User login
Effect of Alirocumab Monotherapy vs Ezetimibe Plus Statin Therapy on LDL-C Lowering in Veterans With History of ASCVD
Atherosclerotic cardiovascular disease (ASCVD) is a significant cause of morbidity and mortality in the United States. ASCVD involves the buildup of cholesterol plaque in arteries and includes acute coronary syndrome, peripheral arterial disease, and events such as myocardial infarction and stroke.1 Cardiovascular disease (CVD) risk factors include high cholesterol levels, elevated blood pressure, insulin resistance, elevated blood glucose levels, smoking, poor dietary habits, and a sedentary lifestyle.2
According to the Centers for Disease Control and Prevention, about 86 million adults aged ≥ 20 years have total cholesterol levels > 200 mg/dL. More than half (54.5%) who could benefit are currently taking cholesterol-lowering medications.3 Controlling high cholesterol in American adults, especially veterans, is essential for reducing CVD morbidity and mortality.
The 2018 American College of Cardiology/American Heart Association (ACC/AHA) guideline recommends a low-density lipoprotein cholesterol (LDL-C) target goal of < 70 mg/dL for patients at high risk for ASCVD. Very high-risk ASCVD includes a history of multiple major ASCVD events or 1 major ASCVD event and multiple high-risk conditions (eg, age ≥ 65 years, smoking, or diabetes).4 Major ASCVD events include recent acute coronary syndrome (within the past 12 months), a history of myocardial infarction or ischemic stroke, and symptomatic peripheral artery disease.
The ACC/AHA guideline suggests that if the LDL-C level remains ≥ 70 mg/dL, adding ezetimibe (a dietary cholesterol absorption inhibitor) to maximally tolerated statin therapy is reasonable. If LDL-C levels remain ≥ 70 mg/dL, adding a proprotein convertase subtilisin/kexin type 9 (PCSK9) inhibitor, such as alirocumab, is reasonable.4 The US Departments of Veterans Affairs/US Department of Defense guidelines recommend using maximally tolerated statins and ezetimibe before PCSK9 inhibitors due to established long-term safety and reduction in CVD events.
Generic statins and ezetimibe are administered orally and widely available. In contrast, PCSK9 inhibitors have unknown long-term safety profiles, require subcutaneous injection once or twice monthly, and are significantly more expensive. They also require patient education on proper use while providing comparable or lesser relative risk reductions.2
These 3 classes of medication vary in their mechanisms of action to reduce LDL.5,6 Ezetimibe and several statin medications are included on the Veterans Affairs Sioux Falls Health Care System (VASFHCS) formulary and do not require review prior to prescribing. Alirocumab is available at VASFHCS but is restricted to patients with a history of ASCVD or a diagnosis of familial hypercholesterolemia, and who are receiving maximally tolerated statin and ezetimibe therapy but require further LDL-C lowering to reduce their ASCVD risk.
Studies have found ezetimibe monotherapy reduces LDL-C in patients with dyslipidemia by 18% after 12 weeks.7 One found that the percentage reduction in LDL-C was significantly greater (P < .001) with all doses of ezetimibe plus simvastatin (46% to 59%) compared with either atorvastatin 10 mg (37%) or simvastatin 20 mg (38%) monotherapy after 6 weeks.8
Although alirocumab can be added to other lipid therapies, most VASFHCS patients are prescribed alirocumab monotherapy. In the ODYSSEY CHOICE II study, patients were randomly assigned to receive either a placebo or alirocumab 150 mg every 4 weeks or alirocumab 75 mg every 2 weeks. The primary efficacy endpoint was LDL-C percentage change from baseline to week 24. In the alirocumab 150 mg every 4 weeks and 75 mg every 2 weeks groups, the least-squares mean LDL-C changes from baseline to week 24 were 51.7% and 53.5%, respectively, compared to a 4.7% increase in the placebo group (both groups P < .001 vs placebo). The authors also reported that alirocumab 150 mg every 4 weeks as monotherapy demonstrated a 47.4% reduction in LDL-C levels from baseline in a phase 1 study.9Although alirocumab monotherapy and ezetimibe plus statin therapy have been shown to effectively decrease LDL-C independently, a direct comparison of alirocumab monotherapy vs ezetimibe plus statin therapy has not been assessed, to our knowledge. Understanding the differences in effectiveness and safety between these 2 regimens will be valuable for clinicians when selecting a medication regimen for veterans with a history of ASCVD.
METHODS
This retrospective, single-center chart review used VASFHCS Computerized Patient Record System (CPRS) and Joint Longitudinal Viewer (JLV) records to compare patients with a history of ASCVD events who were treated with alirocumab monotherapy or ezetimibe plus statin. The 2 groups were randomized in a 1:3 ratio. The primary endpoint was achieving LDL-C < 70 mg/dL after 4 to 12 weeks, 13 to 24 weeks, and 25 to 52 weeks. Secondary endpoints included the mean percentage change from baseline in total cholesterol (TC), high-density lipoprotein cholesterol (HDL-C), LDL-C, and triglycerides (TG) over 52 weeks. The incidence of ASCVD events during this period was also assessed. If LDL-C < 70 mg/dL was achieved > 1 time during each time frame, only 1 incident was counted for analysis. Safety was assessed based on the incidence of any adverse event (AE) that led to treatment discontinuation.
Patients were identified by screening the prescription fill history between October 1, 2019, and December 31, 2022. The 52-week data collection period was counted from the first available fill date. Additionally, the prior authorization drug request file from January 1, 2017, to December 31, 2022, was used to obtain a list of patients prescribed alirocumab. Patients were included if they were veterans aged ≥ 18 years and had a history of an ASCVD event, had a alirocumab monotherapy or ezetimibe plus statin prescription between October 1, 2019, and December 31, 2022, or had an approved prior authorization drug request for alirocumab between January 1, 2017, and December 31, 2022. Patients missing a baseline or follow-up lipid panel and those with concurrent use of alirocumab and ezetimibe and/or statin were excluded.
Baseline characteristics collected for patients included age, sex, race, weight, body mass index, lipid parameters (LDL-C, TC, HDL-C, and TG), dosing of each type of statin before adding ezetimibe, and use of any other antihyperlipidemic medication. We also collected histories of hypertension, hyperlipidemia, diabetes, chronic kidney disease, congestive heart failure, and smoking or tobacco use status. The baseline lipid panel was the most recent lipid panel documented before starting alirocumab or ezetimibe plus statin therapy. Follow-up lipid panel values were gathered at 4 to 12 weeks, 13 to 24 weeks, and 25 to 52 weeks following initiation of either therapy.
High-, moderate-, and low-intensity dosing of statin therapy and alirocumab dosing (75 mg every 2 weeks, 150 mg every 2 weeks, or 300 mg every 4 weeks) were recorded at the specified intervals. However, no patients in this study received the latter dosing regimen. ASCVD events and safety endpoints were recorded based on a review of clinical notes over the 52 weeks following the first available start date.
Statistical Analysis
The primary endpoint of achieving the LDL-C < 70 mg/dL goal from baseline to 4 to 12 weeks, 13 to 24 weeks, and 25 to 52 weeks after initiation was compared between alirocumab monotherapy and ezetimibe plus statin therapy using the χ² test. Mean percentage change from baseline in TC, HDL-C, LDL-C, and TG were compared using the independent t test. P < .05 was considered statistically significant. Incidence of ASCVD events and the safety endpoint (incidence of AEs leading to treatment discontinuation) were also compared using the χ² test. Continuous baseline characteristics were reported mean (SD) and nominal baseline characteristics were reported as a percentage.
RESULTS
There were 80 participants in this study: 20 in the alirocumab monotherapy group and 60 in the ezetimibe plus statin therapy group. More than 100 patients did not meet the prespecified inclusion criteria and were excluded. Mean (SD) age was 75 (8) years in the alirocumab group and 74 (8) years in the ezetimibe plus statin group. There was no significant differences in mean (SD) weight or mean (SD) body mass index. All study participants identified as White and male except for 2 patients in the ezetimibe plus statin therapy group whose race was not documented. Differences in lipid parameters were observed between groups, with mean baseline LDL-C, HDL-C, and TC higher in the alirocumab monotherapy group than in the ezetimibe plus statin therapy group, with significant differences in LDL-C and TC (Table 1).
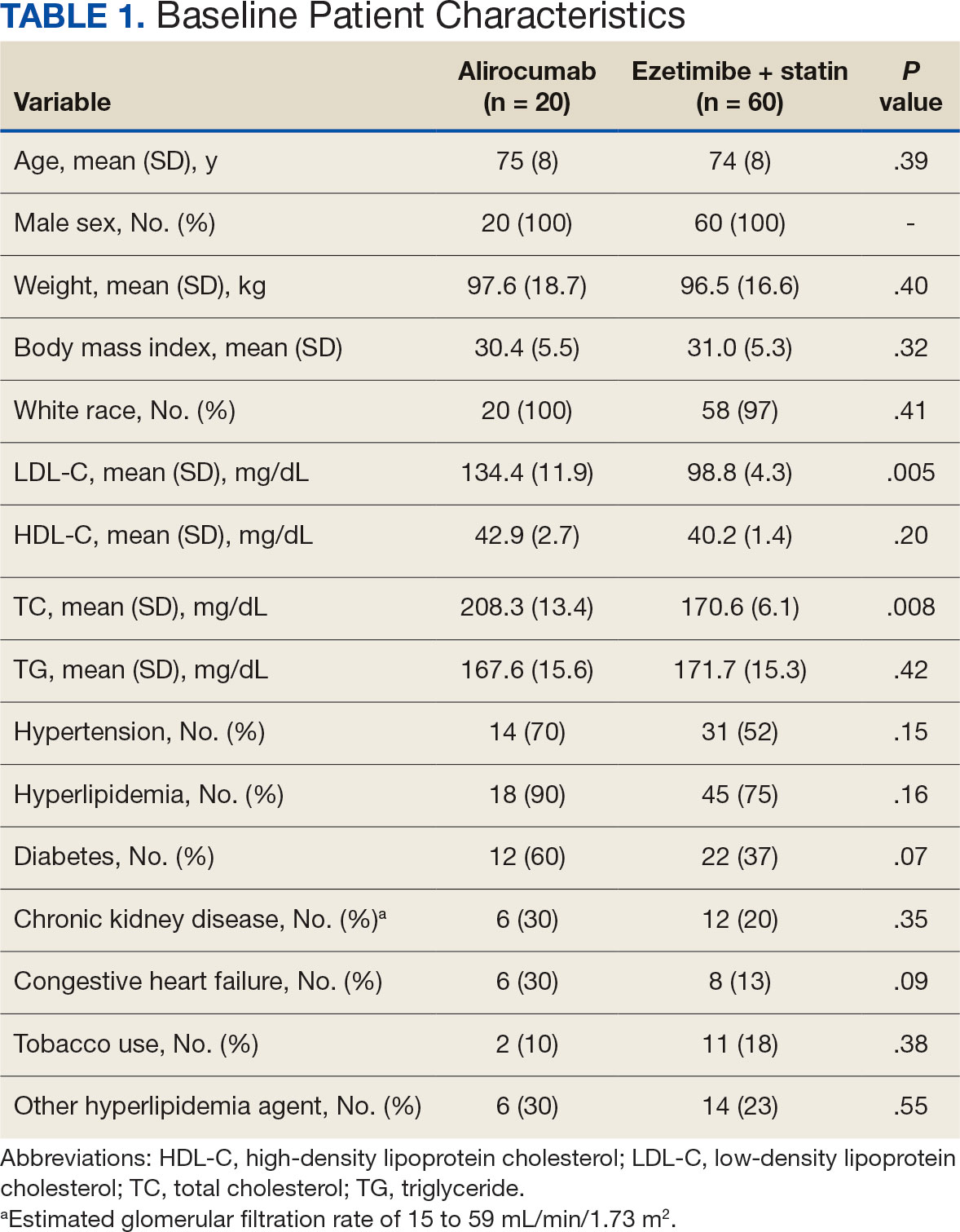
Fourteen patients (70%) in the alirocumab monotherapy group had hypertension, compared with 31 (52%) in the ezetimibe plus statin therapy group. In both groups, most patients had previously been diagnosed with hyperlipidemia. More patients (60%) in the alirocumab group had diabetes than in the ezetimibe plus statin therapy group (37%). The alirocumab monotherapy group also had a higher percentage of patients with diagnoses of congestive heart failure and used other antihyperlipidemic medications than in the ezetimibe plus statin therapy group. Five patients (25%) in the alirocumab monotherapy group and 12 patients (20%) in the ezetimibe plus statin therapy group took fish oil. In the ezetimibe plus statin therapy group, 2 patients (3%) took gemfibrozil, and 2 patients (3%) took fenofibrate. Six (30%) patients in the alirocumab monotherapy group and 12 (20%) patients in the ezetimibe plus statin therapy group had chronic kidney disease. Although the majority of patients in each group did not use tobacco products, there were more tobacco users in the ezetimibe plus statin therapy group.
In the alirocumab monotherapy group, 15 patients (75%) were prescribed 75 mg every 2 weeks and 5 patients (25%) were prescribed 150 mg every 2 weeks. In the ezetimibe plus statin therapy group, 59 patients (98%) were prescribed ezetimibe 10 mg/d (Table 2). Forty-three patients (72%) were prescribed a high-intensity statin 10 received moderate-intensity (17%) and 7 received low-intensity statin (12%). Most patients were prescribed rosuvastatin (45%), followed by atorvastatin (42%), pravastatin (10%), and simvastatin (3%).
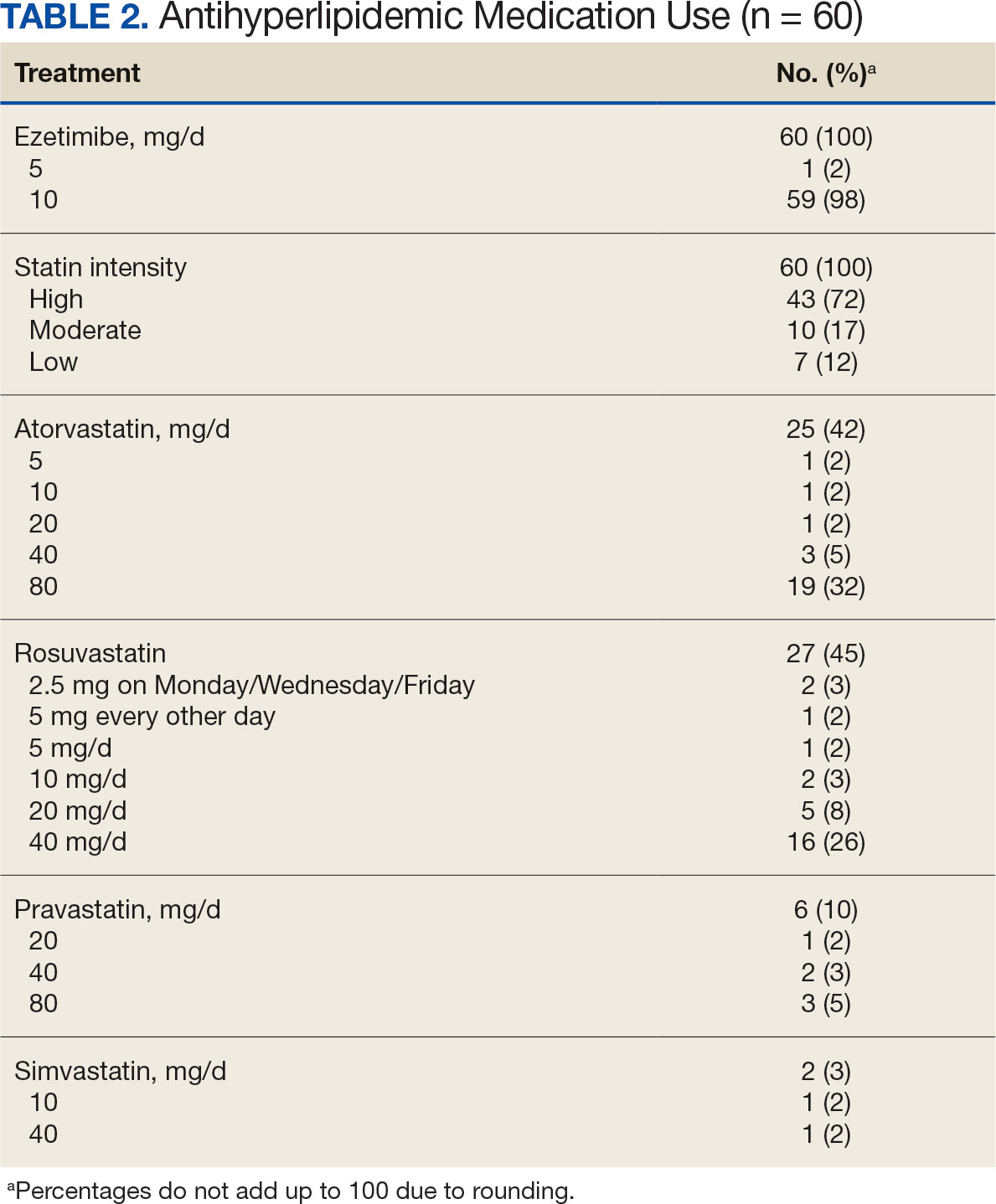
Primary Endpoint
During the 52-week study, more patients met the LDL-C goal of < 70 mg/dL in the alirocumab monotherapy group (70%) than in the ezetimibe plus statin therapy group (57%); however, the difference was not significant (P = .29). Of the patients prescribed alirocumab monotherapy who achieved LDL-C < 70 mg/dL, 15% achieved this goal in 4 to 12 weeks, 40% in 13 to 24 weeks, and 45% in 25 to 52 weeks. In the ezetimibe plus statin therapy group, 28% of patients achieved LDL-C < 70 mg/dL in 4 to 12 weeks, 31% in 13 to 24 weeks, and 41% in 25 to 52 weeks (Table 3).
