;68(7):1104-1106. doi: 10.2106/00004623-198668070-00023
- Shapiro GS, Fanton GS, Dillingham MF. Reconstruction for recurrent dislocation of the proximal tibiofibular joint. A new technique. Orthop Rev. 1993;22(11):1229-1232.
- Mena H, Brautigan B, Johnson DL. Split biceps femoris tendon reconstruction for proximal tibiofibular joint instability. Arthroscopy. 2001;17(6):668-671. doi: 10.1053/jars.2001.22359.
- Miller T. New technique of soft tissue reconstruction for proximal tibiofibular joint instability using iliotibial band and biceps femoris longhead autograft. Tech Orthop. 2014;29(4):243-247. doi: 10.1097/BTO.0000000000000046
- Kobbe P, Flohe S, Wellmann M, Russe K. Stabilization of chronic proximal tibiofibular joint instability with a semitendinosus graft. Acta Orthop Belg. 2010;76(6):830-833.
- Maffulli N, Spiezia F, Oliva F, Testa V, Capasso G, Denaro V. Gracilis autograft for recurrent posttraumatic instability of the superior tibiofibular joint. Am J Sports Med. 2010;38(11):2294-2298. doi: 10.1177/0363546510373472.
- Morrison TD, Shaer JA, Little JE. Bilateral, atraumatic, proximal tibiofibular joint instability. Orthopedics. 2011;34(2):133. doi: 10.3928/01477447-20101221-28.
- Horst PK, LaPrade RF. Anatomic reconstruction of chronic symptomatic anterolateral proximal tibiofibular joint instability. Knee Surg Sports Traumatol Arthrosc. 2010;18(11):1452-1455. doi: 10.1007/s00167-010-1049-9.
- Yaniv M, Koenig U, Imhoff AB. A technical solution for secondary arthritis due to chronic proximal tibiofibular joint instability. Knee Surg Sports Traumatol Arthrosc. 1999;7(5):334-336. doi: 10.1007/s001670050173.
- Ogden JA. The anatomy and function of the proximal tibiofibular joint. Clin Orthop Relat Res. 1974(101):186-191.
- Resnick D, Newell JD, Guerra J, Jr., Danzig LA, Niwayama G, Goergen TG. Proximal tibiofibular joint: anatomic-pathologic-radiographic correlation. AJR Am J Rroentgenol. 1978;131(1):133-138. doi: 10.2214/ajr.131.1.133.
- See A, Bear RR, Owens BD. Anatomic mapping for surgical reconstruction of the proximal tibiofibular ligaments. Orthopedics. 2013;36(1):e58-63. doi: 10.3928/01477447-20121217-19.
- Ogden JA. Subluxation of the proximal tibiofibular joint. Clin Orthop Relat Res. 1974(101):192-197. doi: 10.2106/00004623-197456010-00015
- Bozkurt M, Yilmaz E, Akseki D, Havitcioglu H, Gunal I. The evaluation of the proximal tibiofibular joint for patients with lateral knee pain. Knee. 2004;11(4):307-312. doi: 10.1016/j.knee.2003.08.006
- Dirim B, Wangwinyuvirat M, Frank A, et al. Communication between the proximal tibiofibular joint and knee via the subpopliteal recess: MR arthrography with histologic correlation and stratigraphic dissection. AJR Am J Roentgenol. 2008;191(2):W44-W51. doi: 10.2214/AJR.07.3406.
- Eichenblat M, Nathan H. The proximal tibio fibular joint. An anatomical study with clinical and pathological considerations. Int Orthop. 1983;7(1):31-39. doi: 10.1007/bf00267557
- Espregueira-Mendes JD, da Silva MV. Anatomy of the proximal tibiofibular joint. Knee Surg Sports Traumatol Arthrosc. 2006;14(3):241-249. doi: 10.1007/s00167-005-0684-z.
- Scott J, Lee H, Barsoum W, van den Bogert AJ. The effect of tibiofemoral loading on proximal tibiofibular joint motion. J Anat. 2007;211(5):647-653. doi: 10.1111/j.1469-7580.2007.00803.x.
- Ogden JA. Subluxation and dislocation of the proximal tibiofibular joint. J Bone Joint Surg Am. 1974;56(1):145-154. doi: 10.2106/00004623-197456010-00015
- Sekiya JK, Kuhn JE. Instability of the proximal tibiofibular joint. J Am Acad Orthop Surg. 2003;11(2):120-128. doi: 10.5435/00124635-200303000-00006
- Horan J, Quin G. Proximal tibiofibular dislocation. Emerg Med Jl : EMJ. 2006;23(5):e33. doi: 10.1136/emj.2005.032144.
- Semonian RH, Denlinger PM, Duggan RJ. Proximal tibiofibular subluxation relationship to lateral knee pain: a review of proximal tibiofibular joint pathologies. J Orthop Sports Phys Ther. 1995;21(5):248-257. doi: 10.2519/jospt.1995.21.5.248.
- Veth RP, Kingma LM, Nielsen HK. The abnormal proximal tibiofibular joint. Arch Orthop Trauma Surg. 1984;102(3):167-171. doi: 10.1007/bf00575227
- Boya H, Ozcan O, Oztekin HH. Radiological evaluation of the proximal tibiofibular joint in knees with severe primary osteoarthritis. Knee Surg Sports Traumatol Arthrosc. 2008;16(2):157-159. doi: 10.1007/s00167-007-0442-5.
- Öztuna V, Yildiz A, Özer C, Milcan A, Kuyurtar F, Turgut A. Involvement of the proximal tibiofibular joint in osteoarthritis of the knee. The Knee. 2003;10(4):347-349. doi: 10.1016/s0968-0160(03)00004-8
- Milankov M, Kecojević V, Gvozdenović N, Obradović M. Dislocation of the proximal tibiofibular joint. Med Pregl. 2013;66(9-10):387-391. doi: 10.2298/mpns1310387m
- Sijbrandij S. Instability of the proximal tibio-fibular joint. Acta Orthop Scand. 1978;49(6):621-626. doi: 10.3109/17453677808993250
- Aladin A, Lam KS, Szypryt EP. The importance of early diagnosis in the management of proximal tibiofibular dislocation: a 9- and 5-year follow-up of a bilateral case. Knee. 2002;9(3):233-236. doi: 10.1016/S0968-0160(02)00012-1
- Turco VJ, Spinella AJ. Anterolateral dislocation of the head of the fibula in sports. Am J Sports Med. 1985;13(4):209-215. doi: 10.1177/036354658501300401.
- Hey HW, Ng LW, Ng YH, Sng WZ, Manohara R, Thambiah JS. Radiographical definition of the proximal tibiofibular joint - A cross-sectional study of 2984 knees and literature review. Injury. 2016;47(6):1276-1281. doi: 10.1016/j.injury.2016.01.035.
- Voglino JA, Denton JR. Acute traumatic proximal tibiofibular joint dislocation confirmed by computed tomography. Orthopedics. 1999;22(2):255-258.
- Burke CJ, Grimm LJ, Boyle MJ, Moorman CT, 3rd, Hash TW, 2nd. Imaging of Proximal Tibiofibular Joint Instability: A 10 year retrospective case series. Clin Imaging. 2016;40(3):470-476. doi: 10.1016/j.clinimag.2015.12.011.
- Parkes JC II, Zelko RR. Isolated acute dislocation of the proximal tibiofibular joint. Case report. J Bone Joint Surg Am. 1973;55(1):177-183. Doi: 10.2106/00004623-197355010-00019
- Gvozdenović N, Gvozdenović K, Obradović M, Stanković M. Modified technique of the treatment for proximal tibiofibular joint dislocation. Vojnosanitetski Pregled. 2017;74(3):282-286. doi: 10.2298/VSP150318177G
- van den Bekerom MP, Weir A, van der Flier RE. Surgical stabilisation of the proximal tibiofibular joint using temporary fixation: a technical note. Acta Orthop Belg. 2004;70(6):604-608.
- Calabró T, Cevolani L, Chehrassan M, Gasbarrini A. A new technique of reduction for isolated proximal tibiofibular joint dislocation: a case report. Eur Rev Med Pharmacol Sci. 2014;18(1):93-95.
- Camarda L, Abruzzese A, D'Arienzo M. Proximal tibiofibular joint reconstruction with autogenous semitendinosus tendon graft. Tech Orthop. 2013;28(3):269-272. doi. 10.1097/BTO.0b013e31827b7182
- Herzog GA, Serrano-Riera R, Sagi HC. Traumatic Proximal Tibiofibular Dislocation: A Marker of Severely Traumatized Extremities. J Orthop Trauma. 2015;29(10):456-459. doi: 10.1097/BOT.0000000000000348.
- Haupt S, Frima H, Sommer C. Proximal tibiofibular joint dislocation associated with tibial shaft fractures - 7 cases. Injury. 2016;47(4):950-953. doi: 10.1016/j.injury.2016.01.037.
- Warner BT, Moulton SG, Cram TR, LaPrade RF. Anatomic Reconstruction of the Proximal Tibiofibular Joint. Arthrosc Tech. 2016;5(1):e207-e210. doi: 10.1016/j.eats.2015.11.004
- Giachino AA. Recurrent dislocations of the proximal tibiofibular joint. Report of two cases. J Bone Joint Surg Am. 1986;68(7):1104-1106. doi: 10.2106/00004623-198668070-00023
- Shapiro GS, Fanton GS, Dillingham MF. Reconstruction for recurrent dislocation of the proximal tibiofibular joint. A new technique. Orthop Rev. 1993;22(11):1229-1232.
- Mena H, Brautigan B, Johnson DL. Split biceps femoris tendon reconstruction for proximal tibiofibular joint instability. Arthroscopy. 2001;17(6):668-671. doi: 10.1053/jars.2001.22359.
- Miller T. New technique of soft tissue reconstruction for proximal tibiofibular joint instability using iliotibial band and biceps femoris longhead autograft. Tech Orthop. 2014;29(4):243-247. doi: 10.1097/BTO.0000000000000046
- Kobbe P, Flohe S, Wellmann M, Russe K. Stabilization of chronic proximal tibiofibular joint instability with a semitendinosus graft. Acta Orthop Belg. 2010;76(6):830-833.
- Maffulli N, Spiezia F, Oliva F, Testa V, Capasso G, Denaro V. Gracilis autograft for recurrent posttraumatic instability of the superior tibiofibular joint. Am J Sports Med. 2010;38(11):2294-2298. doi: 10.1177/0363546510373472.
- Morrison TD, Shaer JA, Little JE. Bilateral, atraumatic, proximal tibiofibular joint instability. Orthopedics. 2011;34(2):133. doi: 10.3928/01477447-20101221-28.
- Horst PK, LaPrade RF. Anatomic reconstruction of chronic symptomatic anterolateral proximal tibiofibular joint instability. Knee Surg Sports Traumatol Arthrosc. 2010;18(11):1452-1455. doi: 10.1007/s00167-010-1049-9.