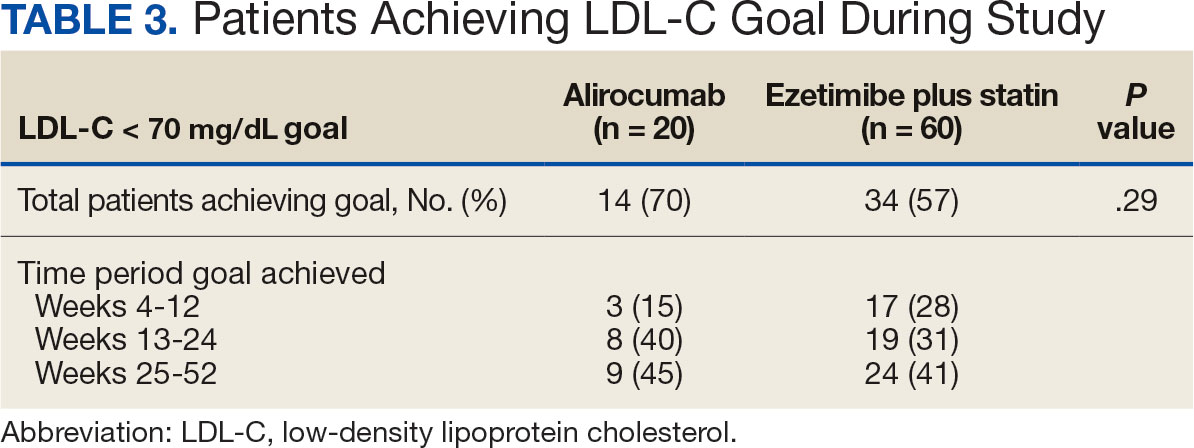
Secondary Endpoints
During weeks 4 to 52 of treatment, the mean percentage change decreased in LDL-C (37.7% vs 21.4%; P = .01), TC (24.7% vs 12.5%; P = .01), and TG (0.9% vs 7.0%; P = .28) in the alirocumab monotherapy group and the ezetimibe plus statin therapy group, respectively (Table 4). The mean percentage change increased in HDL-C by 3.6% in the alirocumab monotherapy group and 1.8% in the ezetimibe plus statin therapy group (P = .36). During the study, ASCVD events occurred in 1 patient (5%) in the alirocumab monotherapy group and 3 patients (5%) in the ezetimibe plus statin therapy group (P = .99). The patient in the alirocumab monotherapy group had unstable angina 1 month after taking alirocumab. One patient in the ezetimibe plus statin therapy group had coronary artery disease and 2 patients had coronary heart disease that required stents during the 52-week period. There was 1 patient in each group who reported an AE that led to treatment discontinuation (P = .41). One patient stopped alirocumab after a trial of 2 months due to intolerance, but no specific AE was reported in the CPRS. In the ezetimibe plus statin therapy group, 1 patient requested to discontinue ezetimibe after a trial of 3 months without a specific reason noted in the medical record.
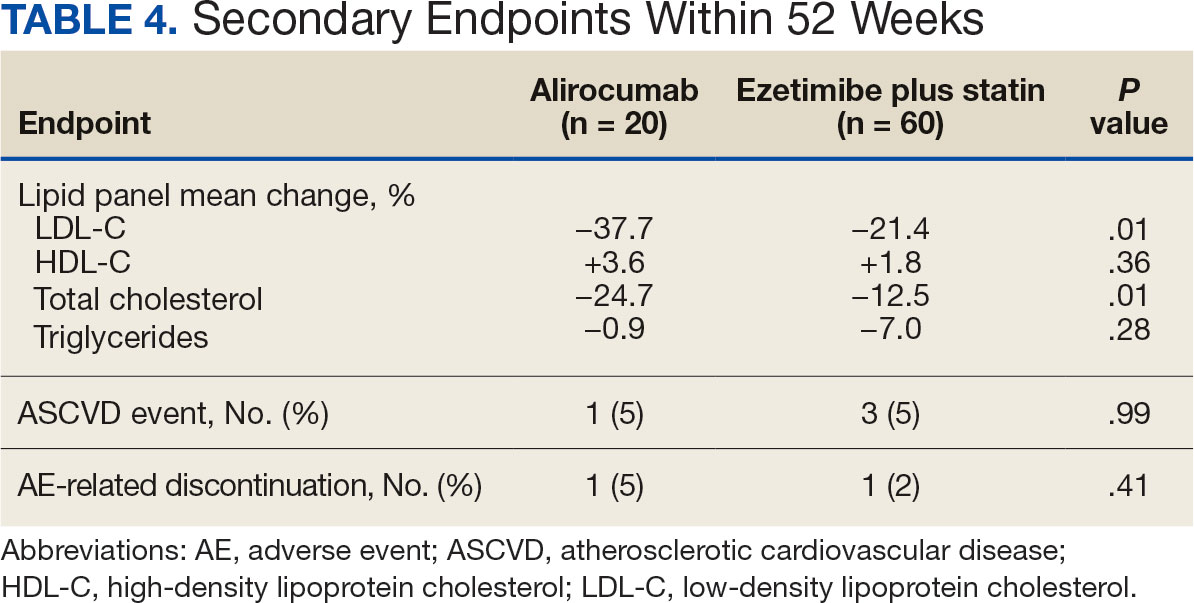
DISCUSSION
This study found no statistically significant difference in the incidence of reaching an LDL-C goal of < 70 mg/dL after alirocumab monotherapy initiation compared with ezetimibe plus statin therapy. This occurred despite baseline LDL-C being lower in the ezetimibe plus statin therapy group, which required a smaller reduction in LDL-C to reach the primary goal. Most patients on alirocumab monotherapy were prescribed a lower initial dose of 75 mg every 2 weeks. Of those patients, 30% did not achieve the LDL-C goal < 70 mg/dL. Thus, a higher dose may have led to more patients achieving the LDL-C goal.
Secondary endpoints, including mean percentage change in HDL-C and TG and incidence of ASCVD events during 52 weeks of treatment, were not statistically significant. The mean percentage increase in HDL-C was negligible in both groups, while the mean percentage reduction in TG favored the ezetimibe plus statin therapy group. In the ezetimibe plus statin therapy group, patients who also took fenofibrate experienced a significant reduction in TG while none of the patients in the alirocumab group were prescribed fenofibrate. Although the alirocumab monotherapy group had a statistically significant greater reduction in LDL-C and TC compared with those prescribed ezetimibe plus statin, the mean baseline LDL-C and TC were significantly greater in the alirocumab monotherapy group, which could contribute to higher reductions in LDL-C and TC after alirocumab monotherapy.Based on the available literature, we expected greater reductions in LDL-C in both study groups compared with statin therapy alone.8,9 However, it was unclear whether the LDL-C and TC reductions were clinically significant.
Limitations
The study design did not permit randomization prior to the treatments, restricting our ability to account for some confounding factors, such as diet, exercise, other antihyperlipidemic medication, and medication adherence, which may have affected LDL-C, HDL-C, TG, and TC levels. Differences in baseline characteristics—particularly major risk factors, such as hypertension, diabetes, and tobacco use—also could have confounding affect on lipid levels and ASCVD events. Additionally, patients prescribed alirocumab monotherapy may have switched from statin or ezetimibe therapy, and the washout period was not reviewed or recorded, which could have affected the lipid panel results.
The small sample size of this study also may have limited the ability to detect significant differences between groups. A direct comparison of alirocumab monotherapy vs ezetimibe plus statin therapy has not been performed, making it difficult to prospectively evaluate what sample size would be needed to power this study. A posthoc analysis was used to calculate power, which was found to be only 17%. Many patients were excluded due to a lack of laboratory results within the study period, contributing to the small sample size.
Another limitation was the reliance on documentation in CPRS and JLV. For example, having documentation of the specific AEs for the 2 patients who discontinued alirocumab or ezetimibe could have helped determine the severity of the AEs. Several patients were followed by non-VA clinicians, which could have contributed to limited documentation in the CPRS and JLV. It is difficult to draw any conclusions regarding ASCVD events and AEs that led to treatment discontinuation between alirocumab monotherapy and ezetimibe plus statin therapy based on the results of this retrospective study due to the limited number of events within the 52-week period.
CONCLUSIONS
This study found that there was no statistically significant difference in LDL-C reduction to < 70 mg/dL between alirocumab monotherapy and ezetimibe plus statin therapy in a small population of veterans with ASCVD, with a higher percentage of participants in both groups achieving that goal in 25 to 52 weeks. There also was no significant difference in percentage change in HDL-C or TG or in incidence of ASCVD events and AEs leading to treatment discontinuation. However, there was a statistically significant difference in percentage reduction for LDL-C and TC during 52 weeks of alirocumab monotherapy vs ezetimibe plus statin therapy.
Although there was no significant difference in LDL-C reduction to < 70 mg/dL, targeting this goal in patients with ASCVD is still clinically warranted. This study does not support a change in current VA criteria for use of alirocumab or a change in current guidelines for secondary prevention of ASCVD. Still, this study does indicate that the efficacy of alirocumab monotherapy is similar to that of ezetimibe plus statin therapy in patients with a history of ASCVD and may be useful in clinical settings when an alternative to ezetimibe plus statin therapy is needed. Alirocumab also may be more effective in lowering LDL-C and TC than ezetimibe plus statin therapy in veterans with ASCVD and could be added to statin therapy or ezetimibe when additional LDL-C or TC reduction is needed.
Lucchi T. Dyslipidemia and prevention of atherosclerotic cardiovascular disease in the elderly. Minerva Med. 2021;112:804-816. doi:10.23736/S0026-4806.21.07347-X
The Management of Dyslipidemia for Cardiovascular Risk Reduction Work Group. VA/DoD Clinical Practice Guideline for the Management of Dyslipidemia for Cardiovascular Risk Reduction. Version 4.0. June 2020. Accessed September 5, 2024. https://www.healthquality.va.gov/guidelines/CD/lipids/VADoDDyslipidemiaCPG5087212020.pdf
Centers for Disease Control and Prevention. High Cholesterol Facts. May 15, 2024. Accessed October 3, 2024. https://www.cdc.gov/cholesterol/data-research/facts-stats/index.html
Grundy SM, Stone NJ, Bailey AL, et al. 2018 AHA/ACC/AACVPR/AAPA/ABC/ACPM/ADA/AGS/APhA/ASPC/NLA/PCNA guideline on the management of blood cholesterol: a report of the American College of Cardiology/American Heart Association Task Force on Clinical Practice Guidelines. Circulation. 2019;139:e1082-e1143. doi:10.1161/CIR.0000000000000625
Vavlukis M, Vavlukis A. Statins alone or in combination with ezetimibe or PCSK9 inhibitors in atherosclerotic cardiovascular disease protection. IntechOpen. January 24, 2019. doi:10.5772/intechopen.82520
Alirocumab. Prescribing information. Regeneron Pharmaceuticals, Inc.; 2024. Accessed September 5, 2024. https://www.regeneron.com/downloads/praluent_pi.pdf
Pandor A, Ara RM, Tumur I, et al. Ezetimibe monotherapy for cholesterol lowering in 2,722 people: systematic review and meta-analysis of randomized controlled trials. J Intern Med. 2009;265(5):568-580. doi:10.1111/j.1365-2796.2008.02062.x
McKenney J, Ballantyne CM, Feldman TA, et al. LDL-C goal attainment with ezetimibe plus simvastatin coadministration vs atorvastatin or simvastatin monotherapy in patients at high risk of CHD. MedGenMed. 2005;7(3):3.
Stroes E, Guyton JR, Lepor N, et al. Efficacy and safety of alirocumab 150 mg every 4 weeks in patients with hypercholesterolemia not on statin therapy: the ODYSSEY CHOICE II study. J Am Heart Assoc. 2016;5(9):e003421. doi:10.1161/JAHA.116.003421
Atherosclerotic cardiovascular disease (ASCVD) is a significant cause of morbidity and mortality in the United States. ASCVD involves the buildup of cholesterol plaque in arteries and includes acute coronary syndrome, peripheral arterial disease, and events such as myocardial infarction and stroke.1 Cardiovascular disease (CVD) risk factors include high cholesterol levels, elevated blood pressure, insulin resistance, elevated blood glucose levels, smoking, poor dietary habits, and a sedentary lifestyle.2
According to the Centers for Disease Control and Prevention, about 86 million adults aged ≥ 20 years have total cholesterol levels > 200 mg/dL. More than half (54.5%) who could benefit are currently taking cholesterol-lowering medications.3 Controlling high cholesterol in American adults, especially veterans, is essential for reducing CVD morbidity and mortality.
The 2018 American College of Cardiology/American Heart Association (ACC/AHA) guideline recommends a low-density lipoprotein cholesterol (LDL-C) target goal of < 70 mg/dL for patients at high risk for ASCVD. Very high-risk ASCVD includes a history of multiple major ASCVD events or 1 major ASCVD event and multiple high-risk conditions (eg, age ≥ 65 years, smoking, or diabetes).4 Major ASCVD events include recent acute coronary syndrome (within the past 12 months), a history of myocardial infarction or ischemic stroke, and symptomatic peripheral artery disease.
The ACC/AHA guideline suggests that if the LDL-C level remains ≥ 70 mg/dL, adding ezetimibe (a dietary cholesterol absorption inhibitor) to maximally tolerated statin therapy is reasonable. If LDL-C levels remain ≥ 70 mg/dL, adding a proprotein convertase subtilisin/kexin type 9 (PCSK9) inhibitor, such as alirocumab, is reasonable.4 The US Departments of Veterans Affairs/US Department of Defense guidelines recommend using maximally tolerated statins and ezetimibe before PCSK9 inhibitors due to established long-term safety and reduction in CVD events.
Generic statins and ezetimibe are administered orally and widely available. In contrast, PCSK9 inhibitors have unknown long-term safety profiles, require subcutaneous injection once or twice monthly, and are significantly more expensive. They also require patient education on proper use while providing comparable or lesser relative risk reductions.2
These 3 classes of medication vary in their mechanisms of action to reduce LDL.5,6 Ezetimibe and several statin medications are included on the Veterans Affairs Sioux Falls Health Care System (VASFHCS) formulary and do not require review prior to prescribing. Alirocumab is available at VASFHCS but is restricted to patients with a history of ASCVD or a diagnosis of familial hypercholesterolemia, and who are receiving maximally tolerated statin and ezetimibe therapy but require further LDL-C lowering to reduce their ASCVD risk.
Studies have found ezetimibe monotherapy reduces LDL-C in patients with dyslipidemia by 18% after 12 weeks.7 One found that the percentage reduction in LDL-C was significantly greater (P < .001) with all doses of ezetimibe plus simvastatin (46% to 59%) compared with either atorvastatin 10 mg (37%) or simvastatin 20 mg (38%) monotherapy after 6 weeks.8
Although alirocumab can be added to other lipid therapies, most VASFHCS patients are prescribed alirocumab monotherapy. In the ODYSSEY CHOICE II study, patients were randomly assigned to receive either a placebo or alirocumab 150 mg every 4 weeks or alirocumab 75 mg every 2 weeks. The primary efficacy endpoint was LDL-C percentage change from baseline to week 24. In the alirocumab 150 mg every 4 weeks and 75 mg every 2 weeks groups, the least-squares mean LDL-C changes from baseline to week 24 were 51.7% and 53.5%, respectively, compared to a 4.7% increase in the placebo group (both groups P < .001 vs placebo). The authors also reported that alirocumab 150 mg every 4 weeks as monotherapy demonstrated a 47.4% reduction in LDL-C levels from baseline in a phase 1 study.9Although alirocumab monotherapy and ezetimibe plus statin therapy have been shown to effectively decrease LDL-C independently, a direct comparison of alirocumab monotherapy vs ezetimibe plus statin therapy has not been assessed, to our knowledge. Understanding the differences in effectiveness and safety between these 2 regimens will be valuable for clinicians when selecting a medication regimen for veterans with a history of ASCVD.
METHODS
This retrospective, single-center chart review used VASFHCS Computerized Patient Record System (CPRS) and Joint Longitudinal Viewer (JLV) records to compare patients with a history of ASCVD events who were treated with alirocumab monotherapy or ezetimibe plus statin. The 2 groups were randomized in a 1:3 ratio. The primary endpoint was achieving LDL-C < 70 mg/dL after 4 to 12 weeks, 13 to 24 weeks, and 25 to 52 weeks. Secondary endpoints included the mean percentage change from baseline in total cholesterol (TC), high-density lipoprotein cholesterol (HDL-C), LDL-C, and triglycerides (TG) over 52 weeks. The incidence of ASCVD events during this period was also assessed. If LDL-C < 70 mg/dL was achieved > 1 time during each time frame, only 1 incident was counted for analysis. Safety was assessed based on the incidence of any adverse event (AE) that led to treatment discontinuation.
Patients were identified by screening the prescription fill history between October 1, 2019, and December 31, 2022. The 52-week data collection period was counted from the first available fill date. Additionally, the prior authorization drug request file from January 1, 2017, to December 31, 2022, was used to obtain a list of patients prescribed alirocumab. Patients were included if they were veterans aged ≥ 18 years and had a history of an ASCVD event, had a alirocumab monotherapy or ezetimibe plus statin prescription between October 1, 2019, and December 31, 2022, or had an approved prior authorization drug request for alirocumab between January 1, 2017, and December 31, 2022. Patients missing a baseline or follow-up lipid panel and those with concurrent use of alirocumab and ezetimibe and/or statin were excluded.
Baseline characteristics collected for patients included age, sex, race, weight, body mass index, lipid parameters (LDL-C, TC, HDL-C, and TG), dosing of each type of statin before adding ezetimibe, and use of any other antihyperlipidemic medication. We also collected histories of hypertension, hyperlipidemia, diabetes, chronic kidney disease, congestive heart failure, and smoking or tobacco use status. The baseline lipid panel was the most recent lipid panel documented before starting alirocumab or ezetimibe plus statin therapy. Follow-up lipid panel values were gathered at 4 to 12 weeks, 13 to 24 weeks, and 25 to 52 weeks following initiation of either therapy.
High-, moderate-, and low-intensity dosing of statin therapy and alirocumab dosing (75 mg every 2 weeks, 150 mg every 2 weeks, or 300 mg every 4 weeks) were recorded at the specified intervals. However, no patients in this study received the latter dosing regimen. ASCVD events and safety endpoints were recorded based on a review of clinical notes over the 52 weeks following the first available start date.
Statistical Analysis
The primary endpoint of achieving the LDL-C < 70 mg/dL goal from baseline to 4 to 12 weeks, 13 to 24 weeks, and 25 to 52 weeks after initiation was compared between alirocumab monotherapy and ezetimibe plus statin therapy using the χ² test. Mean percentage change from baseline in TC, HDL-C, LDL-C, and TG were compared using the independent t test. P < .05 was considered statistically significant. Incidence of ASCVD events and the safety endpoint (incidence of AEs leading to treatment discontinuation) were also compared using the χ² test. Continuous baseline characteristics were reported mean (SD) and nominal baseline characteristics were reported as a percentage.
RESULTS
There were 80 participants in this study: 20 in the alirocumab monotherapy group and 60 in the ezetimibe plus statin therapy group. More than 100 patients did not meet the prespecified inclusion criteria and were excluded. Mean (SD) age was 75 (8) years in the alirocumab group and 74 (8) years in the ezetimibe plus statin group. There was no significant differences in mean (SD) weight or mean (SD) body mass index. All study participants identified as White and male except for 2 patients in the ezetimibe plus statin therapy group whose race was not documented. Differences in lipid parameters were observed between groups, with mean baseline LDL-C, HDL-C, and TC higher in the alirocumab monotherapy group than in the ezetimibe plus statin therapy group, with significant differences in LDL-C and TC (Table 1).
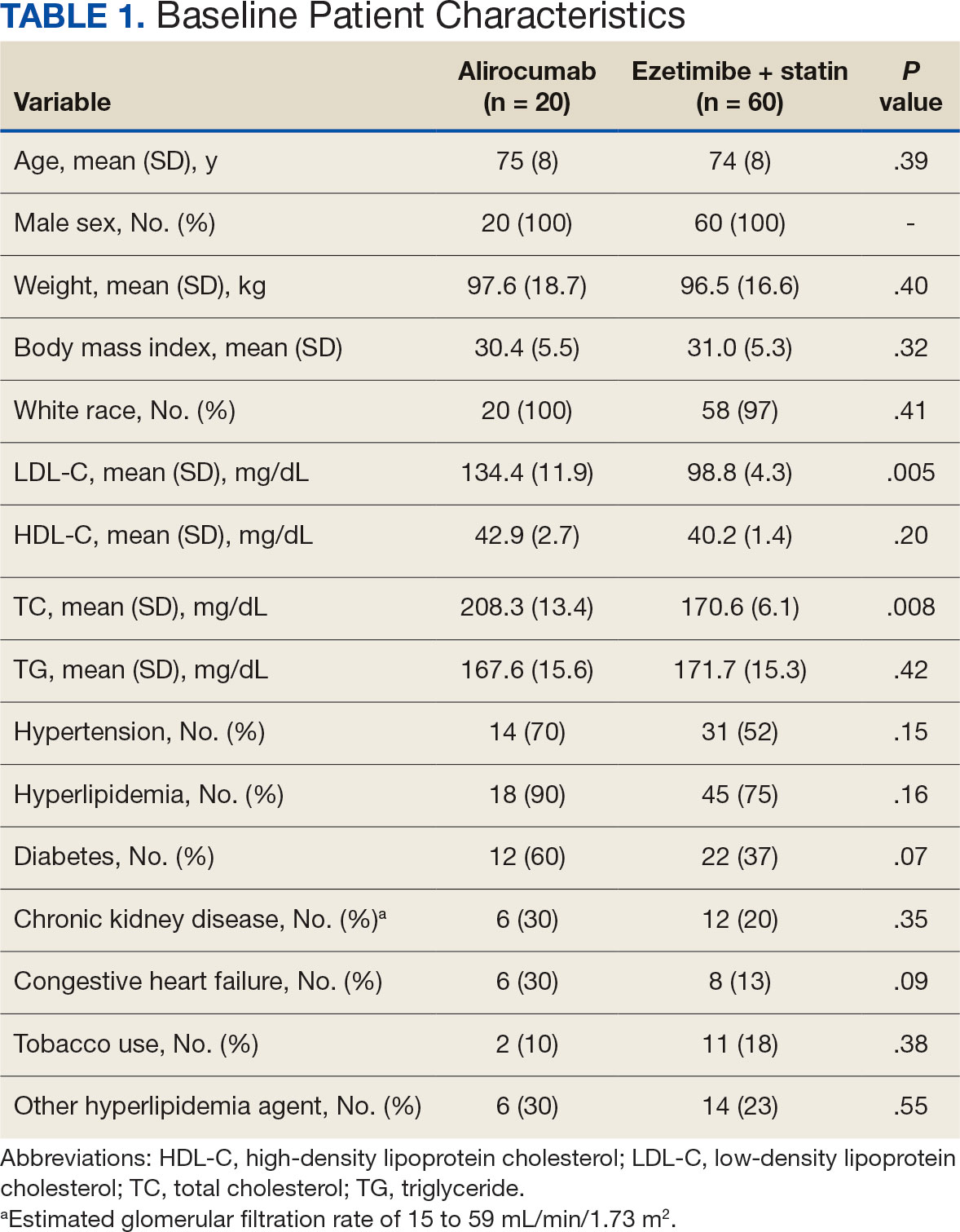
Fourteen patients (70%) in the alirocumab monotherapy group had hypertension, compared with 31 (52%) in the ezetimibe plus statin therapy group. In both groups, most patients had previously been diagnosed with hyperlipidemia. More patients (60%) in the alirocumab group had diabetes than in the ezetimibe plus statin therapy group (37%). The alirocumab monotherapy group also had a higher percentage of patients with diagnoses of congestive heart failure and used other antihyperlipidemic medications than in the ezetimibe plus statin therapy group. Five patients (25%) in the alirocumab monotherapy group and 12 patients (20%) in the ezetimibe plus statin therapy group took fish oil. In the ezetimibe plus statin therapy group, 2 patients (3%) took gemfibrozil, and 2 patients (3%) took fenofibrate. Six (30%) patients in the alirocumab monotherapy group and 12 (20%) patients in the ezetimibe plus statin therapy group had chronic kidney disease. Although the majority of patients in each group did not use tobacco products, there were more tobacco users in the ezetimibe plus statin therapy group.
In the alirocumab monotherapy group, 15 patients (75%) were prescribed 75 mg every 2 weeks and 5 patients (25%) were prescribed 150 mg every 2 weeks. In the ezetimibe plus statin therapy group, 59 patients (98%) were prescribed ezetimibe 10 mg/d (Table 2). Forty-three patients (72%) were prescribed a high-intensity statin 10 received moderate-intensity (17%) and 7 received low-intensity statin (12%). Most patients were prescribed rosuvastatin (45%), followed by atorvastatin (42%), pravastatin (10%), and simvastatin (3%).
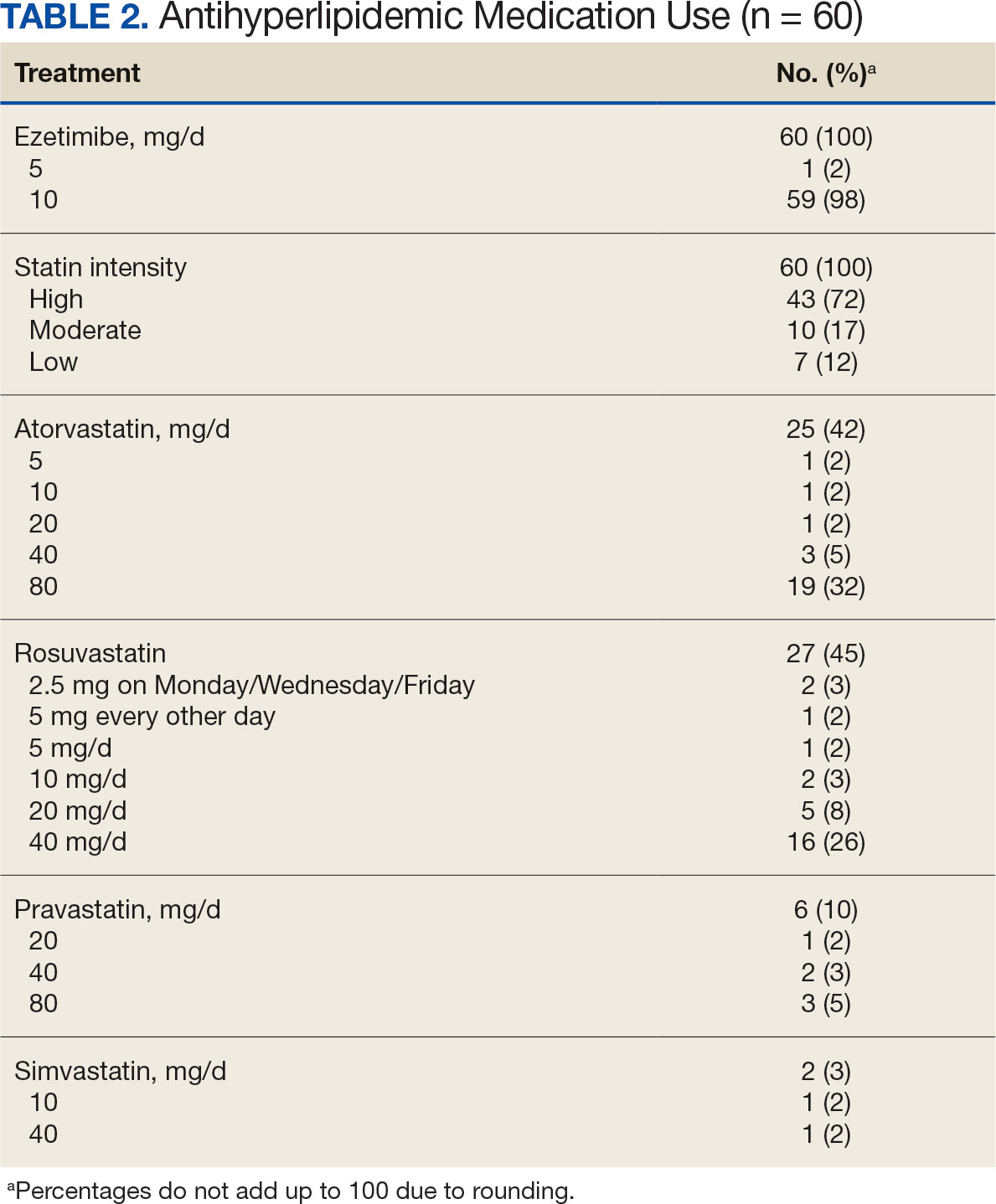
Primary Endpoint
During the 52-week study, more patients met the LDL-C goal of < 70 mg/dL in the alirocumab monotherapy group (70%) than in the ezetimibe plus statin therapy group (57%); however, the difference was not significant (P = .29). Of the patients prescribed alirocumab monotherapy who achieved LDL-C < 70 mg/dL, 15% achieved this goal in 4 to 12 weeks, 40% in 13 to 24 weeks, and 45% in 25 to 52 weeks. In the ezetimibe plus statin therapy group, 28% of patients achieved LDL-C < 70 mg/dL in 4 to 12 weeks, 31% in 13 to 24 weeks, and 41% in 25 to 52 weeks (Table 3).
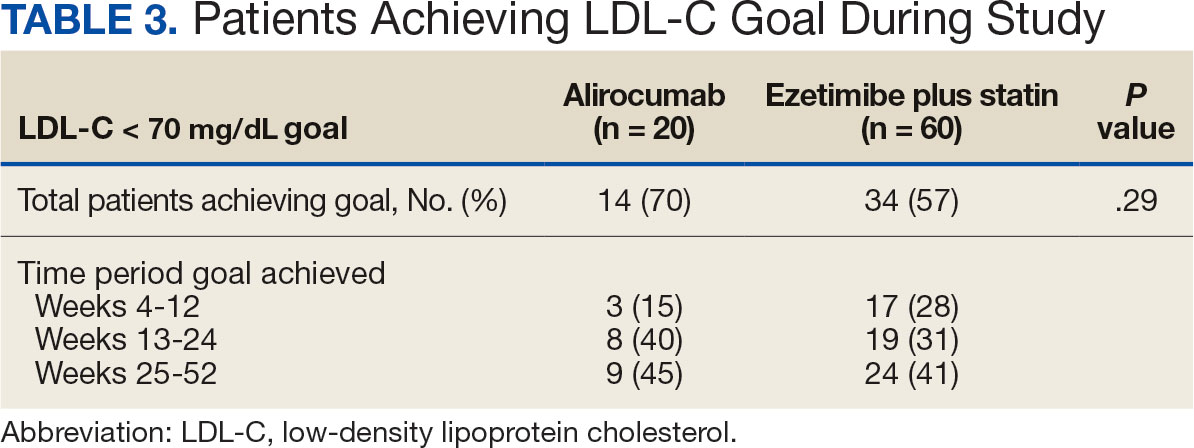
Secondary Endpoints
During weeks 4 to 52 of treatment, the mean percentage change decreased in LDL-C (37.7% vs 21.4%; P = .01), TC (24.7% vs 12.5%; P = .01), and TG (0.9% vs 7.0%; P = .28) in the alirocumab monotherapy group and the ezetimibe plus statin therapy group, respectively (Table 4). The mean percentage change increased in HDL-C by 3.6% in the alirocumab monotherapy group and 1.8% in the ezetimibe plus statin therapy group (P = .36). During the study, ASCVD events occurred in 1 patient (5%) in the alirocumab monotherapy group and 3 patients (5%) in the ezetimibe plus statin therapy group (P = .99). The patient in the alirocumab monotherapy group had unstable angina 1 month after taking alirocumab. One patient in the ezetimibe plus statin therapy group had coronary artery disease and 2 patients had coronary heart disease that required stents during the 52-week period. There was 1 patient in each group who reported an AE that led to treatment discontinuation (P = .41). One patient stopped alirocumab after a trial of 2 months due to intolerance, but no specific AE was reported in the CPRS. In the ezetimibe plus statin therapy group, 1 patient requested to discontinue ezetimibe after a trial of 3 months without a specific reason noted in the medical record.
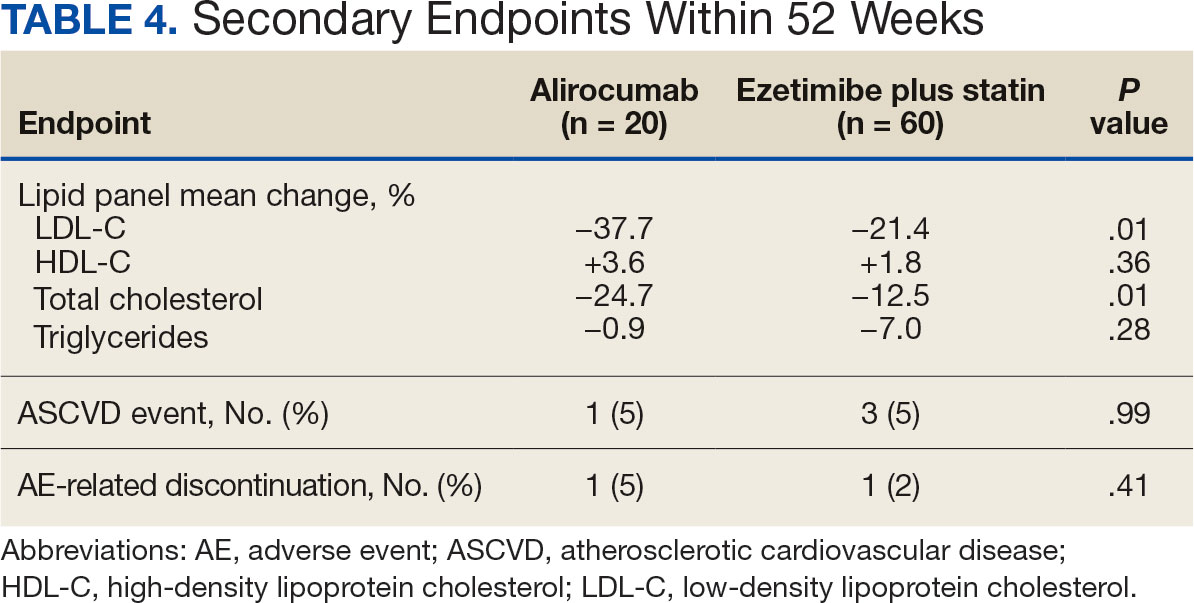
DISCUSSION
This study found no statistically significant difference in the incidence of reaching an LDL-C goal of < 70 mg/dL after alirocumab monotherapy initiation compared with ezetimibe plus statin therapy. This occurred despite baseline LDL-C being lower in the ezetimibe plus statin therapy group, which required a smaller reduction in LDL-C to reach the primary goal. Most patients on alirocumab monotherapy were prescribed a lower initial dose of 75 mg every 2 weeks. Of those patients, 30% did not achieve the LDL-C goal < 70 mg/dL. Thus, a higher dose may have led to more patients achieving the LDL-C goal.