- Yaniv M, Koenig U, Imhoff AB. A technical solution for secondary arthritis due to chronic proximal tibiofibular joint instability. Knee Surg Sports Traumatol Arthrosc. 1999;7(5):334-336. doi: 10.1007/s001670050173.
TAKE-HOME POINTS
- Problems of the proximal tibiofibular joint (PFTJ) should be considered in the differential diagnosis when a patient presents with complaints in the lateral aspect of the knee.
- The primary function of the PTFJ is to transmit and absorb axial loads from weight bearing on the extremity, and to dissipate torsional loads applied to the leg and ankle.
- The most common instability pattern is anterolateral fibular displacement.
- Most proximal tibiofibular joint instabilities can be treated with closed reduction and conservative care, but some require internal fixation or soft-tissue reconstruction.
- Arthritic conditions of the PTFJ are treated similar to those of any diarthrodial joint, with additional option of surgical arthrodesis or resection arthroplasty.
Preoperative Corticosteroid Use for Medical Conditions is Associated with Increased Postoperative Infectious Complications and Readmissions After Total Hip Arthroplasty: A Propensity-Matched Study
ABSTRACT
Systemic corticosteroids are used to treat a number of medical conditions; however, they are associated with numerous adverse effects. The impact of preoperative chronic corticosteroid use on postoperative outcomes following total hip arthroplasty (THA) is unclear. The purpose of this study was to assess the independent effect of chronic systemic preoperative steroid use on short-term perioperative complications and readmissions after THA.
All patients undergoing primary THA in the American College of Surgeons National Surgical Quality Improvement Program registry from 2005 to -–2015 were identified. Patients were considered chronic steroid users if they used any dosage of oral or parenteral steroids for >10 of the preceding 30 days before THA. Two equally sized propensity-matched groups based on preoperative steroid use were generated to account for differences in operative and baseline characteristics between the groups. Thirty-day complications and hospital readmissions rates were compared using bivariate analysis.
Of 101,532 THA patients who underwent primary THA, 3714 (3.7%) were identified as chronic corticosteroid users. Comparison of propensity-matched cohorts identified an increased rate of any complication (odds ratio [OR] 1.30, P = .003), sepsis (OR 2.07, P = .022), urinary tract infection (OR 1.61, P = .020), superficial surgical site infection (OR 1.73, P = .038), and hospital readmission (OR 1.50, P < .001) in patients who used systemic steroids preoperatively. Readmissions in preoperative steroid users were most commonly for infectious reasons.
Patients prescribed chronic corticosteroids are at a significantly increased risk of both 30-day periopative complications and hospital readmissions. This finding has important implications for pre- and postoperative patient counseling as well as preoperative risk stratification.
Continue to: Corticosteroids are powerful...
Corticosteroids are powerful anti-inflammatory steroid hormones that have many indications in the treatment of medical diseases, including advanced or poorly controlled asthma, chronic obstructive pulmonary disease (COPD), inflammatory bowel disease, allergic conditions, among other indications.1-4 In orthopedics and rheumatology, systemic steroids are, at times, used in patients with rheumatoid arthritis, systemic lupus erythematosus, and vasculitides.5-7 Overman and colleagues,8 using data from the National Health and Nutrition Examination Survey between 1999 and 2008 identified both a 1.2% prevalence of chronic corticosteroid usage in the United States across all age groups and a positive correlation between steroid use prevalence and increasing age. In that study, nearly two-thirds of survey respondents reported using corticosteroids chronically for >90 days. Another observational study in the United Kingdom found that long-term steroid prescriptions increased between 1989 to 2008 and that 13.6% of patients with rheumatoid arthritis and 66.5% of patients with polymyalgia rheumatica or giant cell arteritis used long-term steroids.9
Enterally- or parenterally-administered corticosteroids have numerous systemic effects that are of particular relevance to orthopedic surgeons. Corticosteroids induce osteoporosis by preferentially inducing osteoclastic activity while inhibiting the differentiation of osteoblasts, ultimately leading to decreased bone quality and mass.10 As a consequence, patients who have previously used corticosteroids are more than twice as likely to have a hip fracture.11 Steroids also increase the risk of both osteonecrosis and myopathy, among other musculoskeletal effects.12 In addition to orthopedic complications, steroids have broad inhibitory effects on both acquired and innate immunity, which significantly increases the risk of infections.13 This increased risk of infection is dose-dependent14 and synergistic with other immunosuppressive drugs.15
Patients with hip pain may receive localized corticosteroid hip joint injections during the nonoperative management of various hip pathologies, including arthritis, bursitis, and labral tears.16,17 Outcomes of patients who received intra-articular corticosteroid injections before total hip arthroplasty (THA) were evaluated in a systematic review of 9 studies by Pereira and colleagues.17 These authors found that the infection rate (both superficial and deep surgical site infections [SSI]) after THA in patients who received local steroid injection into the hip before surgery was between 0% and 30%.17 However, similar studies assessing the impact that systemic steroids have on outcomes after THA are lacking. Patients who undergo THA for conditions associated with higher lifetime steroid usage have worse outcomes than those who do not. For instance, in patients undergoing THA for rheumatoid arthritis, the rates of both postoperative periprosthetic joint infection and hip dislocation are higher, when compared with osteoarthritis.18,19 However, it is unclear how much of this difference in outcomes is due to the underlying disease, adverse effects of steroids, or both. Given the high prevalence of chronic systemic steroid use, it is essential to elucidate more clearly the impact that these medications have on perioperative outcomes after THA.
Therefore, the purpose of this study was to characterize short-term perioperative outcomes, including complication and readmission rates in patients undergoing THA while taking chronic preoperative corticosteroids. We also sought to identify the most common reasons for hospital readmission in patients who did and did not use long-term steroids.
MATERIALS AND METHODS
STUDY DESIGN AND SETTING
This investigation was a retrospective cohort study that utilized the American College of Surgeons National Surgical Quality Improvement Program (ACS-NSQIP) registry.20 The ACS-NSQIP is a prospectively collected, multi-institutional database that collects demographical information, operative variables, and both postoperative complications and hospital readmission data. Data is collected for up to 30 days after the index procedure, and patients are contacted by telephone if they are discharged before 30 days. Patient data is entered by specially trained surgical clinical reviewers and is routinely audited by the ACS-NSQIP, leading to more accurate data when compared with administrative research databases.21,22 The ACS-NSQIP has been used in orthopedic surgery outcomes-based studies.23-25
Continue to: All patients undergoing...
All patients undergoing THA between 2005 and 2015 were identified in the registry using primary Current Procedural Terminology code 27130. Patients were split into 2 groups based on whether or not they chronically used corticosteroids preoperatively for a medical condition. A patient was considered a chronic corticosteroid user if he/she used oral or parenteral corticosteroids within 30 days before the index procedure for >10 of the preceding 30 days. Those who received a 1-time steroid pulse or those who used topical or inhaled steroids were not considered as steroid users in this study.
BASELINE CHARACTERISTICS AND PERIOPERATIVE OUTCOMES
Baseline patient and operative characteristics, including patient age, gender, body mass index (BMI), functional status, American Society of Anesthesiologists (ASA) class, anesthesia type, operative duration, and medical comorbidities including hypertension, COPD, diabetes mellitus, and smoking history, were compared between both groups. Perioperative outcomes that were assessed in this study include death, renal, respiratory, and cardiac complications, deep vein thrombosis or pulmonary embolism, stroke, sepsis, return to the operating room, urinary tract infection (UTI), wound dehiscence, superficial and deep SSI, need for a blood transfusion within 72 hours of index surgical procedure, and hospital readmissions. Renal complications were defined as acute or progressive renal insufficiency; respiratory complications were defined as failure to wean from the ventilator, need for intubation after the index procedure, and the occurrence of pneumonia; and cardiac complications were defined as myocardial infarction or cardiac arrest requiring cardiopulmonary resuscitation. Patients were excluded if they had missing baseline or operative characteristic data, an unclean wound classification at the time of admission, or if their THA was considered emergent.
STATISTICAL ANALYSIS
A propensity score-matched comparison was performed to adjust for differences in baseline and operative characteristics between the 2 cohorts in this study. In the current study, the propensity score was defined as the conditional probability that a patient chronically used preoperative corticosteroids for a medical condition, as a function of age, BMI, gender, ASA class, functional status, medical comorbidities, anesthesia type, and operative duration. A 1:1 matching with tight calipers (0.0001), and nearest-neighbor matching was used to generate 2 equally-sized, propensity-matched cohorts based on steroid status.26 Nearest-neighbor matching identifies patients in both cohorts with the closest propensity scores for inclusion in propensity-matched cohorts. This matching is continued until 1 group runs out of patients to match. Baseline patient and operative characteristics for the unadjusted and propensity-matched groups were compared using Pearson’s χ2 analysis. Outcomes after THA by steroid status were also compared in both unadjusted and propensity-matched groups. Finally, all patients who were readmitted were identified, and the reason for readmission was determined using the International Classification of Disease Ninth (ICD-9) and Tenth (ICD-10) edition codes. Patients were classified as having an infectious readmission only if the ICD code clearly stated an infectious etiology. For instance, a patient with an intestinal infection due to Clostridium difficile (ICD-9 008.45) was counted as a gastrointestinal infection, whereas diarrhea without a distinctly specified etiology (ICD-9 787.91, ICD-10 R19.7) was counted as a gastrointestinal medical complication. Readmission data was only available in ACS-NSQIP from 2011 to 2015, constituting 92.5% of all patients included in this study. We used SPSS version 23 (IBM Corporation) for all statistical analyses, and defined a significant P value as <.05.
RESULTS
BASELINE PATIENTS AND OPERATIVE CHARACTERISTICS
In total, we identified 101,532 patients who underwent THA (Table 1). O these, 3714 (3.7%) chronically used corticosteroids preoperatively, whereas 97,818 (96.3%) did not.
When the unadjusted cohorts were compared, patients using corticosteroids were more likely to be female, less likely to obese, more likely to have hypertension, diabetes mellitus, COPD, higher ASA class, undergone THA with general anesthesia, and have a dependent functional status (P < .001 for all comparisons). After propensity matching, 2 equally sized cohorts of 3618 patients each were generated based on steroid status and no differences in baseline and operative characteristics were identified between the 2 groups.
Continue to: CLINICAL OUTCOMES BY STEROID STATUS
CLINCIAL OUTCOMES BY STEROID STATUS
A comparison of unadjusted cohorts showed that patients who used preoperative steroids had an increased rate of any complication (7.89%) when compared with those who did not (4.87%) (Table 2).
Similarly, those who used corticosteroids preoperatively had an increased rate of renal complications, respiratory complications, return to the operating room, sepsis, UTI, superficial and deep SSI, and perioperative blood transfusions. They also were more likely to have a 30-day hospital readmission (P < .05 for all comparisons).