Secondary endpoints, including mean percentage change in HDL-C and TG and incidence of ASCVD events during 52 weeks of treatment, were not statistically significant. The mean percentage increase in HDL-C was negligible in both groups, while the mean percentage reduction in TG favored the ezetimibe plus statin therapy group. In the ezetimibe plus statin therapy group, patients who also took fenofibrate experienced a significant reduction in TG while none of the patients in the alirocumab group were prescribed fenofibrate. Although the alirocumab monotherapy group had a statistically significant greater reduction in LDL-C and TC compared with those prescribed ezetimibe plus statin, the mean baseline LDL-C and TC were significantly greater in the alirocumab monotherapy group, which could contribute to higher reductions in LDL-C and TC after alirocumab monotherapy.Based on the available literature, we expected greater reductions in LDL-C in both study groups compared with statin therapy alone.8,9 However, it was unclear whether the LDL-C and TC reductions were clinically significant.
Limitations
The study design did not permit randomization prior to the treatments, restricting our ability to account for some confounding factors, such as diet, exercise, other antihyperlipidemic medication, and medication adherence, which may have affected LDL-C, HDL-C, TG, and TC levels. Differences in baseline characteristics—particularly major risk factors, such as hypertension, diabetes, and tobacco use—also could have confounding affect on lipid levels and ASCVD events. Additionally, patients prescribed alirocumab monotherapy may have switched from statin or ezetimibe therapy, and the washout period was not reviewed or recorded, which could have affected the lipid panel results.
The small sample size of this study also may have limited the ability to detect significant differences between groups. A direct comparison of alirocumab monotherapy vs ezetimibe plus statin therapy has not been performed, making it difficult to prospectively evaluate what sample size would be needed to power this study. A posthoc analysis was used to calculate power, which was found to be only 17%. Many patients were excluded due to a lack of laboratory results within the study period, contributing to the small sample size.
Another limitation was the reliance on documentation in CPRS and JLV. For example, having documentation of the specific AEs for the 2 patients who discontinued alirocumab or ezetimibe could have helped determine the severity of the AEs. Several patients were followed by non-VA clinicians, which could have contributed to limited documentation in the CPRS and JLV. It is difficult to draw any conclusions regarding ASCVD events and AEs that led to treatment discontinuation between alirocumab monotherapy and ezetimibe plus statin therapy based on the results of this retrospective study due to the limited number of events within the 52-week period.
CONCLUSIONS
This study found that there was no statistically significant difference in LDL-C reduction to < 70 mg/dL between alirocumab monotherapy and ezetimibe plus statin therapy in a small population of veterans with ASCVD, with a higher percentage of participants in both groups achieving that goal in 25 to 52 weeks. There also was no significant difference in percentage change in HDL-C or TG or in incidence of ASCVD events and AEs leading to treatment discontinuation. However, there was a statistically significant difference in percentage reduction for LDL-C and TC during 52 weeks of alirocumab monotherapy vs ezetimibe plus statin therapy.
Although there was no significant difference in LDL-C reduction to < 70 mg/dL, targeting this goal in patients with ASCVD is still clinically warranted. This study does not support a change in current VA criteria for use of alirocumab or a change in current guidelines for secondary prevention of ASCVD. Still, this study does indicate that the efficacy of alirocumab monotherapy is similar to that of ezetimibe plus statin therapy in patients with a history of ASCVD and may be useful in clinical settings when an alternative to ezetimibe plus statin therapy is needed. Alirocumab also may be more effective in lowering LDL-C and TC than ezetimibe plus statin therapy in veterans with ASCVD and could be added to statin therapy or ezetimibe when additional LDL-C or TC reduction is needed.
Atherosclerotic cardiovascular disease (ASCVD) is a significant cause of morbidity and mortality in the United States. ASCVD involves the buildup of cholesterol plaque in arteries and includes acute coronary syndrome, peripheral arterial disease, and events such as myocardial infarction and stroke.1 Cardiovascular disease (CVD) risk factors include high cholesterol levels, elevated blood pressure, insulin resistance, elevated blood glucose levels, smoking, poor dietary habits, and a sedentary lifestyle.2
According to the Centers for Disease Control and Prevention, about 86 million adults aged ≥ 20 years have total cholesterol levels > 200 mg/dL. More than half (54.5%) who could benefit are currently taking cholesterol-lowering medications.3 Controlling high cholesterol in American adults, especially veterans, is essential for reducing CVD morbidity and mortality.
The 2018 American College of Cardiology/American Heart Association (ACC/AHA) guideline recommends a low-density lipoprotein cholesterol (LDL-C) target goal of < 70 mg/dL for patients at high risk for ASCVD. Very high-risk ASCVD includes a history of multiple major ASCVD events or 1 major ASCVD event and multiple high-risk conditions (eg, age ≥ 65 years, smoking, or diabetes).4 Major ASCVD events include recent acute coronary syndrome (within the past 12 months), a history of myocardial infarction or ischemic stroke, and symptomatic peripheral artery disease.
The ACC/AHA guideline suggests that if the LDL-C level remains ≥ 70 mg/dL, adding ezetimibe (a dietary cholesterol absorption inhibitor) to maximally tolerated statin therapy is reasonable. If LDL-C levels remain ≥ 70 mg/dL, adding a proprotein convertase subtilisin/kexin type 9 (PCSK9) inhibitor, such as alirocumab, is reasonable.4 The US Departments of Veterans Affairs/US Department of Defense guidelines recommend using maximally tolerated statins and ezetimibe before PCSK9 inhibitors due to established long-term safety and reduction in CVD events.
Generic statins and ezetimibe are administered orally and widely available. In contrast, PCSK9 inhibitors have unknown long-term safety profiles, require subcutaneous injection once or twice monthly, and are significantly more expensive. They also require patient education on proper use while providing comparable or lesser relative risk reductions.2
These 3 classes of medication vary in their mechanisms of action to reduce LDL.5,6 Ezetimibe and several statin medications are included on the Veterans Affairs Sioux Falls Health Care System (VASFHCS) formulary and do not require review prior to prescribing. Alirocumab is available at VASFHCS but is restricted to patients with a history of ASCVD or a diagnosis of familial hypercholesterolemia, and who are receiving maximally tolerated statin and ezetimibe therapy but require further LDL-C lowering to reduce their ASCVD risk.
Studies have found ezetimibe monotherapy reduces LDL-C in patients with dyslipidemia by 18% after 12 weeks.7 One found that the percentage reduction in LDL-C was significantly greater (P < .001) with all doses of ezetimibe plus simvastatin (46% to 59%) compared with either atorvastatin 10 mg (37%) or simvastatin 20 mg (38%) monotherapy after 6 weeks.8
Although alirocumab can be added to other lipid therapies, most VASFHCS patients are prescribed alirocumab monotherapy. In the ODYSSEY CHOICE II study, patients were randomly assigned to receive either a placebo or alirocumab 150 mg every 4 weeks or alirocumab 75 mg every 2 weeks. The primary efficacy endpoint was LDL-C percentage change from baseline to week 24. In the alirocumab 150 mg every 4 weeks and 75 mg every 2 weeks groups, the least-squares mean LDL-C changes from baseline to week 24 were 51.7% and 53.5%, respectively, compared to a 4.7% increase in the placebo group (both groups P < .001 vs placebo). The authors also reported that alirocumab 150 mg every 4 weeks as monotherapy demonstrated a 47.4% reduction in LDL-C levels from baseline in a phase 1 study.9Although alirocumab monotherapy and ezetimibe plus statin therapy have been shown to effectively decrease LDL-C independently, a direct comparison of alirocumab monotherapy vs ezetimibe plus statin therapy has not been assessed, to our knowledge. Understanding the differences in effectiveness and safety between these 2 regimens will be valuable for clinicians when selecting a medication regimen for veterans with a history of ASCVD.
METHODS
This retrospective, single-center chart review used VASFHCS Computerized Patient Record System (CPRS) and Joint Longitudinal Viewer (JLV) records to compare patients with a history of ASCVD events who were treated with alirocumab monotherapy or ezetimibe plus statin. The 2 groups were randomized in a 1:3 ratio. The primary endpoint was achieving LDL-C < 70 mg/dL after 4 to 12 weeks, 13 to 24 weeks, and 25 to 52 weeks. Secondary endpoints included the mean percentage change from baseline in total cholesterol (TC), high-density lipoprotein cholesterol (HDL-C), LDL-C, and triglycerides (TG) over 52 weeks. The incidence of ASCVD events during this period was also assessed. If LDL-C < 70 mg/dL was achieved > 1 time during each time frame, only 1 incident was counted for analysis. Safety was assessed based on the incidence of any adverse event (AE) that led to treatment discontinuation.
Patients were identified by screening the prescription fill history between October 1, 2019, and December 31, 2022. The 52-week data collection period was counted from the first available fill date. Additionally, the prior authorization drug request file from January 1, 2017, to December 31, 2022, was used to obtain a list of patients prescribed alirocumab. Patients were included if they were veterans aged ≥ 18 years and had a history of an ASCVD event, had a alirocumab monotherapy or ezetimibe plus statin prescription between October 1, 2019, and December 31, 2022, or had an approved prior authorization drug request for alirocumab between January 1, 2017, and December 31, 2022. Patients missing a baseline or follow-up lipid panel and those with concurrent use of alirocumab and ezetimibe and/or statin were excluded.
Baseline characteristics collected for patients included age, sex, race, weight, body mass index, lipid parameters (LDL-C, TC, HDL-C, and TG), dosing of each type of statin before adding ezetimibe, and use of any other antihyperlipidemic medication. We also collected histories of hypertension, hyperlipidemia, diabetes, chronic kidney disease, congestive heart failure, and smoking or tobacco use status. The baseline lipid panel was the most recent lipid panel documented before starting alirocumab or ezetimibe plus statin therapy. Follow-up lipid panel values were gathered at 4 to 12 weeks, 13 to 24 weeks, and 25 to 52 weeks following initiation of either therapy.
High-, moderate-, and low-intensity dosing of statin therapy and alirocumab dosing (75 mg every 2 weeks, 150 mg every 2 weeks, or 300 mg every 4 weeks) were recorded at the specified intervals. However, no patients in this study received the latter dosing regimen. ASCVD events and safety endpoints were recorded based on a review of clinical notes over the 52 weeks following the first available start date.
Statistical Analysis
The primary endpoint of achieving the LDL-C < 70 mg/dL goal from baseline to 4 to 12 weeks, 13 to 24 weeks, and 25 to 52 weeks after initiation was compared between alirocumab monotherapy and ezetimibe plus statin therapy using the χ² test. Mean percentage change from baseline in TC, HDL-C, LDL-C, and TG were compared using the independent t test. P < .05 was considered statistically significant. Incidence of ASCVD events and the safety endpoint (incidence of AEs leading to treatment discontinuation) were also compared using the χ² test. Continuous baseline characteristics were reported mean (SD) and nominal baseline characteristics were reported as a percentage.
RESULTS
There were 80 participants in this study: 20 in the alirocumab monotherapy group and 60 in the ezetimibe plus statin therapy group. More than 100 patients did not meet the prespecified inclusion criteria and were excluded. Mean (SD) age was 75 (8) years in the alirocumab group and 74 (8) years in the ezetimibe plus statin group. There was no significant differences in mean (SD) weight or mean (SD) body mass index. All study participants identified as White and male except for 2 patients in the ezetimibe plus statin therapy group whose race was not documented. Differences in lipid parameters were observed between groups, with mean baseline LDL-C, HDL-C, and TC higher in the alirocumab monotherapy group than in the ezetimibe plus statin therapy group, with significant differences in LDL-C and TC (Table 1).
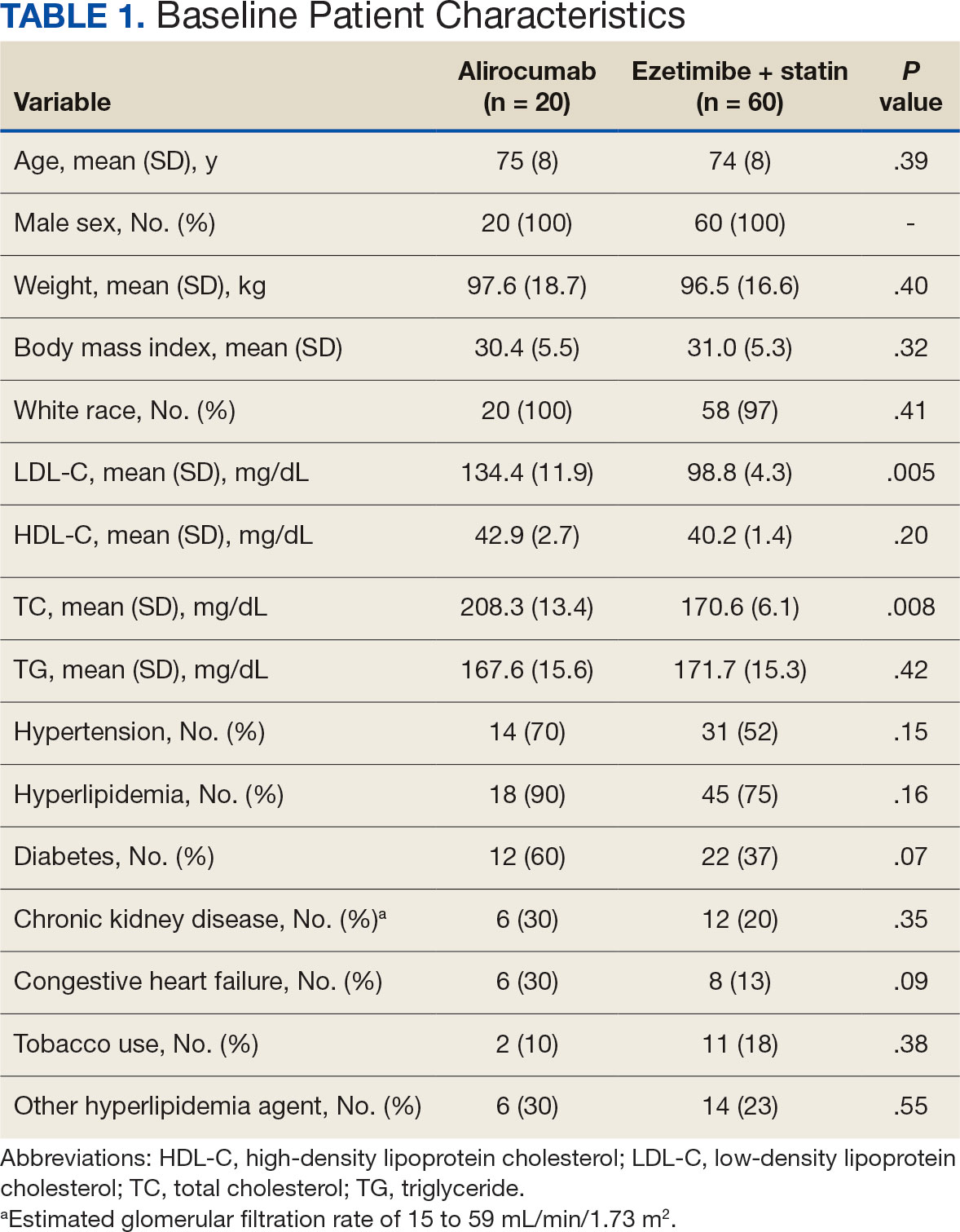
Fourteen patients (70%) in the alirocumab monotherapy group had hypertension, compared with 31 (52%) in the ezetimibe plus statin therapy group. In both groups, most patients had previously been diagnosed with hyperlipidemia. More patients (60%) in the alirocumab group had diabetes than in the ezetimibe plus statin therapy group (37%). The alirocumab monotherapy group also had a higher percentage of patients with diagnoses of congestive heart failure and used other antihyperlipidemic medications than in the ezetimibe plus statin therapy group. Five patients (25%) in the alirocumab monotherapy group and 12 patients (20%) in the ezetimibe plus statin therapy group took fish oil. In the ezetimibe plus statin therapy group, 2 patients (3%) took gemfibrozil, and 2 patients (3%) took fenofibrate. Six (30%) patients in the alirocumab monotherapy group and 12 (20%) patients in the ezetimibe plus statin therapy group had chronic kidney disease. Although the majority of patients in each group did not use tobacco products, there were more tobacco users in the ezetimibe plus statin therapy group.
In the alirocumab monotherapy group, 15 patients (75%) were prescribed 75 mg every 2 weeks and 5 patients (25%) were prescribed 150 mg every 2 weeks. In the ezetimibe plus statin therapy group, 59 patients (98%) were prescribed ezetimibe 10 mg/d (Table 2). Forty-three patients (72%) were prescribed a high-intensity statin 10 received moderate-intensity (17%) and 7 received low-intensity statin (12%). Most patients were prescribed rosuvastatin (45%), followed by atorvastatin (42%), pravastatin (10%), and simvastatin (3%).
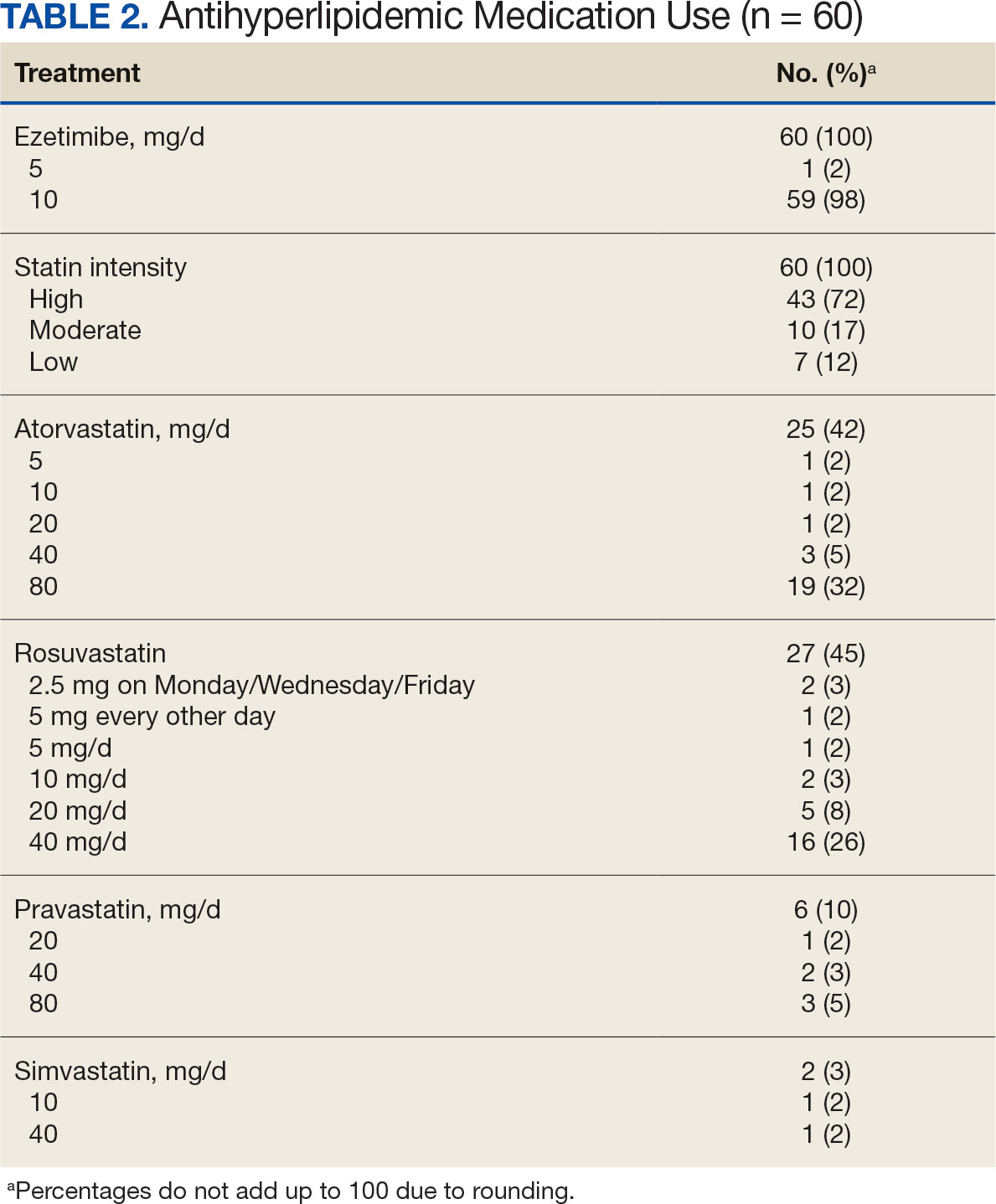
Primary Endpoint
During the 52-week study, more patients met the LDL-C goal of < 70 mg/dL in the alirocumab monotherapy group (70%) than in the ezetimibe plus statin therapy group (57%); however, the difference was not significant (P = .29). Of the patients prescribed alirocumab monotherapy who achieved LDL-C < 70 mg/dL, 15% achieved this goal in 4 to 12 weeks, 40% in 13 to 24 weeks, and 45% in 25 to 52 weeks. In the ezetimibe plus statin therapy group, 28% of patients achieved LDL-C < 70 mg/dL in 4 to 12 weeks, 31% in 13 to 24 weeks, and 41% in 25 to 52 weeks (Table 3).
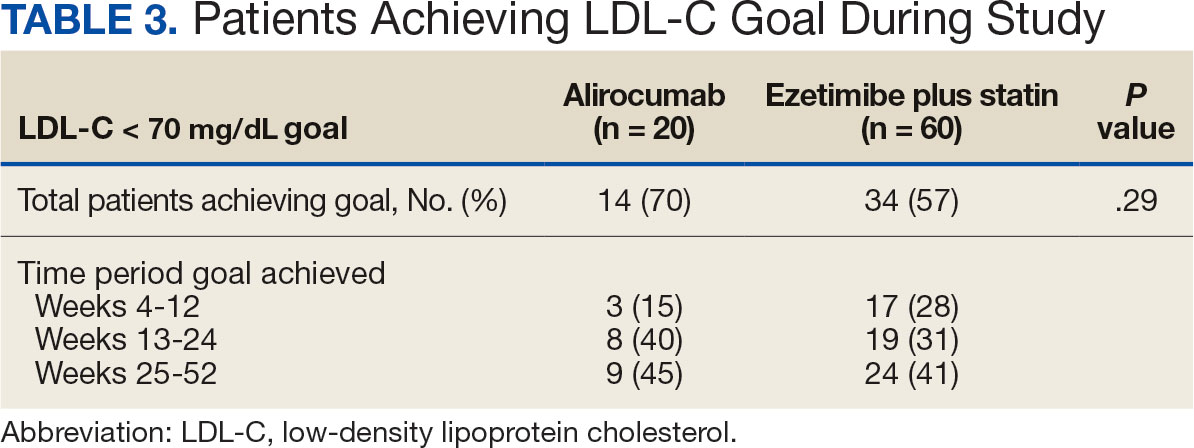
Secondary Endpoints
During weeks 4 to 52 of treatment, the mean percentage change decreased in LDL-C (37.7% vs 21.4%; P = .01), TC (24.7% vs 12.5%; P = .01), and TG (0.9% vs 7.0%; P = .28) in the alirocumab monotherapy group and the ezetimibe plus statin therapy group, respectively (Table 4). The mean percentage change increased in HDL-C by 3.6% in the alirocumab monotherapy group and 1.8% in the ezetimibe plus statin therapy group (P = .36). During the study, ASCVD events occurred in 1 patient (5%) in the alirocumab monotherapy group and 3 patients (5%) in the ezetimibe plus statin therapy group (P = .99). The patient in the alirocumab monotherapy group had unstable angina 1 month after taking alirocumab. One patient in the ezetimibe plus statin therapy group had coronary artery disease and 2 patients had coronary heart disease that required stents during the 52-week period. There was 1 patient in each group who reported an AE that led to treatment discontinuation (P = .41). One patient stopped alirocumab after a trial of 2 months due to intolerance, but no specific AE was reported in the CPRS. In the ezetimibe plus statin therapy group, 1 patient requested to discontinue ezetimibe after a trial of 3 months without a specific reason noted in the medical record.
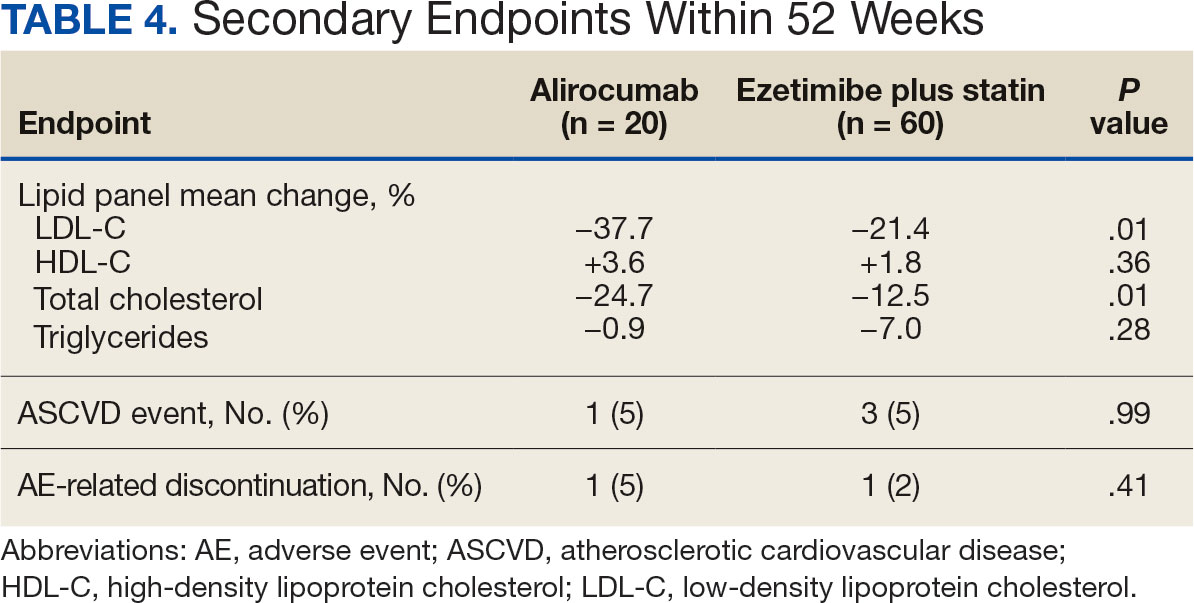
DISCUSSION
This study found no statistically significant difference in the incidence of reaching an LDL-C goal of < 70 mg/dL after alirocumab monotherapy initiation compared with ezetimibe plus statin therapy. This occurred despite baseline LDL-C being lower in the ezetimibe plus statin therapy group, which required a smaller reduction in LDL-C to reach the primary goal. Most patients on alirocumab monotherapy were prescribed a lower initial dose of 75 mg every 2 weeks. Of those patients, 30% did not achieve the LDL-C goal < 70 mg/dL. Thus, a higher dose may have led to more patients achieving the LDL-C goal.
Secondary endpoints, including mean percentage change in HDL-C and TG and incidence of ASCVD events during 52 weeks of treatment, were not statistically significant. The mean percentage increase in HDL-C was negligible in both groups, while the mean percentage reduction in TG favored the ezetimibe plus statin therapy group. In the ezetimibe plus statin therapy group, patients who also took fenofibrate experienced a significant reduction in TG while none of the patients in the alirocumab group were prescribed fenofibrate. Although the alirocumab monotherapy group had a statistically significant greater reduction in LDL-C and TC compared with those prescribed ezetimibe plus statin, the mean baseline LDL-C and TC were significantly greater in the alirocumab monotherapy group, which could contribute to higher reductions in LDL-C and TC after alirocumab monotherapy.Based on the available literature, we expected greater reductions in LDL-C in both study groups compared with statin therapy alone.8,9 However, it was unclear whether the LDL-C and TC reductions were clinically significant.
Limitations
The study design did not permit randomization prior to the treatments, restricting our ability to account for some confounding factors, such as diet, exercise, other antihyperlipidemic medication, and medication adherence, which may have affected LDL-C, HDL-C, TG, and TC levels. Differences in baseline characteristics—particularly major risk factors, such as hypertension, diabetes, and tobacco use—also could have confounding affect on lipid levels and ASCVD events. Additionally, patients prescribed alirocumab monotherapy may have switched from statin or ezetimibe therapy, and the washout period was not reviewed or recorded, which could have affected the lipid panel results.
The small sample size of this study also may have limited the ability to detect significant differences between groups. A direct comparison of alirocumab monotherapy vs ezetimibe plus statin therapy has not been performed, making it difficult to prospectively evaluate what sample size would be needed to power this study. A posthoc analysis was used to calculate power, which was found to be only 17%. Many patients were excluded due to a lack of laboratory results within the study period, contributing to the small sample size.
Another limitation was the reliance on documentation in CPRS and JLV. For example, having documentation of the specific AEs for the 2 patients who discontinued alirocumab or ezetimibe could have helped determine the severity of the AEs. Several patients were followed by non-VA clinicians, which could have contributed to limited documentation in the CPRS and JLV. It is difficult to draw any conclusions regarding ASCVD events and AEs that led to treatment discontinuation between alirocumab monotherapy and ezetimibe plus statin therapy based on the results of this retrospective study due to the limited number of events within the 52-week period.
CONCLUSIONS
This study found that there was no statistically significant difference in LDL-C reduction to < 70 mg/dL between alirocumab monotherapy and ezetimibe plus statin therapy in a small population of veterans with ASCVD, with a higher percentage of participants in both groups achieving that goal in 25 to 52 weeks. There also was no significant difference in percentage change in HDL-C or TG or in incidence of ASCVD events and AEs leading to treatment discontinuation. However, there was a statistically significant difference in percentage reduction for LDL-C and TC during 52 weeks of alirocumab monotherapy vs ezetimibe plus statin therapy.
Although there was no significant difference in LDL-C reduction to < 70 mg/dL, targeting this goal in patients with ASCVD is still clinically warranted. This study does not support a change in current VA criteria for use of alirocumab or a change in current guidelines for secondary prevention of ASCVD. Still, this study does indicate that the efficacy of alirocumab monotherapy is similar to that of ezetimibe plus statin therapy in patients with a history of ASCVD and may be useful in clinical settings when an alternative to ezetimibe plus statin therapy is needed. Alirocumab also may be more effective in lowering LDL-C and TC than ezetimibe plus statin therapy in veterans with ASCVD and could be added to statin therapy or ezetimibe when additional LDL-C or TC reduction is needed.
Lucchi T. Dyslipidemia and prevention of atherosclerotic cardiovascular disease in the elderly. Minerva Med. 2021;112:804-816. doi:10.23736/S0026-4806.21.07347-X
The Management of Dyslipidemia for Cardiovascular Risk Reduction Work Group. VA/DoD Clinical Practice Guideline for the Management of Dyslipidemia for Cardiovascular Risk Reduction. Version 4.0. June 2020. Accessed September 5, 2024. https://www.healthquality.va.gov/guidelines/CD/lipids/VADoDDyslipidemiaCPG5087212020.pdf
Centers for Disease Control and Prevention. High Cholesterol Facts. May 15, 2024. Accessed October 3, 2024. https://www.cdc.gov/cholesterol/data-research/facts-stats/index.html
Grundy SM, Stone NJ, Bailey AL, et al. 2018 AHA/ACC/AACVPR/AAPA/ABC/ACPM/ADA/AGS/APhA/ASPC/NLA/PCNA guideline on the management of blood cholesterol: a report of the American College of Cardiology/American Heart Association Task Force on Clinical Practice Guidelines. Circulation. 2019;139:e1082-e1143. doi:10.1161/CIR.0000000000000625
Vavlukis M, Vavlukis A. Statins alone or in combination with ezetimibe or PCSK9 inhibitors in atherosclerotic cardiovascular disease protection. IntechOpen. January 24, 2019. doi:10.5772/intechopen.82520
Alirocumab. Prescribing information. Regeneron Pharmaceuticals, Inc.; 2024. Accessed September 5, 2024. https://www.regeneron.com/downloads/praluent_pi.pdf
Pandor A, Ara RM, Tumur I, et al. Ezetimibe monotherapy for cholesterol lowering in 2,722 people: systematic review and meta-analysis of randomized controlled trials. J Intern Med. 2009;265(5):568-580. doi:10.1111/j.1365-2796.2008.02062.x
McKenney J, Ballantyne CM, Feldman TA, et al. LDL-C goal attainment with ezetimibe plus simvastatin coadministration vs atorvastatin or simvastatin monotherapy in patients at high risk of CHD. MedGenMed. 2005;7(3):3.
Stroes E, Guyton JR, Lepor N, et al. Efficacy and safety of alirocumab 150 mg every 4 weeks in patients with hypercholesterolemia not on statin therapy: the ODYSSEY CHOICE II study. J Am Heart Assoc. 2016;5(9):e003421. doi:10.1161/JAHA.116.003421
Lucchi T. Dyslipidemia and prevention of atherosclerotic cardiovascular disease in the elderly. Minerva Med. 2021;112:804-816. doi:10.23736/S0026-4806.21.07347-X
The Management of Dyslipidemia for Cardiovascular Risk Reduction Work Group. VA/DoD Clinical Practice Guideline for the Management of Dyslipidemia for Cardiovascular Risk Reduction. Version 4.0. June 2020. Accessed September 5, 2024. https://www.healthquality.va.gov/guidelines/CD/lipids/VADoDDyslipidemiaCPG5087212020.pdf
Centers for Disease Control and Prevention. High Cholesterol Facts. May 15, 2024. Accessed October 3, 2024. https://www.cdc.gov/cholesterol/data-research/facts-stats/index.html
Grundy SM, Stone NJ, Bailey AL, et al. 2018 AHA/ACC/AACVPR/AAPA/ABC/ACPM/ADA/AGS/APhA/ASPC/NLA/PCNA guideline on the management of blood cholesterol: a report of the American College of Cardiology/American Heart Association Task Force on Clinical Practice Guidelines. Circulation. 2019;139:e1082-e1143. doi:10.1161/CIR.0000000000000625
Vavlukis M, Vavlukis A. Statins alone or in combination with ezetimibe or PCSK9 inhibitors in atherosclerotic cardiovascular disease protection. IntechOpen. January 24, 2019. doi:10.5772/intechopen.82520
Alirocumab. Prescribing information. Regeneron Pharmaceuticals, Inc.; 2024. Accessed September 5, 2024. https://www.regeneron.com/downloads/praluent_pi.pdf
Pandor A, Ara RM, Tumur I, et al. Ezetimibe monotherapy for cholesterol lowering in 2,722 people: systematic review and meta-analysis of randomized controlled trials. J Intern Med. 2009;265(5):568-580. doi:10.1111/j.1365-2796.2008.02062.x
McKenney J, Ballantyne CM, Feldman TA, et al. LDL-C goal attainment with ezetimibe plus simvastatin coadministration vs atorvastatin or simvastatin monotherapy in patients at high risk of CHD. MedGenMed. 2005;7(3):3.