When propensity-matched cohorts were compared, patients who used steroids preoperatively were found to have higher rates of any complication (odds Ratio [OR] 1.30, P = .003), sepsis (OR 2.07, P = .022), UTI (OR 1.61, P = .020), superficial SSI (OR 1.73, P = .038), and hospital readmission (OR 1.50, P < .001; Table 3).
REASONS FOR HOSPITAL READMISSION
In total, 3397 patients were readmitted to the hospital within thirty days. Of these, 226 used steroids preoperatively, and 3171 did not (Table 4).
The most common reason for hospital readmission in patients who used preoperative corticosteroids was infectious complications (72 patients, 31.9% of all readmitted patients in this cohort), followed by medical complications (59 patients, 26.1%), and hip-related complications (48 patients, 21.2%). In those who did not use steroids preoperatively, the most common reason for hospital readmission was medical complications (932 patients, 29.4% of all readmitted patients in this cohort), followed by infectious complications (792 patients, 25.0%), and hip-related complications (763 patients, 24.1%).
Continue to: DISCUSSION
DISCUSSION
Nearly 3% of individuals >80 years in the US population chronically use corticosteroids for a medical condition,8 and this rate is likely higher in specific subsets of patients, such as those with rheumatoid arthritis.9 While some studies have assessed the impact of intra-articular corticosteroid hip injections on perioperative outcomes in THA,17 similar studies assessing systemic corticosteroid usage are lacking. The purpose of this study was to characterize short-term perioperative outcomes in patients undergoing THA who chronically use systemic steroids when compared with those who do not. We found that the prevalence of preoperative chronic steroid use in this cohort of THA patients was 3.7%. We also identified increased rates of infectious complications, including sepsis, UTI, and superficial SSI, in patients who used preoperative corticosteroids. Furthermore, we found an increased rate of hospital readmissions in corticosteroid users and identified the most common reason for hospital readmission as infectious complications in this cohort.
The primary finding of this study was an increase in postoperative infections in patients who use preoperative steroids chronically for medical conditions. Immunosuppression has previously been identified as a risk factor for developing periprosthetic joint infections. Tannenbaum and colleagues27 performed a retrospective study of 19 patients who underwent either a kidney or liver transplant and were maintained on an induction regimen of either prednisone and azathioprine or cyclosporine. These 19 patients also underwent either a THA or total knee arthroplasty, and 5 of these patients (26.3%) developed a periprosthetic joint infection after an average of 3.4 years following the arthroplasty procedure. In another study of 37 renal transplant and dialysis patients who underwent a total of 45 THA procedures, there were 3 instances of superficial SSI and 2 instances of deep SSI.28 However, reported infection rates in transplant patients undergoing THA vary significantly, and studies have been unable to assess the true impact that chronic immunosuppression has on perioperative infection rates.29 In this study, patients who used preoperative corticosteroids chronically were at increased risk of perioperative infections, including sepsis, UTI, and superficial SSI.
Deep vein thrombosis is another postoperative complication that has been associated with chronic steroid use.30 In a case-control study of 38,765 patients who developed a venous thromboembolism and 387,650 control patients who did not, Johannesdottir and colleagues30 found an increased thromboembolic risk in current users of systemic glucocorticoids, but not former users, as well as an increased risk as the dose of glucocorticoids increased. We were not able to identify a similar increase in DVT/PE in chronic corticosteroid users, perhaps due to our sample size, or because we could not do subgroup analyses based on the type or dosage of steroid that a patient was taking. Future studies that identify the highest risk patients among those using systemic corticosteroids are important because parenteral corticosteroids are being increasingly used in THA to alleviate postoperative pain as an opioid-sparing measure.31,32
Finally, we also found that patients who use chronic, systemic corticosteroids are at an increased risk for hospital readmission, when compared with those patients who are not using steroids and are most likely to be readmitted for an infectious complication. Schairer and colleagues33 assessed readmission rates after THA and found 30- and 90-day readmission rate of 4% and 7%, respectively. These authors also found that medical complications accounted for approximately 25% of readmissions, and hip-related complications (eg, dislocation, SSI) accounted for >50%. In our study, we found a 30-day readmission rate in non-steroid users of 3.53% and a rate of 6.52% in chronic steroid users. More than 30% of patients using a steroid were readmitted for infectious complications. As THA is becoming increasingly reimbursed under a bundled payments model by Medicare and Medicaid,34-36 reducing short-term readmissions is imperative. Therefore, discharge counseling that emphasizes how to recognize both the signs and symptoms of infection as well as how to prevent infections, such as reducing SSIs through appropriate wound care, may be warranted in higher risk chronic steroid users.
This study has a number of limitations that are inherent to ACS-NSQIP. First, we lacked specific information on a patient’s steroid history, including which corticosteroid they were using, dosage, frequency, and the indication for corticosteroid therapy. Therefore, we were unable to establish a dose-dependent relationship between steroid exposure and postoperative complications after THA. Second, we were able to assess only 30-day rates of complications and readmissions, and therefore, we were unable to identify intermediate- and long-term effects of systemic corticosteroid use on THA. Finally, we could not determine orthopedic- or hip-specific postoperative outcomes, such as functional scores and range of motion.
Continue to: CONCLUSION
CONCLUSION
In conclusion, this study quantified the increased risk for perioperative complications and hospital readmissions in patients who chronically use corticosteroids and are undergoing THA, when compared with those who do not use corticosteroids. These results suggest that patients who are on long-term steroids are at an increased risk for complications, primarily infectious complications. This finding has important implications for patient counseling, preoperative risk stratification, and suggests that higher risk patients, such as chronic steroid users, may benefit from improved discharge care to decrease complication rates.
1. Normansell R, Kew KM, Mansour G. Different oral corticosteroid regimens for acute asthma. Cochrane Database Syst Rev. 2016;13(5):CD011801. doi: 10.1002/14651858.CD011801.pub2.
2. Walters JA, Tan DJ, White CJ, Wood-Baker R. Different durations of corticosteroid therapy for exacerbations of chronic obstructive pulmonary disease. Cochrane Database Syst Rev. 2014;(12):CD006897.
3. Nunes T, Barreiro-de Acosta M, Marin-Jimenez I, Nos P, Sans M. Oral locally active steroids in inflammatory bowel disease. J Crohns Colitis. 2013;7(3):183-191. doi: 10.1016/j.crohns.2012.06.010.
4. Karatzanis A, Chatzidakis A, Milioni A, Vlaminck S, Kawauchi H, Velegrakis S, et al. Contemporary use of corticosteroids in rhinology. Curr Allergy Asthm R. 2017;17(2). doi: 10.1007/s11882-017-0679-0.
5. Parker BJ, Bruce IN. High dose methylprednisolone therapy for the treatment of severe systemic lupus erythematosus. Lupus. 2007;16(6):387-393. doi: 10.1177/0961203307079502.
6. Ferreira JF, Ahmed Mohamed AA, Emery P. Glucocorticoids and rheumatoid arthritis. Rheum Dis Clin North Am. 2016;42(1):33-46. doi: 10.1016/j.rdc.2015.08.006.
7. Buttgereit F, Dejaco C, Matteson EL, Dasgupta B. Polymyalgia rheumatica and giant cell arteritis: a systematic review. JAMA. 2016;315(22):2442-2458. doi: 10.1001/jama.2016.5444.
8. Overman RA, Yeh JY, Deal CL. Prevalence of oral glucocorticoid usage in the United States: a general population perspective. Arthritis Care Res. 2013;65(2):294-298. doi: 10.1002/acr.21796.
9. Fardet L, Petersen I, Nazareth I. Prevalence of long-term oral glucocorticoid prescriptions in the UK over the past 20 years. Rheumatology. 2011;50(11):1982-1990. doi: 10.1093/rheumatology/ker017.
10. Canalis E, Mazziotti G, Giustina A, Bilezikian JP. Glucocorticoid-induced osteoporosis: pathophysiology and therapy.Osteoporos Int. 2007;18(10):1319-1328. doi: 10.1007/s00198-007-0394-0.
11. Kanis JA, Johansson H, Oden A, Johnell O, de Laet C, Melton LJ, et al. A meta-analysis of prior corticosteroid use and fracture risk. J Bone Miner Res. 2004;19(6):893-899. doi: /10.1359/JBMR.040134.
12. Caplan A, Fett N, Rosenbach M, Werth VP, Micheletti RG. Prevention and management of glucocorticoid-induced side effects: a comprehensive review: a review of glucocorticoid pharmacology and bone health. J Am Acad Dermatol. 2017;76(1):1-9. doi: 10.1016/j.jaad.2016.01.062.
13. Cutolo M, Seriolo B, Pizzorni C, Secchi ME, Soldano S, Paolino S, et al. Use of glucocorticoids and risk of infections. Autoimmun Rev. 2008;8(2):153-155. doi: 10.1016/j.autrev.2008.07.010.
14. Blackwood LL, Pennington JE. Dose-dependent effect of glucocorticosteroids on pulmonary defenses in a steroid-resistant host. Am Rev Respir Dis. 1982;126(6):1045-1049.
15. Toruner M, Loftus EV, Jr., Harmsen WS, Zinsmeister AR, Orenstein R, Sandborn WJ, et al. Risk factors for opportunistic infections in patients with inflammatory bowel disease. Gastroenterology. 2008;134(4):929-936. doi: 10.1053/j.gastro.2008.01.012.
16. Barratt PA, Brookes N, Newson A. Conservative treatments for greater trochanteric pain syndrome: a systematic review. Br J Sports Med. 2017;51(2):97-104. doi: 10.1136/bjsports-2015-095858.
17. Pereira LC, Kerr J, Jolles BM. Intra-articular steroid injection for osteoarthritis of the hip prior to total hip arthroplasty: is it safe? a systematic review. Bone Joint J. 2016;98-B(8):1027-1035. doi: 10.1302/0301-620X.98B8.37420.
18. Ravi B, Escott B, Shah PS, Jenkinson R, Chahal J, Bogoch E, et al. A systematic review and meta-analysis comparing complications following total joint arthroplasty for rheumatoid arthritis versus for osteoarthritis. Arthritis Rheum. 2012;64(12):3839-3849. doi: 10.1002/art.37690.
19. Ravi B, Croxford R, Hollands S, Paterson JM, Bogoch E, Kreder H, et al. Increased risk of complications following total joint arthroplasty in patients with rheumatoid arthritis. Arthritis Rheumatol. 2014;66(2):254-263. doi: 10.1002/art.38231.
20. ACS NSQIP Participant Use Data Files. https://www.facs.org/quality-programs/acs-nsqip/program-specifics/participant-use. Accessed December 6, 2018.