Stroes E, Guyton JR, Lepor N, et al. Efficacy and safety of alirocumab 150 mg every 4 weeks in patients with hypercholesterolemia not on statin therapy: the ODYSSEY CHOICE II study. J Am Heart Assoc. 2016;5(9):e003421. doi:10.1161/JAHA.116.003421
The Safety and Efficacy of AUC/MIC-Guided vs Trough-Guided Vancomycin Monitoring Among Veterans
Vancomycin is a commonly used glycopeptide antibiotic used to treat infections caused by gram-positive organisms. Vancomycin is most often used as a parenteral agent for empiric or definitive treatment of methicillin-resistant Staphylococcus aureus (MRSA). It can also be used for the treatment of other susceptible Staphylococcus or Enterococcus species. Adverse effects of parenteral vancomycin include infusion-related reactions, ototoxicity, and nephrotoxicity.1 Higher vancomycin trough levels have been associated with an increased risk of nephrotoxicity.1-4 The major safety concern with vancomycin is acute kidney injury (AKI). Even mild AKI can prolong hospitalizations, increase the cost of health care, and increase morbidity.2
In March 2020, the American Society of Health-System Pharmacists, the Infectious Diseases Society of America (IDSA), the Pediatric Infectious Disease Society, and the Society of Infectious Diseases Pharmacists released a consensus statement and guidelines regarding the optimization of vancomycin dosing and monitoring for patients with suspected or definitive serious MRSA infections. Based on these guidelines, it is recommended to target an individualized area under the curve/minimum inhibitory concentration (AUC/MIC) ratio of 400 to 600 mg × h/L to maximize clinical efficacy and minimize the risk of AKI.2
Before March 2020, the vancomycin monitoring recommendation was to target trough levels of 10 to 20 mg/L. A goal trough of 15 to 20 mg/L was recommended for severe infections, including sepsis, endocarditis, hospital-acquired pneumonia, meningitis, and osteomyelitis, caused by MRSA. A goal trough of 10 to 15 mg/L was recommended for noninvasive infections, such as skin and soft tissue infections and urinary tract infections, caused by MRSA. Targeting these trough levels was thought to achieve an AUC/MIC ≥ 400 mg × h/L.5 Evidence has since shown that trough values may not be an optimal marker for AUC/MIC values.2
The updated vancomycin therapeutic drug monitoring (TDM) guidelines recommend that health systems transition to AUC/MIC-guided monitoring for suspected or confirmed infections caused by MRSA. There is not enough evidence to recommend AUC/MIC-guided monitoring in patients with noninvasive infections or infections caused by other microbes.2
AUC/MIC-guided monitoring can be achieved in 2 ways. The first method is collecting Cmax (peak level) and Cmin (trough level) serum concentrations, preferably during the same dosing interval. Ideally, Cmax should be drawn 1 to 2 hours after the vancomycin infusion and Cmin should be drawn at the end of the dosing interval. First-order pharmacokinetic equations are used to estimate the AUC/MIC with this method. Bayesian software pharmacokinetic modeling based on 1 or 2 vancomycin concentrations with 1 trough level also can be used for monitoring. Preferably, 2 levels would be obtained to estimate the AUC/MIC when using Bayesian modeling.2
The bactericidal activity of vancomycin was achieved with AUC/MIC ratios of ≥ 400 mg × h/L. AUC/MIC ratios of < 400 mg × h/L increase the incidence of resistant and intermediate strains of S aureus. AUC/MIC-guided monitoring assumes an MIC of 1 mg/L. When the MIC is > 1 mg/L, it is less likely that an AUC/MIC ≥ 400 mg × h/L is achievable. Regardless of the TDM method used, AUC/MIC ratios ≥ 400 mg × h/L are not achievable with conventional dosing methods if the vancomycin MIC is > 2 mg/L in patients with normal renal function. Alternative therapy is recommended to be used for these patients.2
There are multiple studies investigating the therapeutic dosing of vancomycin and the associated incidence of AKI. Previous studies have correlated vancomycin AUC/MICs of 400 mg to 600 mg × h/L with clinical effectiveness.2,6 In 2017, Neely and colleagues looked at the therapeutic dosing of vancomycin in 252 adults with ≥ 1 vancomycin level.7 During this prospective trial, they evaluated patients for 1 year and targeted trough concentrations of 10 to 20 mg/L with infection-specific goal ranges of 10 to 15 mg/L and 15 to 20 mg/L for noninvasive and invasive infections, respectively. They also targeted AUC/MIC ratios ≥ 400 mg × h/L regardless of trough concentration using Bayesian estimated AUC/MICs for 2 years. They found only 19% of trough concentrations to be therapeutic compared with 70% of AUC/MICs. A secondary outcome assessed by Neely and colleagues was nephrotoxicity, which was identified in 8% of patients with trough targets and 2% of patients with AUC/MIC targets.8
Previous studies evaluating the use of vancomycin in the veteran population have focused on AKI incidence, general nephrotoxicity, and 30-day readmission rates.4,7,9,10 Poston-Blahnik and colleagues investigated the rates of AKI in 200 veterans using AUC/MIC-guided vancomycin TDM.5 They found an AKI incidence of 42% of patients with AUC/MICs ≥ 550 mg × h/L and 2% of patients with AUC/MICs < 550 mg × h/L.5 Gyamlani and colleagues investigated the rates of AKI in 33,527 veterans and found that serum vancomycin trough levels ≥ 20 mg/L were associated with a higher risk of AKI.8 Prabaker and colleagues investigated the association between vancomycin trough levels and nephrotoxicity, defined as 0.5 mg/L or a 50% increase in serum creatinine (sCr) in 348 veterans. They found nephrotoxicity in 8.9% of patients.10 Patel and colleagues investigated the effect of AKI on 30-day readmission rates in 216 veterans.10 AKI occurred in 8.8% of patients and of those 19.4% were readmitted within 30 days.10 Current literature lacks evidence regarding the comparison of the safety and efficacy of vancomycin trough-guided vs AUC/MIC-guided TDM in the veteran population. Therefore, the objective of this study was to investigate the differences in the safety and efficacy of vancomycin TDM in the veteran population based on the different monitoring methods used.
METHODS
This study was a retrospective, single-center, quasi-experimental chart review conducted at the Sioux Falls Veterans Affairs Health Care System (SFVAHCS) in South Dakota. Data were collected from the Computerized Patient Record System (CPRS). The SFVAHCS transitioned from trough-guided to AUC/MIC-guided TDM in November 2020.
Patients included in this study were veterans aged ≥ 18 years with orders for parenteral vancomycin between February 1, 2020, and October 31, 2020, for the trough-guided TDM group and between December 1, 2020, and August 31, 2021, for the AUC/MIC-guided TDM group. Patients with vancomycin courses initiated during November 2020 were excluded as both TDM methods were being used at that time. Patients were excluded if their vancomycin course began before February 1, 2020, for the trough-guided TDM group or began during November 2020 for the AUC/MIC-guided TDM group. Patients were excluded if their vancomycin course extended past October 31, 2020, for the trough group or past August 31, 2021, for the AUC/MIC group. Patients on dialysis or missing Cmax, Cmin, or sCr levels were excluded.
This study evaluated both safety (AKI incidence) and effectiveness (time spent in therapeutic range and time to therapeutic range). The primary endpoint was presence of vancomycin-induced AKI, which was based on the most recent Kidney Disease: Improving Global Outcomes (KDIGO) AKI definition: increased sCr of ≥ 0.3 mg/dL or by 50% from baseline sustained over 48 hours without any other explanation for the change.11 A secondary endpoint was the absence or presence of AKI.
Additional secondary endpoints included the presence of the initial trough or AUC/MIC of each vancomycin course within the therapeutic range and the percentage of all trough levels or AUC/MICs within therapeutic, subtherapeutic, and supratherapeutic ranges. The therapeutic range for AUC/MIC-guided TDM was 400 to 600 mg × h/L and 10 to 20 mg/L depending on indication for trough-guided TDM (15-20 mg/L for severe infections and 10-15 mg/L for less invasive infections). The percentage of trough levels or AUC/MICs within therapeutic, subtherapeutic, and supratherapeutic ranges were calculated as a ratio of levels within each range to total levels taken for each patient.
For AUC/MIC-guided TDM the Cmax levels were ideally drawn 1 to 2 hours after vancomycin infusion and Cmin levels were ideally drawn 30 minutes before the next dose. First-order pharmacokinetic equations were used to estimate the AUC/MIC.12 If the timing of a vancomycin level was inappropriate, actual levels were extrapolated based on the timing of the blood draw compared with the ideal Cmin or Cmax time. Extrapolated levels were used for both trough-guided and AUC/MIC-guided TDM groups when appropriate. Vancomycin levels were excluded if they were drawn during the vancomycin infusion.
Study participant age, sex, race, weight, baseline estimated glomerular filtration (eGFR) rate, baseline sCr, concomitant nephrotoxic medications, duration of vancomycin course, indication of vancomycin, and acuity of illness based on indication were collected. sCr levels were collected from the initial day vancomycin was ordered through 72 hours following completion of a vancomycin course to evaluate for AKI. Patients’ charts were reviewed for the use of the following nephrotoxic medications: nonsteroidal anti-inflammatories, angiotensin-converting enzyme inhibitors, angiotensin II receptor blockers, aminoglycosides, piperacillin/tazobactam, loop diuretics, amphotericin B, acyclovir, intravenous contrast, and nephrotoxic chemotherapy (cisplatin). The category of concomitant nephrotoxic medications was also collected including the continuation of a home nephrotoxic medication vs the initiation of a new nephrotoxic medication.
Statistical Analysis
The primary endpoint of the incidence of vancomycin-induced AKI was compared using a Fisher exact test. The secondary endpoint of the percentage of trough levels or AUC/MICs in the therapeutic, subtherapeutic, and supratherapeutic range were compared using a student t test. The secondary endpoint of first level or AUC/MIC within goal range was compared using a χ2 test. Continuous baseline characteristics were reported as a mean and compared using a student t test. Nominal baseline characteristics were reported as a percentage and compared using the χ2 test. P values < .05 were considered statistically significant.
RESULTS
This study included 97 patients, 43 in the AUC/MIC group and 54 in the trough group.
One (2%) patient in the AUC/MIC group and 2 (4%) patients in the trough group experienced vancomycin-induced AKI (P = .10) (Table 2).
DISCUSSION
There was no statistically significant difference between the 2 groups for the vancomycin-induced AKI (P = .10), the primary endpoint, or overall AKI (P = .29), the secondary endpoint. It should be noted that there was more overall AKI in the AUC/MIC group. Veterans in the AUC/MIC group were found to have their first AUC/MIC within the therapeutic range statistically significantly more often than the first trough level in the trough group (P = .04). The percentage of time spent within therapeutic range was statistically significantly higher in the AUC/MIC-guided TDM group (P = .02). The percentage of time spent subtherapeutic of goal range was statistically significantly higher in the trough-guided TDM group (P < .001). There was no statistically significant difference found in the percent of time spent supratherapeutic of goal range (P = .25). However, the observed percentage of time spent supratherapeutic of goal range was higher in the AUC/MIC group. These results indicate that AUC/MIC-guided TDM may be more efficacious with regard to time in therapeutic range and time to therapeutic range.
The finding of increased AKI with AUC/MIC-guided TDM does not align with previous studies.8 The prospective study by Neely and colleagues found that AUC/MIC-guided TDM resulted in more time in the therapeutic range as well as less nephrotoxicity compared with trough-guided TDM, although it was limited by its lack of randomization and did not account for other causes of nephrotoxicity.8 They found that only 19% of trough concentrations were therapeutic compared with 70% of AUC/MICs and found nephrotoxicity in 8% of trough-guided TDM patients compared with 2% of AUC/MIC-guided TDM patients.8
Unlike Nealy and colleagues, our study did not find lower nephrotoxicity associated with AUC/MIC-guided TDM. Multiple factors may have influenced our results. Our AUC/MIC group had significantly more newly started concomitant nephrotoxins and other nephrotoxic medications used during the vancomycin courses compared with the trough-guided group, which may have influenced AKI outcomes. It also should be noted that there was significantly more time spent subtherapeutic of the goal range and significantly less time in the goal range in the trough group compared with the AUC/MIC group. In our study, the trough-guided group had significantly more patients with acute illness compared with the AUC/MIC group (skin, soft tissue, and joint infections were similar between the groups). The group with more acutely ill patients would have been expected to have more nephrotoxicity. However, despite the acute illnesses, patients in the trough-guided group spent more time in the subtherapeutic range. This may explain the increased nephrotoxicity in the AUC/MIC group since those patients spent more time in the therapeutic range.
This study used the most recent KDIGO AKI definition: either an increase in sCr of ≥ 0.3 mg/dL or a 50% increase in sCr from baseline sustained over 48 hours without any other explanation for the change in renal function.11 This AKI definition is stricter than the previous definition, which was used by earlier studies, including Neely and colleagues, to evaluate rates of vancomycin-induced AKI.2,3 Therefore, the rates of overall AKI found in this study may be higher than in previous studies due to the definition of AKI used.
Limitations
This study was limited by its retrospective nature, lack of randomization, and small sample size. To decrease the potential for error in this study, analysis of power and a larger study sample would have been beneficial. During the COVID-19 pandemic, increased pneumonia cases may have hidden bacterial causes and caused an undercount. Nephrotoxicity may also be related to volume depletion, severe systemic illness, dehydration, or hypotension. Screening was completed via chart review for these alternative causes of nephrotoxicity in this study but may not be completely accounted for due to lack of documentation and the retrospective nature of this study.
CONCLUSIONS
This study did not find a significant difference in the rates of vancomycin-induced or overall AKI between AUC/MIC-guided and trough-guided TDM. However, this study may not have been powered to detect a significant difference in the primary endpoint. This study indicated that AUC/MIC-guided TDM of vancomycin resulted in a quicker time to the therapeutic range and a higher percentage of overall time in the therapeutic range as compared with trough-guided TDM. The results of this study indicated that trough-guided monitoring resulted in a higher percentage of time in a subtherapeutic range. This study also found that the first AUC/MIC calculated was within therapeutic range more often than the first trough level collected.
These results indicate that AUC/MIC-guided TDM may be more effective than trough-guided TDM in the veteran population. However, while AUC/MIC-guided TDM may be more effective with regards to time in therapeutic range and time to therapeutic range, this study did not indicate any safety benefit of AUC/MIC-guided over trough-guided TDM with regards to AKI incidence. Our data indicate that AUC/MIC-guided TDM increases the amount of time in the therapeutic range compared with trough-guided TDM and is not more nephrotoxic. The findings of this study support the recommendation to transition to the use of AUC/MIC-guided TDM of vancomycin in the veteran population.
Acknowledgments
This material is the result of work supported with the use of facilities and resources from the Sioux Falls Veterans Affairs Health Care System.
1. Gallagher J, MacDougall C. Glycopeptides and short-acting lipoglycopeptides In: Antibiotics Simplified. Jones & Bartlett Learning; 2018.
2. Rybak MJ, Le J, Lodise TP, et al. Therapeutic monitoring of vancomycin for serious methicillin-resistant Staphylococcus aureus infections: a revised consensus guideline and review by the American Society of Health-System Pharmacists, the Infectious Diseases Society of America, the Pediatric Infectious Diseases Society, and the Society of Infectious Diseases Pharmacists. Am J Health Syst Pharm. 2020;77(11):835-864. doi:10.1093/ajhp/zxaa036
3. Hermsen ED, Hanson M, Sankaranarayanan J, Stoner JA, Florescu MC, Rupp ME. Clinical outcomes and nephrotoxicity associated with vancomycin trough concentrations during treatment of deep-seated infections. Expert Opin Drug Saf. 2010;9(1):9-14. doi:10.1517/14740330903413514
4. Poston-Blahnik A, Moenster R. Association between vancomycin area under the curve and nephrotoxicity: a single center, retrospective cohort study in a veteran population. Open Forum Infect Dis. 2021;8(5):ofab094. Published 2021 Mar 12. doi:10.1093/ofid/ofab094
5. Rybak M, Lomaestro B, Rotschafer JC, et al. Therapeutic monitoring of vancomycin in adult patients: a consensus review of the American Society of Health-System Pharmacists, the Infectious Diseases Society of America, and the Society of Infectious Diseases Pharmacists. Am J Health Syst Pharm. 2009;66(1):82-98. doi:10.2146/ajhp080434
6. Moise-Broder PA, Forrest A, Birmingham MC, Schentag JJ. Pharmacodynamics of vancomycin and other antimicrobials in patients with Staphylococcus aureus lower respiratory tract infections. Clin Pharmacokinet. 2004;43(13):925-942. doi:10.2165/00003088-200443130-00005
7. Gyamlani G, Potukuchi PK, Thomas F, et al. Vancomycin-Associated Acute Kidney Injury in a Large Veteran Population. Am J Nephrol. 2019;49(2):133-142. doi:10.1159/000496484
8. Neely MN, Kato L, Youn G, et al. Prospective Trial on the Use of Trough Concentration versus Area under the Curve To Determine Therapeutic Vancomycin Dosing. Antimicrob Agents Chemother. 2018;62(2):e02042-17. Published 2018 Jan 25. doi:10.1128/AAC.02042-17
9. Prabaker KK, Tran TP, Pratummas T, Goetz MB, Graber CJ. Elevated vancomycin trough is not associated with nephrotoxicity among inpatient veterans. J Hosp Med. 2012;7(2):91-97. doi:10.1002/jhm.946
10. Patel N, Stornelli N, Sangiovanni RJ, Huang DB, Lodise TP. Effect of vancomycin-associated acute kidney injury on incidence of 30-day readmissions among hospitalized Veterans Affairs patients with skin and skin structure infections. Antimicrob Agents Chemother. 2020;64(10):e01268-20. Published 2020 Sep 21. doi:10.1128/AAC.01268-20
11. Acute Kidney Injury Work Group. Kidney Disease: Improving Global Outcomes (KDIGO) Clinical Practice Guideline for Acute Kidney Injury. Kidney Int. 2012;2(suppl 1):1-138.
12. Pai MP, Neely M, Rodvold KA, Lodise TP. Innovative approaches to optimizing the delivery of vancomycin in individual patients. Adv Drug Deliv Rev. 2014;77:50-57. doi:10.1016/j.addr.2014.05.016
Vancomycin is a commonly used glycopeptide antibiotic used to treat infections caused by gram-positive organisms. Vancomycin is most often used as a parenteral agent for empiric or definitive treatment of methicillin-resistant Staphylococcus aureus (MRSA). It can also be used for the treatment of other susceptible Staphylococcus or Enterococcus species. Adverse effects of parenteral vancomycin include infusion-related reactions, ototoxicity, and nephrotoxicity.1 Higher vancomycin trough levels have been associated with an increased risk of nephrotoxicity.1-4 The major safety concern with vancomycin is acute kidney injury (AKI). Even mild AKI can prolong hospitalizations, increase the cost of health care, and increase morbidity.2
In March 2020, the American Society of Health-System Pharmacists, the Infectious Diseases Society of America (IDSA), the Pediatric Infectious Disease Society, and the Society of Infectious Diseases Pharmacists released a consensus statement and guidelines regarding the optimization of vancomycin dosing and monitoring for patients with suspected or definitive serious MRSA infections. Based on these guidelines, it is recommended to target an individualized area under the curve/minimum inhibitory concentration (AUC/MIC) ratio of 400 to 600 mg × h/L to maximize clinical efficacy and minimize the risk of AKI.2
Before March 2020, the vancomycin monitoring recommendation was to target trough levels of 10 to 20 mg/L. A goal trough of 15 to 20 mg/L was recommended for severe infections, including sepsis, endocarditis, hospital-acquired pneumonia, meningitis, and osteomyelitis, caused by MRSA. A goal trough of 10 to 15 mg/L was recommended for noninvasive infections, such as skin and soft tissue infections and urinary tract infections, caused by MRSA. Targeting these trough levels was thought to achieve an AUC/MIC ≥ 400 mg × h/L.5 Evidence has since shown that trough values may not be an optimal marker for AUC/MIC values.2
The updated vancomycin therapeutic drug monitoring (TDM) guidelines recommend that health systems transition to AUC/MIC-guided monitoring for suspected or confirmed infections caused by MRSA. There is not enough evidence to recommend AUC/MIC-guided monitoring in patients with noninvasive infections or infections caused by other microbes.2
AUC/MIC-guided monitoring can be achieved in 2 ways. The first method is collecting Cmax (peak level) and Cmin (trough level) serum concentrations, preferably during the same dosing interval. Ideally, Cmax should be drawn 1 to 2 hours after the vancomycin infusion and Cmin should be drawn at the end of the dosing interval. First-order pharmacokinetic equations are used to estimate the AUC/MIC with this method. Bayesian software pharmacokinetic modeling based on 1 or 2 vancomycin concentrations with 1 trough level also can be used for monitoring. Preferably, 2 levels would be obtained to estimate the AUC/MIC when using Bayesian modeling.2
The bactericidal activity of vancomycin was achieved with AUC/MIC ratios of ≥ 400 mg × h/L. AUC/MIC ratios of < 400 mg × h/L increase the incidence of resistant and intermediate strains of S aureus. AUC/MIC-guided monitoring assumes an MIC of 1 mg/L. When the MIC is > 1 mg/L, it is less likely that an AUC/MIC ≥ 400 mg × h/L is achievable. Regardless of the TDM method used, AUC/MIC ratios ≥ 400 mg × h/L are not achievable with conventional dosing methods if the vancomycin MIC is > 2 mg/L in patients with normal renal function. Alternative therapy is recommended to be used for these patients.2
There are multiple studies investigating the therapeutic dosing of vancomycin and the associated incidence of AKI. Previous studies have correlated vancomycin AUC/MICs of 400 mg to 600 mg × h/L with clinical effectiveness.2,6 In 2017, Neely and colleagues looked at the therapeutic dosing of vancomycin in 252 adults with ≥ 1 vancomycin level.7 During this prospective trial, they evaluated patients for 1 year and targeted trough concentrations of 10 to 20 mg/L with infection-specific goal ranges of 10 to 15 mg/L and 15 to 20 mg/L for noninvasive and invasive infections, respectively. They also targeted AUC/MIC ratios ≥ 400 mg × h/L regardless of trough concentration using Bayesian estimated AUC/MICs for 2 years. They found only 19% of trough concentrations to be therapeutic compared with 70% of AUC/MICs. A secondary outcome assessed by Neely and colleagues was nephrotoxicity, which was identified in 8% of patients with trough targets and 2% of patients with AUC/MIC targets.8
Previous studies evaluating the use of vancomycin in the veteran population have focused on AKI incidence, general nephrotoxicity, and 30-day readmission rates.4,7,9,10 Poston-Blahnik and colleagues investigated the rates of AKI in 200 veterans using AUC/MIC-guided vancomycin TDM.5 They found an AKI incidence of 42% of patients with AUC/MICs ≥ 550 mg × h/L and 2% of patients with AUC/MICs < 550 mg × h/L.5 Gyamlani and colleagues investigated the rates of AKI in 33,527 veterans and found that serum vancomycin trough levels ≥ 20 mg/L were associated with a higher risk of AKI.8 Prabaker and colleagues investigated the association between vancomycin trough levels and nephrotoxicity, defined as 0.5 mg/L or a 50% increase in serum creatinine (sCr) in 348 veterans. They found nephrotoxicity in 8.9% of patients.10 Patel and colleagues investigated the effect of AKI on 30-day readmission rates in 216 veterans.10 AKI occurred in 8.8% of patients and of those 19.4% were readmitted within 30 days.10 Current literature lacks evidence regarding the comparison of the safety and efficacy of vancomycin trough-guided vs AUC/MIC-guided TDM in the veteran population. Therefore, the objective of this study was to investigate the differences in the safety and efficacy of vancomycin TDM in the veteran population based on the different monitoring methods used.
METHODS
This study was a retrospective, single-center, quasi-experimental chart review conducted at the Sioux Falls Veterans Affairs Health Care System (SFVAHCS) in South Dakota. Data were collected from the Computerized Patient Record System (CPRS). The SFVAHCS transitioned from trough-guided to AUC/MIC-guided TDM in November 2020.
Patients included in this study were veterans aged ≥ 18 years with orders for parenteral vancomycin between February 1, 2020, and October 31, 2020, for the trough-guided TDM group and between December 1, 2020, and August 31, 2021, for the AUC/MIC-guided TDM group. Patients with vancomycin courses initiated during November 2020 were excluded as both TDM methods were being used at that time. Patients were excluded if their vancomycin course began before February 1, 2020, for the trough-guided TDM group or began during November 2020 for the AUC/MIC-guided TDM group. Patients were excluded if their vancomycin course extended past October 31, 2020, for the trough group or past August 31, 2021, for the AUC/MIC group. Patients on dialysis or missing Cmax, Cmin, or sCr levels were excluded.
This study evaluated both safety (AKI incidence) and effectiveness (time spent in therapeutic range and time to therapeutic range). The primary endpoint was presence of vancomycin-induced AKI, which was based on the most recent Kidney Disease: Improving Global Outcomes (KDIGO) AKI definition: increased sCr of ≥ 0.3 mg/dL or by 50% from baseline sustained over 48 hours without any other explanation for the change.11 A secondary endpoint was the absence or presence of AKI.
Additional secondary endpoints included the presence of the initial trough or AUC/MIC of each vancomycin course within the therapeutic range and the percentage of all trough levels or AUC/MICs within therapeutic, subtherapeutic, and supratherapeutic ranges. The therapeutic range for AUC/MIC-guided TDM was 400 to 600 mg × h/L and 10 to 20 mg/L depending on indication for trough-guided TDM (15-20 mg/L for severe infections and 10-15 mg/L for less invasive infections). The percentage of trough levels or AUC/MICs within therapeutic, subtherapeutic, and supratherapeutic ranges were calculated as a ratio of levels within each range to total levels taken for each patient.
For AUC/MIC-guided TDM the Cmax levels were ideally drawn 1 to 2 hours after vancomycin infusion and Cmin levels were ideally drawn 30 minutes before the next dose. First-order pharmacokinetic equations were used to estimate the AUC/MIC.12 If the timing of a vancomycin level was inappropriate, actual levels were extrapolated based on the timing of the blood draw compared with the ideal Cmin or Cmax time. Extrapolated levels were used for both trough-guided and AUC/MIC-guided TDM groups when appropriate. Vancomycin levels were excluded if they were drawn during the vancomycin infusion.
Study participant age, sex, race, weight, baseline estimated glomerular filtration (eGFR) rate, baseline sCr, concomitant nephrotoxic medications, duration of vancomycin course, indication of vancomycin, and acuity of illness based on indication were collected. sCr levels were collected from the initial day vancomycin was ordered through 72 hours following completion of a vancomycin course to evaluate for AKI. Patients’ charts were reviewed for the use of the following nephrotoxic medications: nonsteroidal anti-inflammatories, angiotensin-converting enzyme inhibitors, angiotensin II receptor blockers, aminoglycosides, piperacillin/tazobactam, loop diuretics, amphotericin B, acyclovir, intravenous contrast, and nephrotoxic chemotherapy (cisplatin). The category of concomitant nephrotoxic medications was also collected including the continuation of a home nephrotoxic medication vs the initiation of a new nephrotoxic medication.
Statistical Analysis
The primary endpoint of the incidence of vancomycin-induced AKI was compared using a Fisher exact test. The secondary endpoint of the percentage of trough levels or AUC/MICs in the therapeutic, subtherapeutic, and supratherapeutic range were compared using a student t test. The secondary endpoint of first level or AUC/MIC within goal range was compared using a χ2 test. Continuous baseline characteristics were reported as a mean and compared using a student t test. Nominal baseline characteristics were reported as a percentage and compared using the χ2 test. P values < .05 were considered statistically significant.
RESULTS
This study included 97 patients, 43 in the AUC/MIC group and 54 in the trough group.
One (2%) patient in the AUC/MIC group and 2 (4%) patients in the trough group experienced vancomycin-induced AKI (P = .10) (Table 2).
DISCUSSION
There was no statistically significant difference between the 2 groups for the vancomycin-induced AKI (P = .10), the primary endpoint, or overall AKI (P = .29), the secondary endpoint. It should be noted that there was more overall AKI in the AUC/MIC group. Veterans in the AUC/MIC group were found to have their first AUC/MIC within the therapeutic range statistically significantly more often than the first trough level in the trough group (P = .04). The percentage of time spent within therapeutic range was statistically significantly higher in the AUC/MIC-guided TDM group (P = .02). The percentage of time spent subtherapeutic of goal range was statistically significantly higher in the trough-guided TDM group (P < .001). There was no statistically significant difference found in the percent of time spent supratherapeutic of goal range (P = .25). However, the observed percentage of time spent supratherapeutic of goal range was higher in the AUC/MIC group. These results indicate that AUC/MIC-guided TDM may be more efficacious with regard to time in therapeutic range and time to therapeutic range.
The finding of increased AKI with AUC/MIC-guided TDM does not align with previous studies.8 The prospective study by Neely and colleagues found that AUC/MIC-guided TDM resulted in more time in the therapeutic range as well as less nephrotoxicity compared with trough-guided TDM, although it was limited by its lack of randomization and did not account for other causes of nephrotoxicity.8 They found that only 19% of trough concentrations were therapeutic compared with 70% of AUC/MICs and found nephrotoxicity in 8% of trough-guided TDM patients compared with 2% of AUC/MIC-guided TDM patients.8
Unlike Nealy and colleagues, our study did not find lower nephrotoxicity associated with AUC/MIC-guided TDM. Multiple factors may have influenced our results. Our AUC/MIC group had significantly more newly started concomitant nephrotoxins and other nephrotoxic medications used during the vancomycin courses compared with the trough-guided group, which may have influenced AKI outcomes. It also should be noted that there was significantly more time spent subtherapeutic of the goal range and significantly less time in the goal range in the trough group compared with the AUC/MIC group. In our study, the trough-guided group had significantly more patients with acute illness compared with the AUC/MIC group (skin, soft tissue, and joint infections were similar between the groups). The group with more acutely ill patients would have been expected to have more nephrotoxicity. However, despite the acute illnesses, patients in the trough-guided group spent more time in the subtherapeutic range. This may explain the increased nephrotoxicity in the AUC/MIC group since those patients spent more time in the therapeutic range.
This study used the most recent KDIGO AKI definition: either an increase in sCr of ≥ 0.3 mg/dL or a 50% increase in sCr from baseline sustained over 48 hours without any other explanation for the change in renal function.11 This AKI definition is stricter than the previous definition, which was used by earlier studies, including Neely and colleagues, to evaluate rates of vancomycin-induced AKI.2,3 Therefore, the rates of overall AKI found in this study may be higher than in previous studies due to the definition of AKI used.
Limitations
This study was limited by its retrospective nature, lack of randomization, and small sample size. To decrease the potential for error in this study, analysis of power and a larger study sample would have been beneficial. During the COVID-19 pandemic, increased pneumonia cases may have hidden bacterial causes and caused an undercount. Nephrotoxicity may also be related to volume depletion, severe systemic illness, dehydration, or hypotension. Screening was completed via chart review for these alternative causes of nephrotoxicity in this study but may not be completely accounted for due to lack of documentation and the retrospective nature of this study.
CONCLUSIONS
This study did not find a significant difference in the rates of vancomycin-induced or overall AKI between AUC/MIC-guided and trough-guided TDM. However, this study may not have been powered to detect a significant difference in the primary endpoint. This study indicated that AUC/MIC-guided TDM of vancomycin resulted in a quicker time to the therapeutic range and a higher percentage of overall time in the therapeutic range as compared with trough-guided TDM. The results of this study indicated that trough-guided monitoring resulted in a higher percentage of time in a subtherapeutic range. This study also found that the first AUC/MIC calculated was within therapeutic range more often than the first trough level collected.
These results indicate that AUC/MIC-guided TDM may be more effective than trough-guided TDM in the veteran population. However, while AUC/MIC-guided TDM may be more effective with regards to time in therapeutic range and time to therapeutic range, this study did not indicate any safety benefit of AUC/MIC-guided over trough-guided TDM with regards to AKI incidence. Our data indicate that AUC/MIC-guided TDM increases the amount of time in the therapeutic range compared with trough-guided TDM and is not more nephrotoxic. The findings of this study support the recommendation to transition to the use of AUC/MIC-guided TDM of vancomycin in the veteran population.
Acknowledgments
This material is the result of work supported with the use of facilities and resources from the Sioux Falls Veterans Affairs Health Care System.
Vancomycin is a commonly used glycopeptide antibiotic used to treat infections caused by gram-positive organisms. Vancomycin is most often used as a parenteral agent for empiric or definitive treatment of methicillin-resistant Staphylococcus aureus (MRSA). It can also be used for the treatment of other susceptible Staphylococcus or Enterococcus species. Adverse effects of parenteral vancomycin include infusion-related reactions, ototoxicity, and nephrotoxicity.1 Higher vancomycin trough levels have been associated with an increased risk of nephrotoxicity.1-4 The major safety concern with vancomycin is acute kidney injury (AKI). Even mild AKI can prolong hospitalizations, increase the cost of health care, and increase morbidity.2
In March 2020, the American Society of Health-System Pharmacists, the Infectious Diseases Society of America (IDSA), the Pediatric Infectious Disease Society, and the Society of Infectious Diseases Pharmacists released a consensus statement and guidelines regarding the optimization of vancomycin dosing and monitoring for patients with suspected or definitive serious MRSA infections. Based on these guidelines, it is recommended to target an individualized area under the curve/minimum inhibitory concentration (AUC/MIC) ratio of 400 to 600 mg × h/L to maximize clinical efficacy and minimize the risk of AKI.2
Before March 2020, the vancomycin monitoring recommendation was to target trough levels of 10 to 20 mg/L. A goal trough of 15 to 20 mg/L was recommended for severe infections, including sepsis, endocarditis, hospital-acquired pneumonia, meningitis, and osteomyelitis, caused by MRSA. A goal trough of 10 to 15 mg/L was recommended for noninvasive infections, such as skin and soft tissue infections and urinary tract infections, caused by MRSA. Targeting these trough levels was thought to achieve an AUC/MIC ≥ 400 mg × h/L.5 Evidence has since shown that trough values may not be an optimal marker for AUC/MIC values.2
The updated vancomycin therapeutic drug monitoring (TDM) guidelines recommend that health systems transition to AUC/MIC-guided monitoring for suspected or confirmed infections caused by MRSA. There is not enough evidence to recommend AUC/MIC-guided monitoring in patients with noninvasive infections or infections caused by other microbes.2
AUC/MIC-guided monitoring can be achieved in 2 ways. The first method is collecting Cmax (peak level) and Cmin (trough level) serum concentrations, preferably during the same dosing interval. Ideally, Cmax should be drawn 1 to 2 hours after the vancomycin infusion and Cmin should be drawn at the end of the dosing interval. First-order pharmacokinetic equations are used to estimate the AUC/MIC with this method. Bayesian software pharmacokinetic modeling based on 1 or 2 vancomycin concentrations with 1 trough level also can be used for monitoring. Preferably, 2 levels would be obtained to estimate the AUC/MIC when using Bayesian modeling.2
The bactericidal activity of vancomycin was achieved with AUC/MIC ratios of ≥ 400 mg × h/L. AUC/MIC ratios of < 400 mg × h/L increase the incidence of resistant and intermediate strains of S aureus. AUC/MIC-guided monitoring assumes an MIC of 1 mg/L. When the MIC is > 1 mg/L, it is less likely that an AUC/MIC ≥ 400 mg × h/L is achievable. Regardless of the TDM method used, AUC/MIC ratios ≥ 400 mg × h/L are not achievable with conventional dosing methods if the vancomycin MIC is > 2 mg/L in patients with normal renal function. Alternative therapy is recommended to be used for these patients.2
There are multiple studies investigating the therapeutic dosing of vancomycin and the associated incidence of AKI. Previous studies have correlated vancomycin AUC/MICs of 400 mg to 600 mg × h/L with clinical effectiveness.2,6 In 2017, Neely and colleagues looked at the therapeutic dosing of vancomycin in 252 adults with ≥ 1 vancomycin level.7 During this prospective trial, they evaluated patients for 1 year and targeted trough concentrations of 10 to 20 mg/L with infection-specific goal ranges of 10 to 15 mg/L and 15 to 20 mg/L for noninvasive and invasive infections, respectively. They also targeted AUC/MIC ratios ≥ 400 mg × h/L regardless of trough concentration using Bayesian estimated AUC/MICs for 2 years. They found only 19% of trough concentrations to be therapeutic compared with 70% of AUC/MICs. A secondary outcome assessed by Neely and colleagues was nephrotoxicity, which was identified in 8% of patients with trough targets and 2% of patients with AUC/MIC targets.8
Previous studies evaluating the use of vancomycin in the veteran population have focused on AKI incidence, general nephrotoxicity, and 30-day readmission rates.4,7,9,10 Poston-Blahnik and colleagues investigated the rates of AKI in 200 veterans using AUC/MIC-guided vancomycin TDM.5 They found an AKI incidence of 42% of patients with AUC/MICs ≥ 550 mg × h/L and 2% of patients with AUC/MICs < 550 mg × h/L.5 Gyamlani and colleagues investigated the rates of AKI in 33,527 veterans and found that serum vancomycin trough levels ≥ 20 mg/L were associated with a higher risk of AKI.8 Prabaker and colleagues investigated the association between vancomycin trough levels and nephrotoxicity, defined as 0.5 mg/L or a 50% increase in serum creatinine (sCr) in 348 veterans. They found nephrotoxicity in 8.9% of patients.10 Patel and colleagues investigated the effect of AKI on 30-day readmission rates in 216 veterans.10 AKI occurred in 8.8% of patients and of those 19.4% were readmitted within 30 days.10 Current literature lacks evidence regarding the comparison of the safety and efficacy of vancomycin trough-guided vs AUC/MIC-guided TDM in the veteran population. Therefore, the objective of this study was to investigate the differences in the safety and efficacy of vancomycin TDM in the veteran population based on the different monitoring methods used.