21. Lawson EH, Louie R, Zingmond DS, Brook RH, Hall BL, Han L, et al. A comparison of clinical registry versus administrative claims data for reporting of 30-day surgical complications. Ann Surg. 2012;256(6):973-981. doi: 10.1097/SLA.0b013e31826b4c4f.
22. Weiss A, Anderson JE, Chang DC. Comparing the national surgical quality improvement program with the nationwide inpatient sample database. JAMA Surg. 2015;150(8):815-816. doi: 10.1001/jamasurg.2015.0962.
23. Boddapati V, Fu MC, Mayman DJ, Su EP, Sculco PK, McLawhorn AS. Revision total knee arthroplasty for periprosthetic joint infection is associated with increased postoperative morbidity and mortality relative to noninfectious revisions. J Arthroplasty. 2018;33(2):521-526. doi: 10.1016/j.arth.2017.09.021.
24. Boddapati V, Fu MC, Schairer WW, Gulotta LV, Dines DM, Dines JS. Revision total shoulder arthroplasty is associated with increased thirty-day postoperative complications and wound infections relative to primary total shoulder arthroplasty. HSS J. 2018;14(1):23-28. doi: 10.1007/s11420-017-9573-5.
25. Boddapati V, Fu MC, Schiarer WW, Ranawat AS, Dines DM, Taylor SA, Dines DM. Increased shoulder arthroscopy time is associated with overnight hospital stay and surgical site infection. Arthroscopy. 2018;34(2):363-368. doi: 10.1016/j.arthro.2017.08.243.
26. Lunt M. Selecting an appropriate caliper can be essential for achieving good balance with propensity score matching. Am J Epidemiol. 2014 Jan 15;179(2):226-235. doi: 10.1093/aje/kwt212.
27. Tannenbaum DA, Matthews LS, Grady-Benson JC. Infection around joint replacements in patients who have a renal or liver transplantation. J Bone Joint Surg Am. 1997;79(1):36-43.
28. Shrader MW, Schall D, Parvizi J, McCarthy JT, Lewallen DG. Total hip arthroplasty in patients with renal failure: a comparison between transplant and dialysis patients. J Arthroplasty. 2006;21(3):324-329. doi: 10.1016/j.arth.2005.07.008.
29. Nowicki P, Chaudhary H. Total hip replacement in renal transplant patients. J Bone Joint Surg Br. 2007;89(12):1561-1566.
30. Johannesdottir SA, Horváth-Puhó E, Dekkers OM, Cannegieter SC, Jørgensen JO, Ehrenstein V, et al. Use of glucocorticoids and risk of venous thromboembolism: a nationwide population-based case-control study. JAMA Intern Med. 2013;173(9):743-752. doi: 10.1001/jamainternmed.2013.122.
31. Hartman J, Khanna V, Habib A, Farrokhyar F, Memon M, Adili A. Perioperative systemic glucocorticoids in total hip and knee arthroplasty: a systematic review of outcomes. J Orthop. 2017;14(2):294-301. doi: 10.1016/j.jor.2017.03.012.
32. Sculco PK, McLawhorn AS, Desai N, Su EP, Padgett DE, Jules-Elysee K. The effect of perioperative corticosteroids in total hip arthroplasty: a prospective double-blind placebo controlled pilot study. J Arthroplasty. 2016;31(6):1208-1212. doi: 10.1016/j.arth.2015.11.011.
33. Schairer WW, Sing DC, Vail TP, Bozic KJ. Causes and frequency of unplanned hospital readmission after total hip arthroplasty. Clin Orthop Relat Res. 2014;472(2):464-470. doi: 10.1007/s11999-013-3121-5.
34. US Department of Health and Human Services. Comprehensive Care for Joint Replacement Model. Centers for Medicare & Medicaid Services. https://innovation.cms.gov/initiatives/cjr. Accessed June 15, 2017.
35. Bozic KJ, Ward L, Vail TP, Maze M. Bundled payments in total joint arthroplasty: targeting opportunities for quality improvement and cost reduction. Clin Orthop Relat Res. 2014;472(1):188-193. doi: 10.1007/s11999-013-3034-3.
36. Bosco JA, 3rd, Karkenny AJ, Hutzler LH, Slover JD, Iorio R. Cost burden of 30-day readmissions following Medicare total hip and knee arthroplasty. J Arthroplasty. 2014;29(5): 903-905. doi: 10.1016/j.arth.2013.11.006.
ABSTRACT
Systemic corticosteroids are used to treat a number of medical conditions; however, they are associated with numerous adverse effects. The impact of preoperative chronic corticosteroid use on postoperative outcomes following total hip arthroplasty (THA) is unclear. The purpose of this study was to assess the independent effect of chronic systemic preoperative steroid use on short-term perioperative complications and readmissions after THA.
All patients undergoing primary THA in the American College of Surgeons National Surgical Quality Improvement Program registry from 2005 to -–2015 were identified. Patients were considered chronic steroid users if they used any dosage of oral or parenteral steroids for >10 of the preceding 30 days before THA. Two equally sized propensity-matched groups based on preoperative steroid use were generated to account for differences in operative and baseline characteristics between the groups. Thirty-day complications and hospital readmissions rates were compared using bivariate analysis.
Of 101,532 THA patients who underwent primary THA, 3714 (3.7%) were identified as chronic corticosteroid users. Comparison of propensity-matched cohorts identified an increased rate of any complication (odds ratio [OR] 1.30, P = .003), sepsis (OR 2.07, P = .022), urinary tract infection (OR 1.61, P = .020), superficial surgical site infection (OR 1.73, P = .038), and hospital readmission (OR 1.50, P < .001) in patients who used systemic steroids preoperatively. Readmissions in preoperative steroid users were most commonly for infectious reasons.
Patients prescribed chronic corticosteroids are at a significantly increased risk of both 30-day periopative complications and hospital readmissions. This finding has important implications for pre- and postoperative patient counseling as well as preoperative risk stratification.
Continue to: Corticosteroids are powerful...
Corticosteroids are powerful anti-inflammatory steroid hormones that have many indications in the treatment of medical diseases, including advanced or poorly controlled asthma, chronic obstructive pulmonary disease (COPD), inflammatory bowel disease, allergic conditions, among other indications.1-4 In orthopedics and rheumatology, systemic steroids are, at times, used in patients with rheumatoid arthritis, systemic lupus erythematosus, and vasculitides.5-7 Overman and colleagues,8 using data from the National Health and Nutrition Examination Survey between 1999 and 2008 identified both a 1.2% prevalence of chronic corticosteroid usage in the United States across all age groups and a positive correlation between steroid use prevalence and increasing age. In that study, nearly two-thirds of survey respondents reported using corticosteroids chronically for >90 days. Another observational study in the United Kingdom found that long-term steroid prescriptions increased between 1989 to 2008 and that 13.6% of patients with rheumatoid arthritis and 66.5% of patients with polymyalgia rheumatica or giant cell arteritis used long-term steroids.9
Enterally- or parenterally-administered corticosteroids have numerous systemic effects that are of particular relevance to orthopedic surgeons. Corticosteroids induce osteoporosis by preferentially inducing osteoclastic activity while inhibiting the differentiation of osteoblasts, ultimately leading to decreased bone quality and mass.10 As a consequence, patients who have previously used corticosteroids are more than twice as likely to have a hip fracture.11 Steroids also increase the risk of both osteonecrosis and myopathy, among other musculoskeletal effects.12 In addition to orthopedic complications, steroids have broad inhibitory effects on both acquired and innate immunity, which significantly increases the risk of infections.13 This increased risk of infection is dose-dependent14 and synergistic with other immunosuppressive drugs.15
Patients with hip pain may receive localized corticosteroid hip joint injections during the nonoperative management of various hip pathologies, including arthritis, bursitis, and labral tears.16,17 Outcomes of patients who received intra-articular corticosteroid injections before total hip arthroplasty (THA) were evaluated in a systematic review of 9 studies by Pereira and colleagues.17 These authors found that the infection rate (both superficial and deep surgical site infections [SSI]) after THA in patients who received local steroid injection into the hip before surgery was between 0% and 30%.17 However, similar studies assessing the impact that systemic steroids have on outcomes after THA are lacking. Patients who undergo THA for conditions associated with higher lifetime steroid usage have worse outcomes than those who do not. For instance, in patients undergoing THA for rheumatoid arthritis, the rates of both postoperative periprosthetic joint infection and hip dislocation are higher, when compared with osteoarthritis.18,19 However, it is unclear how much of this difference in outcomes is due to the underlying disease, adverse effects of steroids, or both. Given the high prevalence of chronic systemic steroid use, it is essential to elucidate more clearly the impact that these medications have on perioperative outcomes after THA.
Therefore, the purpose of this study was to characterize short-term perioperative outcomes, including complication and readmission rates in patients undergoing THA while taking chronic preoperative corticosteroids. We also sought to identify the most common reasons for hospital readmission in patients who did and did not use long-term steroids.
MATERIALS AND METHODS
STUDY DESIGN AND SETTING
This investigation was a retrospective cohort study that utilized the American College of Surgeons National Surgical Quality Improvement Program (ACS-NSQIP) registry.20 The ACS-NSQIP is a prospectively collected, multi-institutional database that collects demographical information, operative variables, and both postoperative complications and hospital readmission data. Data is collected for up to 30 days after the index procedure, and patients are contacted by telephone if they are discharged before 30 days. Patient data is entered by specially trained surgical clinical reviewers and is routinely audited by the ACS-NSQIP, leading to more accurate data when compared with administrative research databases.21,22 The ACS-NSQIP has been used in orthopedic surgery outcomes-based studies.23-25
Continue to: All patients undergoing...
All patients undergoing THA between 2005 and 2015 were identified in the registry using primary Current Procedural Terminology code 27130. Patients were split into 2 groups based on whether or not they chronically used corticosteroids preoperatively for a medical condition. A patient was considered a chronic corticosteroid user if he/she used oral or parenteral corticosteroids within 30 days before the index procedure for >10 of the preceding 30 days. Those who received a 1-time steroid pulse or those who used topical or inhaled steroids were not considered as steroid users in this study.
BASELINE CHARACTERISTICS AND PERIOPERATIVE OUTCOMES
Baseline patient and operative characteristics, including patient age, gender, body mass index (BMI), functional status, American Society of Anesthesiologists (ASA) class, anesthesia type, operative duration, and medical comorbidities including hypertension, COPD, diabetes mellitus, and smoking history, were compared between both groups. Perioperative outcomes that were assessed in this study include death, renal, respiratory, and cardiac complications, deep vein thrombosis or pulmonary embolism, stroke, sepsis, return to the operating room, urinary tract infection (UTI), wound dehiscence, superficial and deep SSI, need for a blood transfusion within 72 hours of index surgical procedure, and hospital readmissions. Renal complications were defined as acute or progressive renal insufficiency; respiratory complications were defined as failure to wean from the ventilator, need for intubation after the index procedure, and the occurrence of pneumonia; and cardiac complications were defined as myocardial infarction or cardiac arrest requiring cardiopulmonary resuscitation. Patients were excluded if they had missing baseline or operative characteristic data, an unclean wound classification at the time of admission, or if their THA was considered emergent.
STATISTICAL ANALYSIS
A propensity score-matched comparison was performed to adjust for differences in baseline and operative characteristics between the 2 cohorts in this study. In the current study, the propensity score was defined as the conditional probability that a patient chronically used preoperative corticosteroids for a medical condition, as a function of age, BMI, gender, ASA class, functional status, medical comorbidities, anesthesia type, and operative duration. A 1:1 matching with tight calipers (0.0001), and nearest-neighbor matching was used to generate 2 equally-sized, propensity-matched cohorts based on steroid status.26 Nearest-neighbor matching identifies patients in both cohorts with the closest propensity scores for inclusion in propensity-matched cohorts. This matching is continued until 1 group runs out of patients to match. Baseline patient and operative characteristics for the unadjusted and propensity-matched groups were compared using Pearson’s χ2 analysis. Outcomes after THA by steroid status were also compared in both unadjusted and propensity-matched groups. Finally, all patients who were readmitted were identified, and the reason for readmission was determined using the International Classification of Disease Ninth (ICD-9) and Tenth (ICD-10) edition codes. Patients were classified as having an infectious readmission only if the ICD code clearly stated an infectious etiology. For instance, a patient with an intestinal infection due to Clostridium difficile (ICD-9 008.45) was counted as a gastrointestinal infection, whereas diarrhea without a distinctly specified etiology (ICD-9 787.91, ICD-10 R19.7) was counted as a gastrointestinal medical complication. Readmission data was only available in ACS-NSQIP from 2011 to 2015, constituting 92.5% of all patients included in this study. We used SPSS version 23 (IBM Corporation) for all statistical analyses, and defined a significant P value as <.05.
RESULTS
BASELINE PATIENTS AND OPERATIVE CHARACTERISTICS
In total, we identified 101,532 patients who underwent THA (Table 1). O these, 3714 (3.7%) chronically used corticosteroids preoperatively, whereas 97,818 (96.3%) did not.
When the unadjusted cohorts were compared, patients using corticosteroids were more likely to be female, less likely to obese, more likely to have hypertension, diabetes mellitus, COPD, higher ASA class, undergone THA with general anesthesia, and have a dependent functional status (P < .001 for all comparisons). After propensity matching, 2 equally sized cohorts of 3618 patients each were generated based on steroid status and no differences in baseline and operative characteristics were identified between the 2 groups.
Continue to: CLINICAL OUTCOMES BY STEROID STATUS
CLINCIAL OUTCOMES BY STEROID STATUS
A comparison of unadjusted cohorts showed that patients who used preoperative steroids had an increased rate of any complication (7.89%) when compared with those who did not (4.87%) (Table 2).
Similarly, those who used corticosteroids preoperatively had an increased rate of renal complications, respiratory complications, return to the operating room, sepsis, UTI, superficial and deep SSI, and perioperative blood transfusions. They also were more likely to have a 30-day hospital readmission (P < .05 for all comparisons).
When propensity-matched cohorts were compared, patients who used steroids preoperatively were found to have higher rates of any complication (odds Ratio [OR] 1.30, P = .003), sepsis (OR 2.07, P = .022), UTI (OR 1.61, P = .020), superficial SSI (OR 1.73, P = .038), and hospital readmission (OR 1.50, P < .001; Table 3).
REASONS FOR HOSPITAL READMISSION
In total, 3397 patients were readmitted to the hospital within thirty days. Of these, 226 used steroids preoperatively, and 3171 did not (Table 4).
The most common reason for hospital readmission in patients who used preoperative corticosteroids was infectious complications (72 patients, 31.9% of all readmitted patients in this cohort), followed by medical complications (59 patients, 26.1%), and hip-related complications (48 patients, 21.2%). In those who did not use steroids preoperatively, the most common reason for hospital readmission was medical complications (932 patients, 29.4% of all readmitted patients in this cohort), followed by infectious complications (792 patients, 25.0%), and hip-related complications (763 patients, 24.1%).
Continue to: DISCUSSION
DISCUSSION
Nearly 3% of individuals >80 years in the US population chronically use corticosteroids for a medical condition,8 and this rate is likely higher in specific subsets of patients, such as those with rheumatoid arthritis.9 While some studies have assessed the impact of intra-articular corticosteroid hip injections on perioperative outcomes in THA,17 similar studies assessing systemic corticosteroid usage are lacking. The purpose of this study was to characterize short-term perioperative outcomes in patients undergoing THA who chronically use systemic steroids when compared with those who do not. We found that the prevalence of preoperative chronic steroid use in this cohort of THA patients was 3.7%. We also identified increased rates of infectious complications, including sepsis, UTI, and superficial SSI, in patients who used preoperative corticosteroids. Furthermore, we found an increased rate of hospital readmissions in corticosteroid users and identified the most common reason for hospital readmission as infectious complications in this cohort.
The primary finding of this study was an increase in postoperative infections in patients who use preoperative steroids chronically for medical conditions. Immunosuppression has previously been identified as a risk factor for developing periprosthetic joint infections. Tannenbaum and colleagues27 performed a retrospective study of 19 patients who underwent either a kidney or liver transplant and were maintained on an induction regimen of either prednisone and azathioprine or cyclosporine. These 19 patients also underwent either a THA or total knee arthroplasty, and 5 of these patients (26.3%) developed a periprosthetic joint infection after an average of 3.4 years following the arthroplasty procedure. In another study of 37 renal transplant and dialysis patients who underwent a total of 45 THA procedures, there were 3 instances of superficial SSI and 2 instances of deep SSI.28 However, reported infection rates in transplant patients undergoing THA vary significantly, and studies have been unable to assess the true impact that chronic immunosuppression has on perioperative infection rates.29 In this study, patients who used preoperative corticosteroids chronically were at increased risk of perioperative infections, including sepsis, UTI, and superficial SSI.
Deep vein thrombosis is another postoperative complication that has been associated with chronic steroid use.30 In a case-control study of 38,765 patients who developed a venous thromboembolism and 387,650 control patients who did not, Johannesdottir and colleagues30 found an increased thromboembolic risk in current users of systemic glucocorticoids, but not former users, as well as an increased risk as the dose of glucocorticoids increased. We were not able to identify a similar increase in DVT/PE in chronic corticosteroid users, perhaps due to our sample size, or because we could not do subgroup analyses based on the type or dosage of steroid that a patient was taking. Future studies that identify the highest risk patients among those using systemic corticosteroids are important because parenteral corticosteroids are being increasingly used in THA to alleviate postoperative pain as an opioid-sparing measure.31,32
Finally, we also found that patients who use chronic, systemic corticosteroids are at an increased risk for hospital readmission, when compared with those patients who are not using steroids and are most likely to be readmitted for an infectious complication. Schairer and colleagues33 assessed readmission rates after THA and found 30- and 90-day readmission rate of 4% and 7%, respectively. These authors also found that medical complications accounted for approximately 25% of readmissions, and hip-related complications (eg, dislocation, SSI) accounted for >50%. In our study, we found a 30-day readmission rate in non-steroid users of 3.53% and a rate of 6.52% in chronic steroid users. More than 30% of patients using a steroid were readmitted for infectious complications. As THA is becoming increasingly reimbursed under a bundled payments model by Medicare and Medicaid,34-36 reducing short-term readmissions is imperative. Therefore, discharge counseling that emphasizes how to recognize both the signs and symptoms of infection as well as how to prevent infections, such as reducing SSIs through appropriate wound care, may be warranted in higher risk chronic steroid users.
This study has a number of limitations that are inherent to ACS-NSQIP. First, we lacked specific information on a patient’s steroid history, including which corticosteroid they were using, dosage, frequency, and the indication for corticosteroid therapy. Therefore, we were unable to establish a dose-dependent relationship between steroid exposure and postoperative complications after THA. Second, we were able to assess only 30-day rates of complications and readmissions, and therefore, we were unable to identify intermediate- and long-term effects of systemic corticosteroid use on THA. Finally, we could not determine orthopedic- or hip-specific postoperative outcomes, such as functional scores and range of motion.
Continue to: CONCLUSION
CONCLUSION
In conclusion, this study quantified the increased risk for perioperative complications and hospital readmissions in patients who chronically use corticosteroids and are undergoing THA, when compared with those who do not use corticosteroids. These results suggest that patients who are on long-term steroids are at an increased risk for complications, primarily infectious complications. This finding has important implications for patient counseling, preoperative risk stratification, and suggests that higher risk patients, such as chronic steroid users, may benefit from improved discharge care to decrease complication rates.
ABSTRACT
Systemic corticosteroids are used to treat a number of medical conditions; however, they are associated with numerous adverse effects. The impact of preoperative chronic corticosteroid use on postoperative outcomes following total hip arthroplasty (THA) is unclear. The purpose of this study was to assess the independent effect of chronic systemic preoperative steroid use on short-term perioperative complications and readmissions after THA.
All patients undergoing primary THA in the American College of Surgeons National Surgical Quality Improvement Program registry from 2005 to -–2015 were identified. Patients were considered chronic steroid users if they used any dosage of oral or parenteral steroids for >10 of the preceding 30 days before THA. Two equally sized propensity-matched groups based on preoperative steroid use were generated to account for differences in operative and baseline characteristics between the groups. Thirty-day complications and hospital readmissions rates were compared using bivariate analysis.
Of 101,532 THA patients who underwent primary THA, 3714 (3.7%) were identified as chronic corticosteroid users. Comparison of propensity-matched cohorts identified an increased rate of any complication (odds ratio [OR] 1.30, P = .003), sepsis (OR 2.07, P = .022), urinary tract infection (OR 1.61, P = .020), superficial surgical site infection (OR 1.73, P = .038), and hospital readmission (OR 1.50, P < .001) in patients who used systemic steroids preoperatively. Readmissions in preoperative steroid users were most commonly for infectious reasons.
Patients prescribed chronic corticosteroids are at a significantly increased risk of both 30-day periopative complications and hospital readmissions. This finding has important implications for pre- and postoperative patient counseling as well as preoperative risk stratification.
Continue to: Corticosteroids are powerful...