METHODS
This study was a retrospective, single-center, quasi-experimental chart review conducted at the Sioux Falls Veterans Affairs Health Care System (SFVAHCS) in South Dakota. Data were collected from the Computerized Patient Record System (CPRS). The SFVAHCS transitioned from trough-guided to AUC/MIC-guided TDM in November 2020.
Patients included in this study were veterans aged ≥ 18 years with orders for parenteral vancomycin between February 1, 2020, and October 31, 2020, for the trough-guided TDM group and between December 1, 2020, and August 31, 2021, for the AUC/MIC-guided TDM group. Patients with vancomycin courses initiated during November 2020 were excluded as both TDM methods were being used at that time. Patients were excluded if their vancomycin course began before February 1, 2020, for the trough-guided TDM group or began during November 2020 for the AUC/MIC-guided TDM group. Patients were excluded if their vancomycin course extended past October 31, 2020, for the trough group or past August 31, 2021, for the AUC/MIC group. Patients on dialysis or missing Cmax, Cmin, or sCr levels were excluded.
This study evaluated both safety (AKI incidence) and effectiveness (time spent in therapeutic range and time to therapeutic range). The primary endpoint was presence of vancomycin-induced AKI, which was based on the most recent Kidney Disease: Improving Global Outcomes (KDIGO) AKI definition: increased sCr of ≥ 0.3 mg/dL or by 50% from baseline sustained over 48 hours without any other explanation for the change.11 A secondary endpoint was the absence or presence of AKI.
Additional secondary endpoints included the presence of the initial trough or AUC/MIC of each vancomycin course within the therapeutic range and the percentage of all trough levels or AUC/MICs within therapeutic, subtherapeutic, and supratherapeutic ranges. The therapeutic range for AUC/MIC-guided TDM was 400 to 600 mg × h/L and 10 to 20 mg/L depending on indication for trough-guided TDM (15-20 mg/L for severe infections and 10-15 mg/L for less invasive infections). The percentage of trough levels or AUC/MICs within therapeutic, subtherapeutic, and supratherapeutic ranges were calculated as a ratio of levels within each range to total levels taken for each patient.
For AUC/MIC-guided TDM the Cmax levels were ideally drawn 1 to 2 hours after vancomycin infusion and Cmin levels were ideally drawn 30 minutes before the next dose. First-order pharmacokinetic equations were used to estimate the AUC/MIC.12 If the timing of a vancomycin level was inappropriate, actual levels were extrapolated based on the timing of the blood draw compared with the ideal Cmin or Cmax time. Extrapolated levels were used for both trough-guided and AUC/MIC-guided TDM groups when appropriate. Vancomycin levels were excluded if they were drawn during the vancomycin infusion.
Study participant age, sex, race, weight, baseline estimated glomerular filtration (eGFR) rate, baseline sCr, concomitant nephrotoxic medications, duration of vancomycin course, indication of vancomycin, and acuity of illness based on indication were collected. sCr levels were collected from the initial day vancomycin was ordered through 72 hours following completion of a vancomycin course to evaluate for AKI. Patients’ charts were reviewed for the use of the following nephrotoxic medications: nonsteroidal anti-inflammatories, angiotensin-converting enzyme inhibitors, angiotensin II receptor blockers, aminoglycosides, piperacillin/tazobactam, loop diuretics, amphotericin B, acyclovir, intravenous contrast, and nephrotoxic chemotherapy (cisplatin). The category of concomitant nephrotoxic medications was also collected including the continuation of a home nephrotoxic medication vs the initiation of a new nephrotoxic medication.
Statistical Analysis
The primary endpoint of the incidence of vancomycin-induced AKI was compared using a Fisher exact test. The secondary endpoint of the percentage of trough levels or AUC/MICs in the therapeutic, subtherapeutic, and supratherapeutic range were compared using a student t test. The secondary endpoint of first level or AUC/MIC within goal range was compared using a χ2 test. Continuous baseline characteristics were reported as a mean and compared using a student t test. Nominal baseline characteristics were reported as a percentage and compared using the χ2 test. P values < .05 were considered statistically significant.
RESULTS
This study included 97 patients, 43 in the AUC/MIC group and 54 in the trough group.
One (2%) patient in the AUC/MIC group and 2 (4%) patients in the trough group experienced vancomycin-induced AKI (P = .10) (Table 2).
DISCUSSION
There was no statistically significant difference between the 2 groups for the vancomycin-induced AKI (P = .10), the primary endpoint, or overall AKI (P = .29), the secondary endpoint. It should be noted that there was more overall AKI in the AUC/MIC group. Veterans in the AUC/MIC group were found to have their first AUC/MIC within the therapeutic range statistically significantly more often than the first trough level in the trough group (P = .04). The percentage of time spent within therapeutic range was statistically significantly higher in the AUC/MIC-guided TDM group (P = .02). The percentage of time spent subtherapeutic of goal range was statistically significantly higher in the trough-guided TDM group (P < .001). There was no statistically significant difference found in the percent of time spent supratherapeutic of goal range (P = .25). However, the observed percentage of time spent supratherapeutic of goal range was higher in the AUC/MIC group. These results indicate that AUC/MIC-guided TDM may be more efficacious with regard to time in therapeutic range and time to therapeutic range.
The finding of increased AKI with AUC/MIC-guided TDM does not align with previous studies.8 The prospective study by Neely and colleagues found that AUC/MIC-guided TDM resulted in more time in the therapeutic range as well as less nephrotoxicity compared with trough-guided TDM, although it was limited by its lack of randomization and did not account for other causes of nephrotoxicity.8 They found that only 19% of trough concentrations were therapeutic compared with 70% of AUC/MICs and found nephrotoxicity in 8% of trough-guided TDM patients compared with 2% of AUC/MIC-guided TDM patients.8
Unlike Nealy and colleagues, our study did not find lower nephrotoxicity associated with AUC/MIC-guided TDM. Multiple factors may have influenced our results. Our AUC/MIC group had significantly more newly started concomitant nephrotoxins and other nephrotoxic medications used during the vancomycin courses compared with the trough-guided group, which may have influenced AKI outcomes. It also should be noted that there was significantly more time spent subtherapeutic of the goal range and significantly less time in the goal range in the trough group compared with the AUC/MIC group. In our study, the trough-guided group had significantly more patients with acute illness compared with the AUC/MIC group (skin, soft tissue, and joint infections were similar between the groups). The group with more acutely ill patients would have been expected to have more nephrotoxicity. However, despite the acute illnesses, patients in the trough-guided group spent more time in the subtherapeutic range. This may explain the increased nephrotoxicity in the AUC/MIC group since those patients spent more time in the therapeutic range.
This study used the most recent KDIGO AKI definition: either an increase in sCr of ≥ 0.3 mg/dL or a 50% increase in sCr from baseline sustained over 48 hours without any other explanation for the change in renal function.11 This AKI definition is stricter than the previous definition, which was used by earlier studies, including Neely and colleagues, to evaluate rates of vancomycin-induced AKI.2,3 Therefore, the rates of overall AKI found in this study may be higher than in previous studies due to the definition of AKI used.
Limitations
This study was limited by its retrospective nature, lack of randomization, and small sample size. To decrease the potential for error in this study, analysis of power and a larger study sample would have been beneficial. During the COVID-19 pandemic, increased pneumonia cases may have hidden bacterial causes and caused an undercount. Nephrotoxicity may also be related to volume depletion, severe systemic illness, dehydration, or hypotension. Screening was completed via chart review for these alternative causes of nephrotoxicity in this study but may not be completely accounted for due to lack of documentation and the retrospective nature of this study.
CONCLUSIONS
This study did not find a significant difference in the rates of vancomycin-induced or overall AKI between AUC/MIC-guided and trough-guided TDM. However, this study may not have been powered to detect a significant difference in the primary endpoint. This study indicated that AUC/MIC-guided TDM of vancomycin resulted in a quicker time to the therapeutic range and a higher percentage of overall time in the therapeutic range as compared with trough-guided TDM. The results of this study indicated that trough-guided monitoring resulted in a higher percentage of time in a subtherapeutic range. This study also found that the first AUC/MIC calculated was within therapeutic range more often than the first trough level collected.
These results indicate that AUC/MIC-guided TDM may be more effective than trough-guided TDM in the veteran population. However, while AUC/MIC-guided TDM may be more effective with regards to time in therapeutic range and time to therapeutic range, this study did not indicate any safety benefit of AUC/MIC-guided over trough-guided TDM with regards to AKI incidence. Our data indicate that AUC/MIC-guided TDM increases the amount of time in the therapeutic range compared with trough-guided TDM and is not more nephrotoxic. The findings of this study support the recommendation to transition to the use of AUC/MIC-guided TDM of vancomycin in the veteran population.
Acknowledgments
This material is the result of work supported with the use of facilities and resources from the Sioux Falls Veterans Affairs Health Care System.
1. Gallagher J, MacDougall C. Glycopeptides and short-acting lipoglycopeptides In: Antibiotics Simplified. Jones & Bartlett Learning; 2018.
2. Rybak MJ, Le J, Lodise TP, et al. Therapeutic monitoring of vancomycin for serious methicillin-resistant Staphylococcus aureus infections: a revised consensus guideline and review by the American Society of Health-System Pharmacists, the Infectious Diseases Society of America, the Pediatric Infectious Diseases Society, and the Society of Infectious Diseases Pharmacists. Am J Health Syst Pharm. 2020;77(11):835-864. doi:10.1093/ajhp/zxaa036
3. Hermsen ED, Hanson M, Sankaranarayanan J, Stoner JA, Florescu MC, Rupp ME. Clinical outcomes and nephrotoxicity associated with vancomycin trough concentrations during treatment of deep-seated infections. Expert Opin Drug Saf. 2010;9(1):9-14. doi:10.1517/14740330903413514
4. Poston-Blahnik A, Moenster R. Association between vancomycin area under the curve and nephrotoxicity: a single center, retrospective cohort study in a veteran population. Open Forum Infect Dis. 2021;8(5):ofab094. Published 2021 Mar 12. doi:10.1093/ofid/ofab094
5. Rybak M, Lomaestro B, Rotschafer JC, et al. Therapeutic monitoring of vancomycin in adult patients: a consensus review of the American Society of Health-System Pharmacists, the Infectious Diseases Society of America, and the Society of Infectious Diseases Pharmacists. Am J Health Syst Pharm. 2009;66(1):82-98. doi:10.2146/ajhp080434
6. Moise-Broder PA, Forrest A, Birmingham MC, Schentag JJ. Pharmacodynamics of vancomycin and other antimicrobials in patients with Staphylococcus aureus lower respiratory tract infections. Clin Pharmacokinet. 2004;43(13):925-942. doi:10.2165/00003088-200443130-00005
7. Gyamlani G, Potukuchi PK, Thomas F, et al. Vancomycin-Associated Acute Kidney Injury in a Large Veteran Population. Am J Nephrol. 2019;49(2):133-142. doi:10.1159/000496484
8. Neely MN, Kato L, Youn G, et al. Prospective Trial on the Use of Trough Concentration versus Area under the Curve To Determine Therapeutic Vancomycin Dosing. Antimicrob Agents Chemother. 2018;62(2):e02042-17. Published 2018 Jan 25. doi:10.1128/AAC.02042-17
9. Prabaker KK, Tran TP, Pratummas T, Goetz MB, Graber CJ. Elevated vancomycin trough is not associated with nephrotoxicity among inpatient veterans. J Hosp Med. 2012;7(2):91-97. doi:10.1002/jhm.946
10. Patel N, Stornelli N, Sangiovanni RJ, Huang DB, Lodise TP. Effect of vancomycin-associated acute kidney injury on incidence of 30-day readmissions among hospitalized Veterans Affairs patients with skin and skin structure infections. Antimicrob Agents Chemother. 2020;64(10):e01268-20. Published 2020 Sep 21. doi:10.1128/AAC.01268-20
11. Acute Kidney Injury Work Group. Kidney Disease: Improving Global Outcomes (KDIGO) Clinical Practice Guideline for Acute Kidney Injury. Kidney Int. 2012;2(suppl 1):1-138.
12. Pai MP, Neely M, Rodvold KA, Lodise TP. Innovative approaches to optimizing the delivery of vancomycin in individual patients. Adv Drug Deliv Rev. 2014;77:50-57. doi:10.1016/j.addr.2014.05.016
1. Gallagher J, MacDougall C. Glycopeptides and short-acting lipoglycopeptides In: Antibiotics Simplified. Jones & Bartlett Learning; 2018.
2. Rybak MJ, Le J, Lodise TP, et al. Therapeutic monitoring of vancomycin for serious methicillin-resistant Staphylococcus aureus infections: a revised consensus guideline and review by the American Society of Health-System Pharmacists, the Infectious Diseases Society of America, the Pediatric Infectious Diseases Society, and the Society of Infectious Diseases Pharmacists. Am J Health Syst Pharm. 2020;77(11):835-864. doi:10.1093/ajhp/zxaa036
3. Hermsen ED, Hanson M, Sankaranarayanan J, Stoner JA, Florescu MC, Rupp ME. Clinical outcomes and nephrotoxicity associated with vancomycin trough concentrations during treatment of deep-seated infections. Expert Opin Drug Saf. 2010;9(1):9-14. doi:10.1517/14740330903413514
4. Poston-Blahnik A, Moenster R. Association between vancomycin area under the curve and nephrotoxicity: a single center, retrospective cohort study in a veteran population. Open Forum Infect Dis. 2021;8(5):ofab094. Published 2021 Mar 12. doi:10.1093/ofid/ofab094
5. Rybak M, Lomaestro B, Rotschafer JC, et al. Therapeutic monitoring of vancomycin in adult patients: a consensus review of the American Society of Health-System Pharmacists, the Infectious Diseases Society of America, and the Society of Infectious Diseases Pharmacists. Am J Health Syst Pharm. 2009;66(1):82-98. doi:10.2146/ajhp080434
6. Moise-Broder PA, Forrest A, Birmingham MC, Schentag JJ. Pharmacodynamics of vancomycin and other antimicrobials in patients with Staphylococcus aureus lower respiratory tract infections. Clin Pharmacokinet. 2004;43(13):925-942. doi:10.2165/00003088-200443130-00005
7. Gyamlani G, Potukuchi PK, Thomas F, et al. Vancomycin-Associated Acute Kidney Injury in a Large Veteran Population. Am J Nephrol. 2019;49(2):133-142. doi:10.1159/000496484
8. Neely MN, Kato L, Youn G, et al. Prospective Trial on the Use of Trough Concentration versus Area under the Curve To Determine Therapeutic Vancomycin Dosing. Antimicrob Agents Chemother. 2018;62(2):e02042-17. Published 2018 Jan 25. doi:10.1128/AAC.02042-17
9. Prabaker KK, Tran TP, Pratummas T, Goetz MB, Graber CJ. Elevated vancomycin trough is not associated with nephrotoxicity among inpatient veterans. J Hosp Med. 2012;7(2):91-97. doi:10.1002/jhm.946
10. Patel N, Stornelli N, Sangiovanni RJ, Huang DB, Lodise TP. Effect of vancomycin-associated acute kidney injury on incidence of 30-day readmissions among hospitalized Veterans Affairs patients with skin and skin structure infections. Antimicrob Agents Chemother. 2020;64(10):e01268-20. Published 2020 Sep 21. doi:10.1128/AAC.01268-20
11. Acute Kidney Injury Work Group. Kidney Disease: Improving Global Outcomes (KDIGO) Clinical Practice Guideline for Acute Kidney Injury. Kidney Int. 2012;2(suppl 1):1-138.
12. Pai MP, Neely M, Rodvold KA, Lodise TP. Innovative approaches to optimizing the delivery of vancomycin in individual patients. Adv Drug Deliv Rev. 2014;77:50-57. doi:10.1016/j.addr.2014.05.016