Corticosteroids are powerful anti-inflammatory steroid hormones that have many indications in the treatment of medical diseases, including advanced or poorly controlled asthma, chronic obstructive pulmonary disease (COPD), inflammatory bowel disease, allergic conditions, among other indications.1-4 In orthopedics and rheumatology, systemic steroids are, at times, used in patients with rheumatoid arthritis, systemic lupus erythematosus, and vasculitides.5-7 Overman and colleagues,8 using data from the National Health and Nutrition Examination Survey between 1999 and 2008 identified both a 1.2% prevalence of chronic corticosteroid usage in the United States across all age groups and a positive correlation between steroid use prevalence and increasing age. In that study, nearly two-thirds of survey respondents reported using corticosteroids chronically for >90 days. Another observational study in the United Kingdom found that long-term steroid prescriptions increased between 1989 to 2008 and that 13.6% of patients with rheumatoid arthritis and 66.5% of patients with polymyalgia rheumatica or giant cell arteritis used long-term steroids.9
Enterally- or parenterally-administered corticosteroids have numerous systemic effects that are of particular relevance to orthopedic surgeons. Corticosteroids induce osteoporosis by preferentially inducing osteoclastic activity while inhibiting the differentiation of osteoblasts, ultimately leading to decreased bone quality and mass.10 As a consequence, patients who have previously used corticosteroids are more than twice as likely to have a hip fracture.11 Steroids also increase the risk of both osteonecrosis and myopathy, among other musculoskeletal effects.12 In addition to orthopedic complications, steroids have broad inhibitory effects on both acquired and innate immunity, which significantly increases the risk of infections.13 This increased risk of infection is dose-dependent14 and synergistic with other immunosuppressive drugs.15
Patients with hip pain may receive localized corticosteroid hip joint injections during the nonoperative management of various hip pathologies, including arthritis, bursitis, and labral tears.16,17 Outcomes of patients who received intra-articular corticosteroid injections before total hip arthroplasty (THA) were evaluated in a systematic review of 9 studies by Pereira and colleagues.17 These authors found that the infection rate (both superficial and deep surgical site infections [SSI]) after THA in patients who received local steroid injection into the hip before surgery was between 0% and 30%.17 However, similar studies assessing the impact that systemic steroids have on outcomes after THA are lacking. Patients who undergo THA for conditions associated with higher lifetime steroid usage have worse outcomes than those who do not. For instance, in patients undergoing THA for rheumatoid arthritis, the rates of both postoperative periprosthetic joint infection and hip dislocation are higher, when compared with osteoarthritis.18,19 However, it is unclear how much of this difference in outcomes is due to the underlying disease, adverse effects of steroids, or both. Given the high prevalence of chronic systemic steroid use, it is essential to elucidate more clearly the impact that these medications have on perioperative outcomes after THA.
Therefore, the purpose of this study was to characterize short-term perioperative outcomes, including complication and readmission rates in patients undergoing THA while taking chronic preoperative corticosteroids. We also sought to identify the most common reasons for hospital readmission in patients who did and did not use long-term steroids.
MATERIALS AND METHODS
STUDY DESIGN AND SETTING
This investigation was a retrospective cohort study that utilized the American College of Surgeons National Surgical Quality Improvement Program (ACS-NSQIP) registry.20 The ACS-NSQIP is a prospectively collected, multi-institutional database that collects demographical information, operative variables, and both postoperative complications and hospital readmission data. Data is collected for up to 30 days after the index procedure, and patients are contacted by telephone if they are discharged before 30 days. Patient data is entered by specially trained surgical clinical reviewers and is routinely audited by the ACS-NSQIP, leading to more accurate data when compared with administrative research databases.21,22 The ACS-NSQIP has been used in orthopedic surgery outcomes-based studies.23-25
Continue to: All patients undergoing...
All patients undergoing THA between 2005 and 2015 were identified in the registry using primary Current Procedural Terminology code 27130. Patients were split into 2 groups based on whether or not they chronically used corticosteroids preoperatively for a medical condition. A patient was considered a chronic corticosteroid user if he/she used oral or parenteral corticosteroids within 30 days before the index procedure for >10 of the preceding 30 days. Those who received a 1-time steroid pulse or those who used topical or inhaled steroids were not considered as steroid users in this study.
BASELINE CHARACTERISTICS AND PERIOPERATIVE OUTCOMES
Baseline patient and operative characteristics, including patient age, gender, body mass index (BMI), functional status, American Society of Anesthesiologists (ASA) class, anesthesia type, operative duration, and medical comorbidities including hypertension, COPD, diabetes mellitus, and smoking history, were compared between both groups. Perioperative outcomes that were assessed in this study include death, renal, respiratory, and cardiac complications, deep vein thrombosis or pulmonary embolism, stroke, sepsis, return to the operating room, urinary tract infection (UTI), wound dehiscence, superficial and deep SSI, need for a blood transfusion within 72 hours of index surgical procedure, and hospital readmissions. Renal complications were defined as acute or progressive renal insufficiency; respiratory complications were defined as failure to wean from the ventilator, need for intubation after the index procedure, and the occurrence of pneumonia; and cardiac complications were defined as myocardial infarction or cardiac arrest requiring cardiopulmonary resuscitation. Patients were excluded if they had missing baseline or operative characteristic data, an unclean wound classification at the time of admission, or if their THA was considered emergent.
STATISTICAL ANALYSIS
A propensity score-matched comparison was performed to adjust for differences in baseline and operative characteristics between the 2 cohorts in this study. In the current study, the propensity score was defined as the conditional probability that a patient chronically used preoperative corticosteroids for a medical condition, as a function of age, BMI, gender, ASA class, functional status, medical comorbidities, anesthesia type, and operative duration. A 1:1 matching with tight calipers (0.0001), and nearest-neighbor matching was used to generate 2 equally-sized, propensity-matched cohorts based on steroid status.26 Nearest-neighbor matching identifies patients in both cohorts with the closest propensity scores for inclusion in propensity-matched cohorts. This matching is continued until 1 group runs out of patients to match. Baseline patient and operative characteristics for the unadjusted and propensity-matched groups were compared using Pearson’s χ2 analysis. Outcomes after THA by steroid status were also compared in both unadjusted and propensity-matched groups. Finally, all patients who were readmitted were identified, and the reason for readmission was determined using the International Classification of Disease Ninth (ICD-9) and Tenth (ICD-10) edition codes. Patients were classified as having an infectious readmission only if the ICD code clearly stated an infectious etiology. For instance, a patient with an intestinal infection due to Clostridium difficile (ICD-9 008.45) was counted as a gastrointestinal infection, whereas diarrhea without a distinctly specified etiology (ICD-9 787.91, ICD-10 R19.7) was counted as a gastrointestinal medical complication. Readmission data was only available in ACS-NSQIP from 2011 to 2015, constituting 92.5% of all patients included in this study. We used SPSS version 23 (IBM Corporation) for all statistical analyses, and defined a significant P value as <.05.
RESULTS
BASELINE PATIENTS AND OPERATIVE CHARACTERISTICS
In total, we identified 101,532 patients who underwent THA (Table 1). O these, 3714 (3.7%) chronically used corticosteroids preoperatively, whereas 97,818 (96.3%) did not.
When the unadjusted cohorts were compared, patients using corticosteroids were more likely to be female, less likely to obese, more likely to have hypertension, diabetes mellitus, COPD, higher ASA class, undergone THA with general anesthesia, and have a dependent functional status (P < .001 for all comparisons). After propensity matching, 2 equally sized cohorts of 3618 patients each were generated based on steroid status and no differences in baseline and operative characteristics were identified between the 2 groups.
Continue to: CLINICAL OUTCOMES BY STEROID STATUS
CLINCIAL OUTCOMES BY STEROID STATUS
A comparison of unadjusted cohorts showed that patients who used preoperative steroids had an increased rate of any complication (7.89%) when compared with those who did not (4.87%) (Table 2).
Similarly, those who used corticosteroids preoperatively had an increased rate of renal complications, respiratory complications, return to the operating room, sepsis, UTI, superficial and deep SSI, and perioperative blood transfusions. They also were more likely to have a 30-day hospital readmission (P < .05 for all comparisons).
When propensity-matched cohorts were compared, patients who used steroids preoperatively were found to have higher rates of any complication (odds Ratio [OR] 1.30, P = .003), sepsis (OR 2.07, P = .022), UTI (OR 1.61, P = .020), superficial SSI (OR 1.73, P = .038), and hospital readmission (OR 1.50, P < .001; Table 3).
REASONS FOR HOSPITAL READMISSION
In total, 3397 patients were readmitted to the hospital within thirty days. Of these, 226 used steroids preoperatively, and 3171 did not (Table 4).
The most common reason for hospital readmission in patients who used preoperative corticosteroids was infectious complications (72 patients, 31.9% of all readmitted patients in this cohort), followed by medical complications (59 patients, 26.1%), and hip-related complications (48 patients, 21.2%). In those who did not use steroids preoperatively, the most common reason for hospital readmission was medical complications (932 patients, 29.4% of all readmitted patients in this cohort), followed by infectious complications (792 patients, 25.0%), and hip-related complications (763 patients, 24.1%).
Continue to: DISCUSSION
DISCUSSION
Nearly 3% of individuals >80 years in the US population chronically use corticosteroids for a medical condition,8 and this rate is likely higher in specific subsets of patients, such as those with rheumatoid arthritis.9 While some studies have assessed the impact of intra-articular corticosteroid hip injections on perioperative outcomes in THA,17 similar studies assessing systemic corticosteroid usage are lacking. The purpose of this study was to characterize short-term perioperative outcomes in patients undergoing THA who chronically use systemic steroids when compared with those who do not. We found that the prevalence of preoperative chronic steroid use in this cohort of THA patients was 3.7%. We also identified increased rates of infectious complications, including sepsis, UTI, and superficial SSI, in patients who used preoperative corticosteroids. Furthermore, we found an increased rate of hospital readmissions in corticosteroid users and identified the most common reason for hospital readmission as infectious complications in this cohort.
The primary finding of this study was an increase in postoperative infections in patients who use preoperative steroids chronically for medical conditions. Immunosuppression has previously been identified as a risk factor for developing periprosthetic joint infections. Tannenbaum and colleagues27 performed a retrospective study of 19 patients who underwent either a kidney or liver transplant and were maintained on an induction regimen of either prednisone and azathioprine or cyclosporine. These 19 patients also underwent either a THA or total knee arthroplasty, and 5 of these patients (26.3%) developed a periprosthetic joint infection after an average of 3.4 years following the arthroplasty procedure. In another study of 37 renal transplant and dialysis patients who underwent a total of 45 THA procedures, there were 3 instances of superficial SSI and 2 instances of deep SSI.28 However, reported infection rates in transplant patients undergoing THA vary significantly, and studies have been unable to assess the true impact that chronic immunosuppression has on perioperative infection rates.29 In this study, patients who used preoperative corticosteroids chronically were at increased risk of perioperative infections, including sepsis, UTI, and superficial SSI.
Deep vein thrombosis is another postoperative complication that has been associated with chronic steroid use.30 In a case-control study of 38,765 patients who developed a venous thromboembolism and 387,650 control patients who did not, Johannesdottir and colleagues30 found an increased thromboembolic risk in current users of systemic glucocorticoids, but not former users, as well as an increased risk as the dose of glucocorticoids increased. We were not able to identify a similar increase in DVT/PE in chronic corticosteroid users, perhaps due to our sample size, or because we could not do subgroup analyses based on the type or dosage of steroid that a patient was taking. Future studies that identify the highest risk patients among those using systemic corticosteroids are important because parenteral corticosteroids are being increasingly used in THA to alleviate postoperative pain as an opioid-sparing measure.31,32
Finally, we also found that patients who use chronic, systemic corticosteroids are at an increased risk for hospital readmission, when compared with those patients who are not using steroids and are most likely to be readmitted for an infectious complication. Schairer and colleagues33 assessed readmission rates after THA and found 30- and 90-day readmission rate of 4% and 7%, respectively. These authors also found that medical complications accounted for approximately 25% of readmissions, and hip-related complications (eg, dislocation, SSI) accounted for >50%. In our study, we found a 30-day readmission rate in non-steroid users of 3.53% and a rate of 6.52% in chronic steroid users. More than 30% of patients using a steroid were readmitted for infectious complications. As THA is becoming increasingly reimbursed under a bundled payments model by Medicare and Medicaid,34-36 reducing short-term readmissions is imperative. Therefore, discharge counseling that emphasizes how to recognize both the signs and symptoms of infection as well as how to prevent infections, such as reducing SSIs through appropriate wound care, may be warranted in higher risk chronic steroid users.
This study has a number of limitations that are inherent to ACS-NSQIP. First, we lacked specific information on a patient’s steroid history, including which corticosteroid they were using, dosage, frequency, and the indication for corticosteroid therapy. Therefore, we were unable to establish a dose-dependent relationship between steroid exposure and postoperative complications after THA. Second, we were able to assess only 30-day rates of complications and readmissions, and therefore, we were unable to identify intermediate- and long-term effects of systemic corticosteroid use on THA. Finally, we could not determine orthopedic- or hip-specific postoperative outcomes, such as functional scores and range of motion.
Continue to: CONCLUSION
CONCLUSION
In conclusion, this study quantified the increased risk for perioperative complications and hospital readmissions in patients who chronically use corticosteroids and are undergoing THA, when compared with those who do not use corticosteroids. These results suggest that patients who are on long-term steroids are at an increased risk for complications, primarily infectious complications. This finding has important implications for patient counseling, preoperative risk stratification, and suggests that higher risk patients, such as chronic steroid users, may benefit from improved discharge care to decrease complication rates.
1. Normansell R, Kew KM, Mansour G. Different oral corticosteroid regimens for acute asthma. Cochrane Database Syst Rev. 2016;13(5):CD011801. doi: 10.1002/14651858.CD011801.pub2.
2. Walters JA, Tan DJ, White CJ, Wood-Baker R. Different durations of corticosteroid therapy for exacerbations of chronic obstructive pulmonary disease. Cochrane Database Syst Rev. 2014;(12):CD006897.
3. Nunes T, Barreiro-de Acosta M, Marin-Jimenez I, Nos P, Sans M. Oral locally active steroids in inflammatory bowel disease. J Crohns Colitis. 2013;7(3):183-191. doi: 10.1016/j.crohns.2012.06.010.
4. Karatzanis A, Chatzidakis A, Milioni A, Vlaminck S, Kawauchi H, Velegrakis S, et al. Contemporary use of corticosteroids in rhinology. Curr Allergy Asthm R. 2017;17(2). doi: 10.1007/s11882-017-0679-0.
5. Parker BJ, Bruce IN. High dose methylprednisolone therapy for the treatment of severe systemic lupus erythematosus. Lupus. 2007;16(6):387-393. doi: 10.1177/0961203307079502.
6. Ferreira JF, Ahmed Mohamed AA, Emery P. Glucocorticoids and rheumatoid arthritis. Rheum Dis Clin North Am. 2016;42(1):33-46. doi: 10.1016/j.rdc.2015.08.006.
7. Buttgereit F, Dejaco C, Matteson EL, Dasgupta B. Polymyalgia rheumatica and giant cell arteritis: a systematic review. JAMA. 2016;315(22):2442-2458. doi: 10.1001/jama.2016.5444.
8. Overman RA, Yeh JY, Deal CL. Prevalence of oral glucocorticoid usage in the United States: a general population perspective. Arthritis Care Res. 2013;65(2):294-298. doi: 10.1002/acr.21796.
9. Fardet L, Petersen I, Nazareth I. Prevalence of long-term oral glucocorticoid prescriptions in the UK over the past 20 years. Rheumatology. 2011;50(11):1982-1990. doi: 10.1093/rheumatology/ker017.
10. Canalis E, Mazziotti G, Giustina A, Bilezikian JP. Glucocorticoid-induced osteoporosis: pathophysiology and therapy.Osteoporos Int. 2007;18(10):1319-1328. doi: 10.1007/s00198-007-0394-0.
11. Kanis JA, Johansson H, Oden A, Johnell O, de Laet C, Melton LJ, et al. A meta-analysis of prior corticosteroid use and fracture risk. J Bone Miner Res. 2004;19(6):893-899. doi: /10.1359/JBMR.040134.
12. Caplan A, Fett N, Rosenbach M, Werth VP, Micheletti RG. Prevention and management of glucocorticoid-induced side effects: a comprehensive review: a review of glucocorticoid pharmacology and bone health. J Am Acad Dermatol. 2017;76(1):1-9. doi: 10.1016/j.jaad.2016.01.062.
13. Cutolo M, Seriolo B, Pizzorni C, Secchi ME, Soldano S, Paolino S, et al. Use of glucocorticoids and risk of infections. Autoimmun Rev. 2008;8(2):153-155. doi: 10.1016/j.autrev.2008.07.010.
14. Blackwood LL, Pennington JE. Dose-dependent effect of glucocorticosteroids on pulmonary defenses in a steroid-resistant host. Am Rev Respir Dis. 1982;126(6):1045-1049.
15. Toruner M, Loftus EV, Jr., Harmsen WS, Zinsmeister AR, Orenstein R, Sandborn WJ, et al. Risk factors for opportunistic infections in patients with inflammatory bowel disease. Gastroenterology. 2008;134(4):929-936. doi: 10.1053/j.gastro.2008.01.012.
16. Barratt PA, Brookes N, Newson A. Conservative treatments for greater trochanteric pain syndrome: a systematic review. Br J Sports Med. 2017;51(2):97-104. doi: 10.1136/bjsports-2015-095858.
17. Pereira LC, Kerr J, Jolles BM. Intra-articular steroid injection for osteoarthritis of the hip prior to total hip arthroplasty: is it safe? a systematic review. Bone Joint J. 2016;98-B(8):1027-1035. doi: 10.1302/0301-620X.98B8.37420.
18. Ravi B, Escott B, Shah PS, Jenkinson R, Chahal J, Bogoch E, et al. A systematic review and meta-analysis comparing complications following total joint arthroplasty for rheumatoid arthritis versus for osteoarthritis. Arthritis Rheum. 2012;64(12):3839-3849. doi: 10.1002/art.37690.
19. Ravi B, Croxford R, Hollands S, Paterson JM, Bogoch E, Kreder H, et al. Increased risk of complications following total joint arthroplasty in patients with rheumatoid arthritis. Arthritis Rheumatol. 2014;66(2):254-263. doi: 10.1002/art.38231.
20. ACS NSQIP Participant Use Data Files. https://www.facs.org/quality-programs/acs-nsqip/program-specifics/participant-use. Accessed December 6, 2018.
21. Lawson EH, Louie R, Zingmond DS, Brook RH, Hall BL, Han L, et al. A comparison of clinical registry versus administrative claims data for reporting of 30-day surgical complications. Ann Surg. 2012;256(6):973-981. doi: 10.1097/SLA.0b013e31826b4c4f.
22. Weiss A, Anderson JE, Chang DC. Comparing the national surgical quality improvement program with the nationwide inpatient sample database. JAMA Surg. 2015;150(8):815-816. doi: 10.1001/jamasurg.2015.0962.
23. Boddapati V, Fu MC, Mayman DJ, Su EP, Sculco PK, McLawhorn AS. Revision total knee arthroplasty for periprosthetic joint infection is associated with increased postoperative morbidity and mortality relative to noninfectious revisions. J Arthroplasty. 2018;33(2):521-526. doi: 10.1016/j.arth.2017.09.021.
24. Boddapati V, Fu MC, Schairer WW, Gulotta LV, Dines DM, Dines JS. Revision total shoulder arthroplasty is associated with increased thirty-day postoperative complications and wound infections relative to primary total shoulder arthroplasty. HSS J. 2018;14(1):23-28. doi: 10.1007/s11420-017-9573-5.
25. Boddapati V, Fu MC, Schiarer WW, Ranawat AS, Dines DM, Taylor SA, Dines DM. Increased shoulder arthroscopy time is associated with overnight hospital stay and surgical site infection. Arthroscopy. 2018;34(2):363-368. doi: 10.1016/j.arthro.2017.08.243.
26. Lunt M. Selecting an appropriate caliper can be essential for achieving good balance with propensity score matching. Am J Epidemiol. 2014 Jan 15;179(2):226-235. doi: 10.1093/aje/kwt212.
27. Tannenbaum DA, Matthews LS, Grady-Benson JC. Infection around joint replacements in patients who have a renal or liver transplantation. J Bone Joint Surg Am. 1997;79(1):36-43.
28. Shrader MW, Schall D, Parvizi J, McCarthy JT, Lewallen DG. Total hip arthroplasty in patients with renal failure: a comparison between transplant and dialysis patients. J Arthroplasty. 2006;21(3):324-329. doi: 10.1016/j.arth.2005.07.008.
29. Nowicki P, Chaudhary H. Total hip replacement in renal transplant patients. J Bone Joint Surg Br. 2007;89(12):1561-1566.
30. Johannesdottir SA, Horváth-Puhó E, Dekkers OM, Cannegieter SC, Jørgensen JO, Ehrenstein V, et al. Use of glucocorticoids and risk of venous thromboembolism: a nationwide population-based case-control study. JAMA Intern Med. 2013;173(9):743-752. doi: 10.1001/jamainternmed.2013.122.
31. Hartman J, Khanna V, Habib A, Farrokhyar F, Memon M, Adili A. Perioperative systemic glucocorticoids in total hip and knee arthroplasty: a systematic review of outcomes. J Orthop. 2017;14(2):294-301. doi: 10.1016/j.jor.2017.03.012.
32. Sculco PK, McLawhorn AS, Desai N, Su EP, Padgett DE, Jules-Elysee K. The effect of perioperative corticosteroids in total hip arthroplasty: a prospective double-blind placebo controlled pilot study. J Arthroplasty. 2016;31(6):1208-1212. doi: 10.1016/j.arth.2015.11.011.
33. Schairer WW, Sing DC, Vail TP, Bozic KJ. Causes and frequency of unplanned hospital readmission after total hip arthroplasty. Clin Orthop Relat Res. 2014;472(2):464-470. doi: 10.1007/s11999-013-3121-5.
34. US Department of Health and Human Services. Comprehensive Care for Joint Replacement Model. Centers for Medicare & Medicaid Services. https://innovation.cms.gov/initiatives/cjr. Accessed June 15, 2017.
35. Bozic KJ, Ward L, Vail TP, Maze M. Bundled payments in total joint arthroplasty: targeting opportunities for quality improvement and cost reduction. Clin Orthop Relat Res. 2014;472(1):188-193. doi: 10.1007/s11999-013-3034-3.
36. Bosco JA, 3rd, Karkenny AJ, Hutzler LH, Slover JD, Iorio R. Cost burden of 30-day readmissions following Medicare total hip and knee arthroplasty. J Arthroplasty. 2014;29(5): 903-905. doi: 10.1016/j.arth.2013.11.006.
1. Normansell R, Kew KM, Mansour G. Different oral corticosteroid regimens for acute asthma. Cochrane Database Syst Rev. 2016;13(5):CD011801. doi: 10.1002/14651858.CD011801.pub2.
2. Walters JA, Tan DJ, White CJ, Wood-Baker R. Different durations of corticosteroid therapy for exacerbations of chronic obstructive pulmonary disease. Cochrane Database Syst Rev. 2014;(12):CD006897.
3. Nunes T, Barreiro-de Acosta M, Marin-Jimenez I, Nos P, Sans M. Oral locally active steroids in inflammatory bowel disease. J Crohns Colitis. 2013;7(3):183-191. doi: 10.1016/j.crohns.2012.06.010.
4. Karatzanis A, Chatzidakis A, Milioni A, Vlaminck S, Kawauchi H, Velegrakis S, et al. Contemporary use of corticosteroids in rhinology. Curr Allergy Asthm R. 2017;17(2). doi: 10.1007/s11882-017-0679-0.
5. Parker BJ, Bruce IN. High dose methylprednisolone therapy for the treatment of severe systemic lupus erythematosus. Lupus. 2007;16(6):387-393. doi: 10.1177/0961203307079502.
6. Ferreira JF, Ahmed Mohamed AA, Emery P. Glucocorticoids and rheumatoid arthritis. Rheum Dis Clin North Am. 2016;42(1):33-46. doi: 10.1016/j.rdc.2015.08.006.
7. Buttgereit F, Dejaco C, Matteson EL, Dasgupta B. Polymyalgia rheumatica and giant cell arteritis: a systematic review. JAMA. 2016;315(22):2442-2458. doi: 10.1001/jama.2016.5444.
8. Overman RA, Yeh JY, Deal CL. Prevalence of oral glucocorticoid usage in the United States: a general population perspective. Arthritis Care Res. 2013;65(2):294-298. doi: 10.1002/acr.21796.
9. Fardet L, Petersen I, Nazareth I. Prevalence of long-term oral glucocorticoid prescriptions in the UK over the past 20 years. Rheumatology. 2011;50(11):1982-1990. doi: 10.1093/rheumatology/ker017.
10. Canalis E, Mazziotti G, Giustina A, Bilezikian JP. Glucocorticoid-induced osteoporosis: pathophysiology and therapy.Osteoporos Int. 2007;18(10):1319-1328. doi: 10.1007/s00198-007-0394-0.
11. Kanis JA, Johansson H, Oden A, Johnell O, de Laet C, Melton LJ, et al. A meta-analysis of prior corticosteroid use and fracture risk. J Bone Miner Res. 2004;19(6):893-899. doi: /10.1359/JBMR.040134.
12. Caplan A, Fett N, Rosenbach M, Werth VP, Micheletti RG. Prevention and management of glucocorticoid-induced side effects: a comprehensive review: a review of glucocorticoid pharmacology and bone health. J Am Acad Dermatol. 2017;76(1):1-9. doi: 10.1016/j.jaad.2016.01.062.
13. Cutolo M, Seriolo B, Pizzorni C, Secchi ME, Soldano S, Paolino S, et al. Use of glucocorticoids and risk of infections. Autoimmun Rev. 2008;8(2):153-155. doi: 10.1016/j.autrev.2008.07.010.
14. Blackwood LL, Pennington JE. Dose-dependent effect of glucocorticosteroids on pulmonary defenses in a steroid-resistant host. Am Rev Respir Dis. 1982;126(6):1045-1049.
15. Toruner M, Loftus EV, Jr., Harmsen WS, Zinsmeister AR, Orenstein R, Sandborn WJ, et al. Risk factors for opportunistic infections in patients with inflammatory bowel disease. Gastroenterology. 2008;134(4):929-936. doi: 10.1053/j.gastro.2008.01.012.
16. Barratt PA, Brookes N, Newson A. Conservative treatments for greater trochanteric pain syndrome: a systematic review. Br J Sports Med. 2017;51(2):97-104. doi: 10.1136/bjsports-2015-095858.
17. Pereira LC, Kerr J, Jolles BM. Intra-articular steroid injection for osteoarthritis of the hip prior to total hip arthroplasty: is it safe? a systematic review. Bone Joint J. 2016;98-B(8):1027-1035. doi: 10.1302/0301-620X.98B8.37420.
18. Ravi B, Escott B, Shah PS, Jenkinson R, Chahal J, Bogoch E, et al. A systematic review and meta-analysis comparing complications following total joint arthroplasty for rheumatoid arthritis versus for osteoarthritis. Arthritis Rheum. 2012;64(12):3839-3849. doi: 10.1002/art.37690.
19. Ravi B, Croxford R, Hollands S, Paterson JM, Bogoch E, Kreder H, et al. Increased risk of complications following total joint arthroplasty in patients with rheumatoid arthritis. Arthritis Rheumatol. 2014;66(2):254-263. doi: 10.1002/art.38231.
20. ACS NSQIP Participant Use Data Files. https://www.facs.org/quality-programs/acs-nsqip/program-specifics/participant-use. Accessed December 6, 2018.
21. Lawson EH, Louie R, Zingmond DS, Brook RH, Hall BL, Han L, et al. A comparison of clinical registry versus administrative claims data for reporting of 30-day surgical complications. Ann Surg. 2012;256(6):973-981. doi: 10.1097/SLA.0b013e31826b4c4f.
22. Weiss A, Anderson JE, Chang DC. Comparing the national surgical quality improvement program with the nationwide inpatient sample database. JAMA Surg. 2015;150(8):815-816. doi: 10.1001/jamasurg.2015.0962.
23. Boddapati V, Fu MC, Mayman DJ, Su EP, Sculco PK, McLawhorn AS. Revision total knee arthroplasty for periprosthetic joint infection is associated with increased postoperative morbidity and mortality relative to noninfectious revisions. J Arthroplasty. 2018;33(2):521-526. doi: 10.1016/j.arth.2017.09.021.
24. Boddapati V, Fu MC, Schairer WW, Gulotta LV, Dines DM, Dines JS. Revision total shoulder arthroplasty is associated with increased thirty-day postoperative complications and wound infections relative to primary total shoulder arthroplasty. HSS J. 2018;14(1):23-28. doi: 10.1007/s11420-017-9573-5.
25. Boddapati V, Fu MC, Schiarer WW, Ranawat AS, Dines DM, Taylor SA, Dines DM. Increased shoulder arthroscopy time is associated with overnight hospital stay and surgical site infection. Arthroscopy. 2018;34(2):363-368. doi: 10.1016/j.arthro.2017.08.243.
26. Lunt M. Selecting an appropriate caliper can be essential for achieving good balance with propensity score matching. Am J Epidemiol. 2014 Jan 15;179(2):226-235. doi: 10.1093/aje/kwt212.
27. Tannenbaum DA, Matthews LS, Grady-Benson JC. Infection around joint replacements in patients who have a renal or liver transplantation. J Bone Joint Surg Am. 1997;79(1):36-43.
28. Shrader MW, Schall D, Parvizi J, McCarthy JT, Lewallen DG. Total hip arthroplasty in patients with renal failure: a comparison between transplant and dialysis patients. J Arthroplasty. 2006;21(3):324-329. doi: 10.1016/j.arth.2005.07.008.
29. Nowicki P, Chaudhary H. Total hip replacement in renal transplant patients. J Bone Joint Surg Br. 2007;89(12):1561-1566.
30. Johannesdottir SA, Horváth-Puhó E, Dekkers OM, Cannegieter SC, Jørgensen JO, Ehrenstein V, et al. Use of glucocorticoids and risk of venous thromboembolism: a nationwide population-based case-control study. JAMA Intern Med. 2013;173(9):743-752. doi: 10.1001/jamainternmed.2013.122.
31. Hartman J, Khanna V, Habib A, Farrokhyar F, Memon M, Adili A. Perioperative systemic glucocorticoids in total hip and knee arthroplasty: a systematic review of outcomes. J Orthop. 2017;14(2):294-301. doi: 10.1016/j.jor.2017.03.012.
32. Sculco PK, McLawhorn AS, Desai N, Su EP, Padgett DE, Jules-Elysee K. The effect of perioperative corticosteroids in total hip arthroplasty: a prospective double-blind placebo controlled pilot study. J Arthroplasty. 2016;31(6):1208-1212. doi: 10.1016/j.arth.2015.11.011.
33. Schairer WW, Sing DC, Vail TP, Bozic KJ. Causes and frequency of unplanned hospital readmission after total hip arthroplasty. Clin Orthop Relat Res. 2014;472(2):464-470. doi: 10.1007/s11999-013-3121-5.
34. US Department of Health and Human Services. Comprehensive Care for Joint Replacement Model. Centers for Medicare & Medicaid Services. https://innovation.cms.gov/initiatives/cjr. Accessed June 15, 2017.
35. Bozic KJ, Ward L, Vail TP, Maze M. Bundled payments in total joint arthroplasty: targeting opportunities for quality improvement and cost reduction. Clin Orthop Relat Res. 2014;472(1):188-193. doi: 10.1007/s11999-013-3034-3.
36. Bosco JA, 3rd, Karkenny AJ, Hutzler LH, Slover JD, Iorio R. Cost burden of 30-day readmissions following Medicare total hip and knee arthroplasty. J Arthroplasty. 2014;29(5): 903-905. doi: 10.1016/j.arth.2013.11.006.
TAKE-HOME POINTS
- The rate of preoperative corticosteroid usage is low (3.7%).
- Patients using preoperative corticosteroids had increased rates of total 30-day complications.
- Adverse outcomes that are increased include infectious complications (eg, sepsis, urinary tract infection, surgical site infection).
- Hospital readmissions are also increased in patients taking preoperative corticosteroids, with the most common reason being infection.
- Increased postoperative counseling and surveillance may be warranted in this patient population.